ABSTRACT
Introduction
Eye drops play a prominent role in the treatment and/or management of many ocular diseases, however, they often have limitations. It is recognized that new therapies are needed in this area, to improve patient care.
Areas covered
This manuscript discusses a novel class of materials, known as fluid gels, for their ability to improve ocular care. The paper focuses on how the materials address several key limitations to eye drops, such as longevity, lubrication, and delivery.
Expert opinion
Eye drops are a common source of treatment/management for many ocular diseases. However, lacking the complexity of the native tear, they often fail to hit the mark in terms of performance. In particular, the longevity of many drops and ocular lubricants is insufficient to keep active compounds in situ and/or offer adequate treatment. Fluid gels have high prospects in this area; as careful control over their microstructures provides a platform that can be applied to the ocular surface for in the order of hours. Not only can retention be finely tuned, but such materials also offer highly lubricating properties. Excitingly, promising pre-clinical data, coupled with the means to manufacture at scale, offers a tantalizing new prospect for the future of ocular treatments.
1. Introduction
Globally at least 2.2 billion people have vision impairment, where blindness from corneal opacities including trachoma account for around 6.2 million. Extrapolating, this presents an estimated 1:1274 of the world’s population. Vision impairment poses an enormous global financial burden with annual global losses associated with uncorrected myopia and presbyopia alone estimated to be US$ 244 billion and US$ 25.4 billion [Citation1,Citation2]. Not surprisingly, the WHO set corneal scarring as a priority area, aiming to use high-quality research to reduce preventable causes of sight loss [Citation3]. In 2013 the James Lind Alliance ‘Setting Priorities for Eye Research,’ ranked developing new therapies for corneal disease and effective management of dry eye as the top two priorities for ‘Corneal and External Disease’ [Citation4]. Despite much attention surrounding novel ocular therapies, delivery and treatment to the eye and ocular surface still remains a constant challenge; dominated by contact lens delivery treatments, topical application of eyedrops, and intraocular injections of actives.
1.1. Current treatments in ocular surface disorders
Optimizing therapeutics and vision in patients with corneal disease frequently requires contact lenses, typically classified as Class IIa/III Medical Devices. These provide physical protective barriers in addition to refractive correction: removed and replaced on a daily basis or after extended periods (up to 3 months). Anatomically, several types exist; those physically supported in the precorneal tear-film (corneal lenses), limbus (limbal lenses) or scleral (mini-scleral or scleral lenses) regions of the eye. The scleral devices vault away from the cornea and anterior bulbar conjunctiva creating a reservoir for therapeutic substance capture. Whilst effective in select patients/indications, contact lenses are limited due to patient tolerability, dexterity/handling capability, and high incidence of adverse medical complications – presenting the largest risk factor to corneal infection, the most common ophthalmic medical emergency in the developed world and contributing to vast a financial deficit [Citation5].
More commonly, eye drops present a more preferable option of treatment; as demonstrated by their prominent feature in the patient care strategies outlined in the ‘TFOS DEWS II Management and Therapy Report’ of ocular surface diseases (OSD) [Citation6]. Although an effort to improve eye drops has been made, seeing the use of synthetic gums to reduce evaporative effects, osmoprotectants (L-carnitine and betaine), antimicrobials (Leptospermum, Manuka Honey), and application of colloidal-based systems to deliver actives, the ability to engineer the complex composition and architectural structures of the native tear film remains elusive [Citation7–12]. Notably, failure to sufficiently recapitulate the latter results in a lack of the biological interactions between tear film and tissue, that would typically account for the tears natural longevity [Citation13–15]. To this end, the majority of current drops, predominantly aqueous-based, are either rapidly cleared (within seconds to minutes) through the nasolacrimal duct, or, fail to be retained: due to either loss of hydrophilic transmembrane mucins essential to aide spreading; or, abnormal phospholipid concentration, increasing surface tension and evaporation [Citation16,Citation17]. Frequently, the bioavailability of any therapeutic active within the drop can be limited, significantly constricting efficacy. The retention of drops across the surface of the eye can be improved via the addition of viscosity modifying agents; typically, through the application of long chained polymers (e.g. hydroxypropyl methylcellulose (HPMC), carboxymethylcellulose, hyaluronates), which intrinsically maintain very high polymer to water holding capacities. Ultimately, alteration to the flow profile of the drop reduces drainage under gravity, slowing clearance [Citation18,Citation19]. However, retention often remains transient, again not only hindering drug availability, but for pathology that includes instability of the tear film, duration of symptomatic relief fails to satiate patient satisfaction [Citation20].
As previously alluded to, eye drops play a pivotal role in the management of dry eye, attempting to reinstate the natural equilibrium between the surface hydrophobicity, tension and osmolarity lost with deterioration of the native tear film [Citation21]. Arguably more importantly, commercially available lubricants aim to dampen biomechanical frictional stresses and corneal neural hypersensitivity caused by the abrasive action of blinking eyelids across a damaged ocular surface: which left unchecked often lead to ocular complications such as inflammation, vision impairment and in more serious cases, corneal scarring – not to mention chronic pain. In these cases, the eye drop is modified to exhibit mucin-like compositions or contain moisturizers intended to impart a soothing, lubricating, effect [Citation22–25].
Due to its multifactorial etiology, varying patient-to-patient presentation, and symptom-sign discordance, quantification of treatment effectiveness presents a clinical challenge. In response, patient reported outcome measures (PROMs) have provided a useful tool, typically focusing on aspects which provide greater quality of life [Citation26–28]. Moreover, close collaboration with clinicians and patients alike, highlighted three key areas that need to be addressed to provide an optimal lubricant eye drop:
Longevity – slowly clearing on timescales measured in hours, allowing patients to be ‘free-from drops’.
Inert – comprising of materials which do not exacerbate pathology or impede treatment.
Lubricating – recapitulate reduced biomechanical stresses associated with a healthy tear film.
Nutritional – providing essential substances to the avascular cornea and conjunctival to maintain a healthy ocular surface ecosystem.
2. The role that fluid gels can play to improve eye drop functionality
To this end, fluid gels (FG), a new generation of shear responsive materials, exhibit great promise in significantly advancing the medical field; their highly controlled physicochemical characteristics providing delivery platforms for challenging, articulated, regions of the body [Citation29–34]. Typically formed through a microstructural design process, a common engineering approach to formulation, whereby a fundamental understanding of the interplay between starting material chemistry and manufacturing process is used to engineer material structures on a micro-level, driving desired macro-material behaviors. More specifically, fluid gels are formed through confinement (shearing), applied to the polymer sol undergoing a gel transition ( i). Such processing hinders the formation of a singular, continuous, gelled network, instead restricting growth of micro-gel particles to a length scale determined by the degree of shear ( ii) [Citation32,Citation35,Citation36]. Subsequently, a complex structure consisting of regions of gelled material dispersed within non-gelled (interstitial) polymer, much like a brick and mortar scenario, expands across an aqueous medium [Citation30]. Ultimately, structuring in this way manifests in associations between the particles, the interstitial polymer and particle-polymer ( iii).
Figure 1. Schematic representation of: (i) the sheared process used to fabricate fluid gels. Reproduced from [Citation29], licensed with CC BY 4.0 (https://creativecommons.org/licenses/by/4.0/). (ii) The microstructure of the fluid gel (particles suspended in interstitial polymer. Reproduced from [Citation30], licensed with CC BY 4.0 (https://creativecommons.org/licenses/by/4.0/). (iii) Representation of interactions between the particles and polymer phase. Reproduced from [Citation30], licensed with CC BY 4.0 (https://creativecommons.org/licenses/by/4.0/).
![Figure 1. Schematic representation of: (i) the sheared process used to fabricate fluid gels. Reproduced from [Citation29], licensed with CC BY 4.0 (https://creativecommons.org/licenses/by/4.0/). (ii) The microstructure of the fluid gel (particles suspended in interstitial polymer. Reproduced from [Citation30], licensed with CC BY 4.0 (https://creativecommons.org/licenses/by/4.0/). (iii) Representation of interactions between the particles and polymer phase. Reproduced from [Citation30], licensed with CC BY 4.0 (https://creativecommons.org/licenses/by/4.0/).](/cms/asset/9b295e2b-f691-45a2-86e9-ead07489ab82/ierl_a_2101998_f0001_oc.jpg)
Fundamentally, promotion of interactions as a function of the processing, stimulates a weak floc-like system at zero shear (rest), driving the formation of a solid-like network and corresponding mechanics. However, the application of an external force for example blinking or administration through an aperture, transiently cleaves these weak interactions, serving to facilitate marked shear thinning (liquifying) behavior; as disruption to the floccs allows particles to start ‘squeezing’ over one another [Citation37]. The rapid reformation of an elastic network, as the eye lid reopens (removal of shear) (), results in the formation of a ‘lens,’ slowly being resorbed, layer-by-layer, with continued blinking. Not only does this prevents rapid drainage, residing in the range of hours (four, anecdotally the longest retention time observed in a blinking eye model) (), but typically having high water content (greater than 99%) creates a highly hydrated environment. Indeed, numerous advantages of such systems include reduced surface hydrophobicity, greater control over osmolarity and enhanced delivery/transport of actives to the ocular surface.
Figure 2. (a) Mechanical analysis showing transition from liquid to solid as the eye drop it is squeezed from the applicator. Reproduced from [Citation29], licensed with CC BY 4.0 (https://creativecommons.org/licenses/by/4.0/). (b) Preclinical retention data obtained on a rat model showing retention over several hours. Reproduced from [Citation42], licensed with CC BY 4.0 (https://creativecommons.org/licenses/by/4.0/). (c) Role of lubrication throughout the blinking process: (i) Schematic representation of Stribeck curve highlighting boundary, mixed and hydrodynamic regimes; (ii) Tribology data for fluid gels showing the reduction in friction coefficient (lubricity) as a function of the formulation. Reproduced from [Citation35], licensed with CC BY 3.0 (https://creativecommons.org/licenses/by/3.0/).
![Figure 2. (a) Mechanical analysis showing transition from liquid to solid as the eye drop it is squeezed from the applicator. Reproduced from [Citation29], licensed with CC BY 4.0 (https://creativecommons.org/licenses/by/4.0/). (b) Preclinical retention data obtained on a rat model showing retention over several hours. Reproduced from [Citation42], licensed with CC BY 4.0 (https://creativecommons.org/licenses/by/4.0/). (c) Role of lubrication throughout the blinking process: (i) Schematic representation of Stribeck curve highlighting boundary, mixed and hydrodynamic regimes; (ii) Tribology data for fluid gels showing the reduction in friction coefficient (lubricity) as a function of the formulation. Reproduced from [Citation35], licensed with CC BY 3.0 (https://creativecommons.org/licenses/by/3.0/).](/cms/asset/474fa1d1-2e85-43e5-8a60-662b78ee0d69/ierl_a_2101998_f0002_oc.jpg)
Interestingly, although it may seem counterintuitive, the flocculating particulate nature of the fluid gel drop promotes a lubricating effect. Actually, literature has demonstrated that by altering particle properties at the micro-scale, it is possible to control material coefficient of friction, capable of imparting significant lubricating effects () [Citation35]. Classically, such properties are described as a product of the particulate structures, aiding to minimize the contact area between the surfaces: similar to mechanical bearings [Citation38–40]. A more recent understanding of these complex systems however, suggests that such initial concepts maybe an over simplification: in reality a multi-factorial combination is more likely, consisting of minimized contact area, micro-gel deformability (resulting in expulsion of liquid), coupled with the interstitial polymer evoking slip layers when mechanically stressed [Citation41]. Although an accurately defined mechanism is yet to be established, the phenomenon suggests a means of providing longer-lasting lubricity to the ocular surface.
Pre-clinical, ex vivo and in vivo, evaluation of the FG-drop performance both as delivery and lubricating vehicles has presented highly promising data [Citation29,Citation42]. In one such experiment, exploration into anti-scarring capacity, using a well-established pseudomonas keratitis model, demonstrated that combination the fluid gel with a proteoglycan (decorin) to form an invesitagive medicinal product (IMP) reported superior outcomes in comparison to the current clinical treatment [Citation29]. In all cases test animals were treated with a course of antibiotics and steroids (Gentamicin and Dexamethasone – standard of care) with additional treatment groups comprising of FG-drop with decorin or the FG-drop vehicle as a comparator. Encouragingly, in the presence of the proteoglycan, subjective scoring of corneal opacity was significantly reduced coupled with vastly improved corneal architecture (re-stratification of the epithelium) () and reduction in fibrotic proteins. In addition, such systems have been closely linked to regeneration of adnexal structures, modulating innervation of the cornea following trauma [Citation43]. Perhaps more surprising, in the absence of any active, the added presence of fluid gel enhanced healing over that of the standard of care alone; again, ameliorating corneal opacity, although more interestingly without significantly altering fibrotic markers ().
Figure 3. (a) Macro and histological images of the cornea following various treatments including standard of care (group 1), standard of care with fluid gel (group 2) and standard of care with fluid gel loaded with decorin (group 3) in a model of pseudomonas keratitis. (b) Immunohistochemistry of the cornea having been stained for specific fibrotic markers: (i)αSMA; (ii) Fibronectin; (iii) Laminin. Data show that fluid gel with standard care (group 2) is superior to standard care alone (group 1), while FG with decorin (group 3) restores the cornea to an ‘intact’ baseline phenotype 16 days post-treatment. Reproduced from [Citation29], licensed with CC BY 4.0 (https://creativecommons.org/licenses/by/4.0/).
![Figure 3. (a) Macro and histological images of the cornea following various treatments including standard of care (group 1), standard of care with fluid gel (group 2) and standard of care with fluid gel loaded with decorin (group 3) in a model of pseudomonas keratitis. (b) Immunohistochemistry of the cornea having been stained for specific fibrotic markers: (i)αSMA; (ii) Fibronectin; (iii) Laminin. Data show that fluid gel with standard care (group 2) is superior to standard care alone (group 1), while FG with decorin (group 3) restores the cornea to an ‘intact’ baseline phenotype 16 days post-treatment. Reproduced from [Citation29], licensed with CC BY 4.0 (https://creativecommons.org/licenses/by/4.0/).](/cms/asset/8385e7c8-c949-4241-bae0-a0d2d520e165/ierl_a_2101998_f0003_oc.jpg)
Mechanistically, such observations pose exciting new avenues of research in order to better grasp their regenerative pathways. However, it is possible that superior medicant retention, protection, and lubrication all play a part in enabling a superior outcome. It is postulated that the long-lasting hydrative barriers serve to reestablish key equilibriums, such as osmolarity commonly lost with impaired tear film (), helping to break the vicious cycle that would otherwise over stimulate a prolonged inflammatory response [Citation22,Citation44,Citation45]. Indeed, early works suggest that the FG-drops may even actively modify the inflammatory environment, sequestering pro-inflammatory cytokines (results not yet published). Hence it is reasonable to assume, that such alterations to the wound bed alter progression through the typical healing phases, potentially promoting kinetics with a much lower propensity to scar [Citation46].
Figure 4. Schematic representation of the fluid gel eye drop upon the cornea, highlighting how the material will aid as an osmoprotectant, by providing tear film retention value through structuring ‘trapping’ of the water. Its hydrophilic nature (potentially) overcomes the loss of mucin seen in dry eye disease: thereby FG technology will go a long way to recapitulating the tear film microarchitecture.
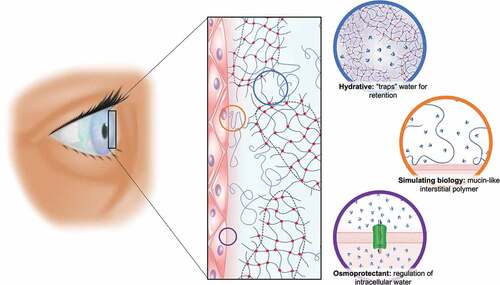
Apart from the array of scientific remits facing not only new ocular, but therapies in general, the movement of these technologies into the clinic are also confronted with many translational challenges (e.g. manufacturing, etc.) [Citation47]. Indeed, expensive toxicological packages and regulatory compliant manufacturing process, which are unarguably integral to maintaining a rigorous outlook to patient wellbeing, are nevertheless, especially challenging for products such as eye drops; where their traditionally, ‘low cost,’ over-the-counter nature is weighed against the large costs of aseptic processing (often required as sensitive constituents cannot withstand conventional sterilization techniques). Again, the future of fluid gels holds much promise, taking advantage of preestablished large-scale manufacturing processes, already employed within industry (for more information on eye drop formulations and fluid gel manufacture, the reader is guided toward [Citation29,Citation32,Citation35,Citation36,Citation47,Citation48]). Moreover, these materials have been scaled appropriately within good manufacturing practices (GMP), along with a comprehensive toxicological package, resulting in a product () that places them on the verge of patient application. Indeed, the next steps in the evaluation of these eye drop technologies are Phase I safety trials. Although primary endpoints are for trials are safety orientated, clinical performance will be collected as important secondary outcomes, and, if successful, should pave the way for more extensive clinical trials.
3. Concluding remarks
In summary, current eye drops are limited, on the most part through an inability to reside on the ocular surface for extended timeframes, ultimately stifling therapeutic bioavailability, minimizing applicability, and failing to provide patient satisfaction/compliance. In response, fluid gels are an interesting class of material, that in the opinion of the authors, have the potential to revolutionize treatments within ophthalmology. These materials have been carefully engineered, taking a materials approach to address three key areas: 1) longevity, clearing on timescales measured in hours; 2) Ability to deliver actives, and; 3) recapitulating the natural lubricating properties associated with a healthy tear film. As such FG-eye drops provide a platform which could be used to deliver of a range of therapeutics to the surface of the eye. Moreover, long retention times not only reduce the need for frequent reapplication but offer the tantalizing prospect of more efficient delivery of existing therapeutics, enhancing bioavailability, and reducing the cost of treatment – particularly important for expensive biopharmaceuticals and emerging ATMPs. With the latter in mind, the horizon for fluid gels could hold for the delivery of ocular cell therapies, having already demonstrated in separate indications the capacity to efficiently deliver populations of fibroblasts to skin through spray devices, allowing migration to the wound site. Indeed, it is hoped that such materials can significantly improve ocular technologies, and ultimately provide enhanced patient benefits.
4. Expert opinion
Eye drops are the most widely used vehicles for the delivery of drug molecules to the eye and are also used as standard to lubricate the ocular surface in the case of dry eye. Although there are some excellent lubricating products, at present most solutions are limited by a relatively short retention time. As such, in some cases patients are prescribed eye drop treatment on an hourly basis, day and night, in order to provide symptomatic relief. In addition, the rapid washout times for most drops loaded with actives results in relatively short drug exposure. Where the drug used is extremely expensive, such as with some biopharmaceuticals, the poor availability means that treatment becomes ineffective or impractical. Efforts have been made to prolong the retention of eye drops on the ocular surface, through the formulation of high-viscosity products or chemical modification: however, these can often be difficult to apply and uncomfortable to use. Furthermore, there is little scope to enhance retention on the surface through compositional modification, due to the requirement for biological compatibility. Indeed, to develop a novel excipient and take it to the market is likely to take many years to collect the appropriate portfolio of safety data.
The work presented here has taken a very different approach to producing a drop, that can be applied easily, retained on the eye, and provide both lubrication and protection to the ocular surface, using materials that have preexisting safety profiles. Rather than modifying composition, it is possible to modify the structure of the polymeric material during manufacture. The result is a material that consists of particles of gel that form a network and thicken at rest, but shear-thin and spread across the surface of the eye during application. It is possible to disperse drugs throughout the structure of this material, then releasing them to the surface of the eye whilst it remains in-situ (>1 hr). This structure also has the tantalizing potential of being able to offer an enhanced lubricating effect for the ocular surface, provided by the ease with which the dispersed particles may pass over one another during shearing. Indeed, this novel particulate structure has been used in the food and fast-moving consumer goods sectors, as an aqueous lubricant. As such it could have significant potential to alleviate the suffering of those with severe dry eye. We believe that the microstructural modification method outlined in this paper provide the opportunity to increase the bioavailability of drugs delivered using eye drops and might provide significant relief for those with severe dry eye – without the need for repeated application. A drop with both enhanced lubrication and ocular retention offers the possibility of easing patient suffering, through lubrication and physical protection while delivering therapeutic agents across the ocular surface. Whilst it is recognized that we must avoid jumping to conclusions guided only by our excellent preclinical findings, we hope that these will be reenforced with imminent first-in-human clinical trials in patients presenting with microbial keratitis (fluid-gel loaded with decorin), and in patients with severe dry eye (serum delivery).
Given the potential for significant modification in structure and properties, we believe that use of the technology for lubrication and ocular surface delivery is just the tip of the iceberg. We believe that it could be used to create a microenvironment to modify the healing process, for cell delivery to the ocular surface, for the delivery of gene therapies or even potent antivirals.
Article highlights
Current eye drop technologies are central to ophthalmology, but often fail to achieve controlled release and sufficient ocular lubrication.
Structuring of polymeric materials can be used to create materials with enhanced functionality.
Fluid gels are an example of one such structured material, which can provide longer retention and superior lubrication.
Preclinical data shows that fluid gels may be used for the delivery of biomacromolecules to mediate scar free healing.
These materials are at the point of first-in-human trials, which if successful, will pave the way for translation into the clinic.
Reviewer disclosures
Peer reviewers on this manuscript have no relevant financial or other relationships to disclose.
Author contributions
All authors conceptualized and worked on the compilation of the manuscript equally.
Disclosure statement
LM Grover and RJ A Moakes are affiliated with Healome Therapeutics Ltd. The authors have no other relevant affiliations or financial involvement with any organization or entity with a financial interest in or financial conflict with the subject matter or materials discussed in the manuscript apart from those disclosed.
Additional information
Funding
References
- Frick KD, Joy SM, Wilson DA, et al. The global burden of potential productivity loss from uncorrected presbyopia. Ophthalmology. 2015;122:1706–1710.
- Naidoo KS, Fricke TR, Frick KD, et al. Potential lost productivity resulting from the global burden of myopia: systematic review, meta-analysis, and modeling. Ophthalmology. 2019;126:338–346.
- World Health Organization. World report on vision; 2019. Available from: https://www.who.int/publications/i/item/9789241516570.
- Loss JL. Sight loss and vision priority setting partnership. Setting priorities for eye research: final report. 2013.
- Moussa G, Hodson J, Gooch N, et al. Calculating the economic burden of presumed microbial keratitis admissions at a tertiary referral centre in the UK. Eye. 2021;35:2146–2154.
- Jones L, Downie LE, Korb D, et al. TFOS DEWS II management and therapy report. Ocul Surf. 2017;15:575–628.
- Corrales RM, Luo L, Chang EY, et al. Effects of osmoprotectants on hyperosmolar stress in cultured human corneal epithelial cells. Cornea. 2008;27:574–579.
- Tan J, Jia T, Liao R, et al. Effect of a formulated eye drop with Leptospermum spp honey on tear film properties. Br J Ophthalmol. 2020;104:1373–1377.
- Albietz JM, Schmid KL. Randomised controlled trial of topical antibacterial Manuka (Leptospermum species) honey for evaporative dry eye due to meibomian gland dysfunction. Clin Exp Optom. 2017;100:603–615.
- Nagarwal RC, Kant S, Singh PN, et al. Polymeric nanoparticulate system: a potential approach for ocular drug delivery. J Control Release. 2009;136:2–13.
- Vandamme TF. Microemulsions as ocular drug delivery systems: recent developments and future challenges. Prog Retin Eye Res. 2002;21:15–34.
- Le Bourlais C, Acar L, Zia H, et al. Ophthalmic drug delivery systems - recent advances. Prog Retin Eye Res. 1998;17:33–58.
- Johnson ME, Murphy PJ. Changes in the tear film and ocular surface from dry eye syndrome. Prog Retin Eye Res. 2004;23:449–474.
- Bron AJ, Tiffany JM, Gouveia SM, et al. Functional aspects of the tear film lipid layer. Exp Eye Res. 2004;78:347–360.
- Mantelli F, Argüeso P. Functions of ocular surface mucins in health and disease. Curr Opin Allergy Clin Immunol. 2008;8:477–483.
- Davies NM. Biopharmaceutical considerations in topical ocular drug delivery. Clin Exp Pharmacol Physiol. 2000;27:558–562.
- Kim M, Lee Y, Mehra D, et al. Dry eye: why artificial tears are not always the answer. BMJ Open Ophthalmol. 2021;6:e000697.
- Benedetto DA, Shah DO, Kaufman HE. The instilled fluid dynamics and surface chemistry of polymers in the preocular tear film. Invest Ophthalmol. 1975;14:887–902.
- Bhamla MS, Giacomin CE, Balemans C, et al. Influence of interfacial rheology on drainage from curved surfaces. Soft Matter. 2014;10:6917–6925.
- Vakros G, Scollo P, Hodson J, et al. Anxiety and depression in inflammatory eye disease: exploring the potential impact of topical treatment frequency as a putative psychometric item. BMJ Open Ophthalmol. 2021;6:649.
- Willcox MDP, Argüeso P, Georgiev GA, et al. TFOS DEWS II tear film report. Ocul Surf. 2017;15:366–403.
- Stern ME, Schaumburg CS, Pflugfelder SC. Dry eye as a mucosal autoimmune disease. Int Rev Immunol. 2013;32:19–41.
- Greaves JL, Wilson CG. Treatment of diseases of the eye with mucoadhesive delivery systems. Adv Drug Deliv Rev. 1993;11:349–383.
- Ludwig A. The use of mucoadhesive polymers in ocular drug delivery. Adv Drug Deliv Rev. 2005;57:1595–1639.
- Bravo-Osuna I, Vicario-De-La-Torre M, Andrés-Guerrero V, et al. Novel water-soluble mucoadhesive carbosilane dendrimers for ocular administration. Mol Pharm. 2016;13:2966–2976.
- Dieckmann G, Ozmen MC, Cox SM, et al. Low-dose naltrexone is effective and well-tolerated for modulating symptoms in patients with neuropathic corneal pain. Ocul Surf. 2021;20:33–38.
- Walt JG, Rowe MM, Stern KL. Evaluating the functional impact of dry eye: the ocular surface disease index. Drug Inf J. 1997;31:1436.
- Lomas RJ, Chandrasekar A, Macdonald-Wallis C, et al. Patient-reported outcome measures for a large cohort of serum eye drops recipients in the UK. Eye. 2021;35:1–8.
- Hill LJ, Moakes RJA, Vareechon C, et al. Sustained release of decorin to the surface of the eye enables scarless corneal regeneration. Npj Regen Med. 2018;3:1–12.
- Foster N, Allen P, El Haj AJ, et al. Tailoring therapeutic responses via engineering micro-environments with a novel synthetic fluid gel. Adv Healthc Mater. 2021;10:2100622.
- Cooke ME, Pearson MJ, Moakes RJA, et al. Geometric confinement is required for recovery and maintenance of chondrocyte phenotype in alginate. APL Bioeng. 2017;1:16104.
- Cooke ME, Jones SW, ter Horst B, et al. Structuring of hydrogels across multiple length scales for biomedical applications. Adv Mater. 2018;30:1705013.
- ter Horst B, Moakes RJA, Chouhan G, et al. A gellan-based fluid gel carrier to enhance topical spray delivery. Acta Biomater. 2019;89:166–179.
- Nikravesh N, Davies OG, Azoidis I, et al. Physical structuring of injectable polymeric systems to controllably deliver nanosized extracellular vesicles. Adv Healthc Mater. 2019;8:1801604.
- Fernández Farrés I, Moakes RJA, Norton IT. Designing biopolymer fluid gels: a microstructural approach. Food Hydrocoll. 2014;42:362–372.
- Norton IT, Jarvis DA, Foster TJ. A molecular model for the formation and properties of fluid gels. Int J Biol Macromol. 1999;26:255–261.
- Gabriele A, Spyropoulos F, Norton IT. Kinetic study of fluid gel formation and viscoelastic response with kappa-carrageenan. Food Hydrocoll. 2009;23:2054–2061.
- Fernández Farrés I, Douaire M, Norton IT. Rheology and tribological properties of Ca-alginate fluid gels produced by diffusion-controlled method. Food Hydrocoll. 2013;32:115–122.
- Garrec DA, Norton IT. Kappa carrageenan fluid gel material properties. Part 2: tribology. Food Hydrocoll. 2013;33:160–167.
- Gabriele A, Spyropoulos F, Norton IT. A conceptual model for fluid gel lubrication. Soft Matter. 2010;6:4205–4213.
- Ghebremedhin M, Seiffert S, Vilgis TA. Physics of agarose fluid gels: rheological properties and microstructure. Curr Res Food Sci. 2021;4:436–448.
- Chouhan G, Moakes RJA, Esmaeili M, et al. A self-healing hydrogel eye drop for the sustained delivery of decorin to prevent corneal scarring. Biomaterials. 2019;210:41–50.
- Wu M, Downie LE, Grover LM, et al. The neuroregenerative effects of topical decorin on the injured mouse cornea. J Neuroinflammation. 2020;17. DOI:10.1186/s12974-020-01812-6.
- Mateo Orobia AJ, Saa J, Lorenzo AO, et al. Combination of hyaluronic acid, carmellose, and osmoprotectants for the treatment of dry eye disease. Clin Ophthalmol. 2018;12:453–461.
- Bron AJ, de Paiva CS, Chauhan SK, et al. TFOS DEWS II pathophysiology report. Ocul Surf. 2017;15:438–510.
- Ferguson MWJ, O’Kane S, Brockes JP. Scar-free healing: from embryonic mechanism to adult therapeutic intervention. Philos Trans R Soc B Biol Sci. 2004;359:839–850.
- Bennett NH, Chinnery HR, Downie LE, et al. Material, immunological, and practical perspectives on eye drop formulation. Adv Funct Mater. 2020;30:1908476.
- Garrec DA, Norton IT. Understanding fluid gel formation and properties. J Food Eng. 2012;112:175–182.