ABSTRACT
Introduction
Keratoconus (KC) is the most prevalent corneal ectasia in the world, and its pathogenesis is influenced by both ocular and systemic factors. This review explores the multifaceted associations between keratoconus and systemic health conditions, ocular characteristics, and various other environmental/exogenous factors, aiming to illuminate how these relationships influence the pathophysiology of the disease.
Areas Covered
This review will summarize the fundamental attributes of KC, review and discuss the systemic and ocular association of KC including molecular biomarkers, and provide an organized overview of the parallel alterations occurring within various biological pathways in KC.
Expert Opinion
Despite the substantial volume of research on keratoconus, the precise etiology of the disease remains elusive. Further studies are necessary to deepen our understanding of this intricate disorder and improve its management.
1. Introduction
Keratoconus (KC) is a progressive, sight-threatening disease and is the most common corneal ectasia in the world [Citation1–3]. It is characterized by thinning and conical protrusion of the cornea [Citation1–4] which often leads to legal blindness or significant visual impairment if left untreated [Citation1]. KC typically onsets during adolescence and progresses into the third or fourth decade of life [Citation1–3] before reaching a stable phase, although the rate of progression and severity varies among demographic and geographic regions [Citation5–7].
The prevalence of KC is variable worldwide, with reported estimates ranging from 1:21 in a young adult population in Saudi Arabia [Citation8,Citation9] to about 1:5700 in an elderly US population [Citation10]. The disease affects both the sexes and all ethnicities [Citation1,Citation11,Citation12], and although male dominance has been reported in the Caucasian KC population [Citation1], the male/female predominance is less clear in higher prevalence regions such as the Middle East. For example, Hashemi et al. reported a higher prevalence in males in Mashhad, Iran [Citation9], but two other studies found a higher prevalence in females in Shahroud, Iran, and Saudi Arabia [Citation8,Citation13]. While it appears that the incidence of KC is increasing worldwide, particularly in developed countries, the higher reported incidences are also due in part to advancements in clinical and diagnostic technology that allow earlier and more accurate diagnoses [Citation1].
KC is diagnosed using many modern techniques. One of the most common methods used is corneal tomography, most commonly the Oculus Pentacam (Pentacam; Oculus Optikgeräte GmbH, Wetzlar, Germany), which maps the anterior and posterior corneas and can allow early detection of the corneal steepening and thinning that occurs in KC [Citation11,Citation14–19]. Another useful tool is the spectral-domain optical coherence tomography (SD-OCT) which can delineate the corneal epithelium and stromal layers [Citation20], unlike topography which only scans the surface of the cornea. Since epithelial thinning is one of the first signs of KC, this method may allow even earlier detection of the disease [Citation21]. Furthermore, changes in corneal biomechanics (reduced corneal hysteresis and resistance factor [Citation22–24]) may be another early and reliable sign of disease and could provide a method for early detection. Castro-Luna et al. have successfully classified subclinical KC using Random Forest, a machine learning model [Citation25], and other techniques being investigated for diagnostics are biomarkers from tears [Citation26], serum [Citation27], saliva [Citation28], and aqueous humor [Citation29], although no distinct biomarkers have been detected for use yet. Genetic testing is also available to assess the risk for KC [Citation11].
KC manifests with unique corneal findings and has been associated with a variety of biomechanical, proteomic, genetic, environmental, metabolic/hormonal, as well as systemic conditions. Precise pathophysiology continues to allude to researchers, with evidence pointing to a multifactorial etiology [Citation1,Citation3,Citation4]. In this review, the systemic and ocular associations of KC will be detailed and considered helping us understand the broader context of the disease.
1.1. Clinical manifestations of KC
KC manifests with varying signs and symptoms based on its severity. Early signs include blurred vision, increased light sensitivity, and increasing astigmatism that standard spherocylindrical spectacle lenses are insufficient to refractively neutralize [Citation30]. In mild-to-moderate cases, localized corneal steepening, usually in the infero-temporal paracentral region, causes an increase in corneal aberrations (e.g. coma) [Citation12,Citation31] that cause the retinoscopy reflex to appear ‘scissored’ [Citation32]. Rizzutti’s sign, a conical reflection on the nasal cornea when light is shone temporally, becomes apparent [Citation33]. Biomicroscopic examination reveals structural changes in KC corneas, such as ferritin deposits and a widening of intracellular epithelial spaces at the base of the cone (Fleisher’s ring) [Citation12,Citation34], visible thinning of the stroma, and stress lines in the posterior stroma (Vogt’s striae) [Citation35–37]. Obvious protrusion of the cornea, displacing the lower eyelid in downgaze (i.e. Munson’s sign) is also observed in severe disease [Citation11,Citation12]. Severe KC may lead to complications such as acute hydrops (occurring in about 1–3% of the cases) [Citation38,Citation39] and stromal scarring; in rare cases, corneal perforation or melt can occur [Citation40,Citation41]. The great visual impact of severe KC, often necessitating a corneal transplant when scarring is significant, highlights the importance of timely and effective management.
1.2. Corneal biomechanics
The corneal tissue has a high tensile strength and elastic modulus, which allows it to maintain its shape and resist deformation under the various surrounding forces [Citation42,Citation43] such as intraocular pressure, blinking, and eye movements [Citation44]. The stroma, normally composed of about 250 collagen lamellae [Citation45], accounts for about 90% of the corneal thickness and likely has the greatest influence on corneal biomechanics [Citation44]. In KC, the cornea appears more susceptible to deformation than normal, which contributes to the conical shape of the cornea (usually inferior) in the disease [Citation46].
Biomechanical changes to the cornea appear early and consistently in KC. The Ocular Response Analyzer (ORA) can measure both corneal hysteresis and corneal resistance factor and is found to be decreased in the disease [Citation22–24]. The newer Corvis ST Tonometer uses a high-speed Scheimpflug camera to capture detailed corneal responses, including parameters such as deformation amplitude, applanation lengths, and corneal velocities. These additional metrics offer deeper insights into the viscoelastic properties of the cornea providing vital information for diagnosing conditions like keratoconus [Citation47–49]. The changes in the corneal deformation properties are potentially a direct result of stromal tissue remodeling [Citation50], splitting of collagen fibrils [Citation51], increased proteolytic activity [Citation52], and/or redistribution of collagen through slippage between lamellae [Citation50,Citation52,Citation53]. Specifically, a reduction in lamellae or the unraveling of lamellae can compromise the cornea’s ability to resist various forces, leading to its increased susceptibility to deformation, as seen in KC. Furthermore, any disturbance in the intricate network of collagen fibrils, through breakdown or redistribution, can impact the cornea’s tensile strength and elasticity [Citation54].
Apoptosis can also lead to keratocyte death in KC and is associated with stromal thinning and degradation [Citation55,Citation56]. In addition, the breakdown of the anterior limiting lamina (i.e. Bowman’s membrane) occurs and could also reduce corneal hysteresis [Citation57]. Since these biomechanical changes are observed early in the disease, they could lead to early detection and more precise monitoring of KC.
1.3. Biomolecular associations in KC
Research over the past 20 years has in part focused on finding a biomarker for KC, assessing cytokines, proteases, cells, metabolites, hormones, and other analytes in KC tears, corneal sections, aqueous humor (AH), and blood. Although obtaining specimens from the cornea and AH is invasive and technically challenging, the tear film and blood provide more easily accessible routes to study biomarkers in the disease. These methods are not without their challenges, such as a small tear volume (as little as 10 μL) that is easily contaminated [Citation26,Citation58], or the somewhat invasive nature of venous blood collection, but much has been learnt about KC from studying these fluids ().
Figure 1. Overview of the various environmental, systemic and ocular associations of keratoconus (KC). Interleukin (IL), matrix metalloproteinase (MMP) Reactive oxygen species (ROS), Lysyl Oxidase (LOX), Collagen Type IV Alpha 3 (COL4A3), Wingless Type 10 A(WNT10A), Visual System Homeobox (VSX), Diphosphoinositol Pentakinase 2 (PPIP5K2), Forkhead Box Protein 01 (FOXO1).
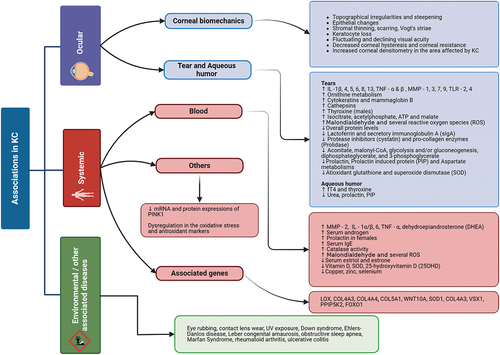
1.3.1. Protein and cellular biomarkers
Several proteomic studies on KC tears have suggested that there is an imbalance of pro- to anti-inflammatory mediators that favor a pro-inflammatory tear phenotype in KC. It is well established that overall protein levels in KC tears are reduced [Citation59–61], most likely due to lower levels of lactoferrin [Citation62] and secretory immunoglobulin A (sIgA) [Citation2,Citation63], two of the largest and most abundant tear proteins. However, despite the reduction in total protein content, there appears to be an increase in inflammatory proteins and a dysregulated balance of pro- and anti-inflammatory proteins that favors a pro-inflammatory environment. For example, there is strong evidence of increases in proteolytic enzymes (i.e. cathepsins [Citation2,Citation64,Citation65], matrix metalloproteinase (MMP)-1 [Citation61,Citation66–68], MMP-9 [Citation2,Citation67,Citation69]) and pro-inflammatory cytokines (i.e. IL-6 and TNF-α), countered by decreases in the anti-inflammatory protease inhibitors (e.g. cystatin) and pro-collagen enzymes (e.g. Prolidase) [Citation60,Citation66,Citation70]. In addition to this strong evidence (i.e. multiple confirming studies), there are other possible alterations in the tear fluid reported, such as an increase in cytokeratins and Mammaglobin B (MGB2) [Citation59,Citation68], as well as increased tear expression of cytokines IL-1β, −4, −5, −8, −13, MMP-3, MMP-7, and TNF-β [Citation66,Citation67], and toll-like receptors (TLR) 2 and 4 (in subclinical KC) [Citation71]. Prolactin-Induced Protein (PIP) is present in many bodily fluids, including tears, where it performs several critical functions related to immune defense and cellular communication. Sharif et al. have demonstrated that PIP levels are notably diminished in the tears and saliva of patients suffering from KC. The reduction in PIP levels in these patients suggests a potential disruption in the protective and regulatory functions of the tear components, which could contribute to the pathophysiology of keratoconus by affecting the corneal surface’s health, its ability to repair and defend against microbial invasion, and possibly influencing the progression of the disease [Citation1,Citation28]. Collectively, over 100 proteins have been assessed in tears in dozens of studies, which collectively support that there are elevated inflammatory cytokines and proteases and a reduction in anti-inflammatory cytokines that favor the classification of a pro-inflammatory tear proteome in KC.
In the blood, alterations have been found in serum proteins in KC, many of which are consistent with the changes seen in the tears, although fewer studies have been reported. Pro-inflammatory factors such as MMP-2, IL-1α/β, IL-6, and TNF-α were found to increase in KC serum in one study [Citation2], and prolidase (enzyme essential for collagen remodeling) has been reported to be reduced [Citation2,Citation72]. Serum immunoglobulins have also been investigated in KC, and an increase in serum IgE levels with and without allergies could provide a molecular association between KC and allergic conditions [Citation2]. In addition, cellular biomarkers such as the monocyte-to-HDL-cholesterol ratio (MHR) and neutrophil-to-lymphocyte ratio (NLR) may be of interest in KC, although one study found both inflammatory biomarkers to be increased [Citation73] and another showed them unchanged [Citation74]. Yet another recent study has found an increase in TLR2 and TLR4 receptors in the monocytes and neutrophils in the KC blood [Citation75], complementing the same increased receptors that have been found in the tears in KC. Although still unclear and in need of additional studies with larger cohorts, these studies do provide evidence that there could be systemic factors that could help understand and diagnose KC.
1.3.2. Metabolic and hormonal biomarkers
The metabolome in KC has been increasingly investigated. A study by Karamichos et al. included 45 subjects in 3 clinically defined groups (healthy, KC patients without corrective lenses, and KC patients wearing Rigid Gas Permeable (RGP) lenses). Analysis by liquid chromatography-mass spectrometry (LCMS) revealed 296 different metabolites and more than 40 of those metabolites showed significant changes in the glycolysis, gluconeogenesis, and urea cycles of KC patients [Citation76,Citation77]. In the urea cycle, there was an upregulation of ornithine and a downregulation of aspartate metabolisms [Citation26]. The study also identified different metabolites belonging to the tricarboxylic acid cycle to be upregulated (isocitrate, acetylphosphate, ATP, and malate) and downregulated (aconitate, malonyl-CoA, glycolysis and/or gluconeogenesis, diphosphateglycerate, and 3-phosphoglycerate) [Citation26]. The upregulation and downregulation of the above metabolites may indicate that the cornea is attempting to maintain homeostasis while experiencing oxidative stress.
Hormones have also been investigated in KC and have shown alterations in the disease. Prolactin and PIP have both been shown to be decreased in the tears and aqueous humor of KC patients [Citation2,Citation28,Citation29], and prolactin has been found to be reduced in KC blood and saliva [Citation2,Citation28]. However, this finding has been challenged by another study that found that prolactin was increased in female KC patients [Citation27], possibly indicating that there are sex differences to be considered. Thyroxine, a hormone involved in metabolism, was found to be elevated in one KC study, and more so in males with the disease [Citation78]. The aqueous humor, located on the opposite side of the cornea, has been found to have increased fT4 and thyroxine concentrations in KC [Citation2,Citation29]. Furthermore, systemic studies have shown a greater incidence of thyroid gland dysfunction in a KC population [Citation79–81], and reductions of serum estriol [Citation2], estrone [Citation2], Vitamin D [Citation2,Citation82,Citation83], and riboflavin levels [Citation84]; dehydroepiandrosterone (DHEA) sulfate [Citation2] and androgen levels [Citation27] were increased in KC serum. These hormonal differences seen in KC compared to normal, while not well defined at this time, could provide insight into how sex and age contribute to KC development and progression.
1.3.3. Evidence of oxidative stress in KC
Several studies have shown altered markers of oxidative stress and an imbalanced redox homeostasis in the blood, cornea, aqueous humor, and tears in KC. A 2021 systematic review and meta-analysis comprehensively summarized the changes in many oxidative and antioxidant stress markers in KC [Citation85]. Notably, there appears to be a consistent increase in several reactive oxygen species (ROS) (e.g. superoxide and nitric oxide) in the tears, cornea, blood, and aqueous humor in KC with an imbalance of antioxidants (e.g. catalase and superoxide dismutase) that are important to reduce ROS-mediated cell damage [Citation65,Citation85–92]. Specifically, decreased blood, tear, and corneal levels of antioxidant glutathione and superoxide dismutase (SOD) have been observed in KC as well as reduced enzymatic activity of lysyl oxidase (LOX) in the tear fluid [Citation2,Citation92–95]. One ROS, malondialdehyde, a reactive aldehyde formed during lipid peroxidation, shows potentially the greatest evidence of consistent increases in the KC cohorts in blood, tear, and cornea samples [Citation86,Citation88,Citation90,Citation92]. In the blood, the total oxidant and antioxidant status have suggested an increase in systemic oxidative stress [Citation92,Citation96–98], and metal ions that are cofactors of enzymes involved in antioxidant activity, crosslinking, and collagen synthesis (i.e. 25-hydroxyvitamin D (25OHD), copper, selenium, iron, and zinc) are lower in KC serum [Citation2,Citation90,Citation99–101], which suggest lowered antioxidative activity in KC patients [Citation99,Citation100]. Furthermore, one group has shown a hyper-response of corneal fibroblasts to oxidative stress in KC cell cultures, including more production of ROS [Citation56,Citation102]. Collectively, it appears that there is an altered oxidative stress response in KC at the local and systemic levels, which could contribute to, or be a sequela of the disease process.
Complementary to the altered ROS response in KC, there may be an irregular response to the degradation of damaged mitochondria. Mitophagy, a conserved, self-degradation process of damaged mitochondria, is reduced at both the mRNA and protein level (gene: PINK1) in the KC corneal epithelium [Citation103]. Shetty et al. reported a difference in the autophagy markers Microtubule-associated protein 1A/1B-light chain 3 (LC3) and ubiquitin-binding protein (p62) in KC corneas when compared to healthy corneas [Citation104,Citation105]. They also saw a clear decrease in the expression of LC3 in the different grades of severity [Citation104,Citation105], ultimately providing evidence that a defective autophagy mechanism could be a consequence of oxidative damage and play a role in KC pathogenesis.
1.4. Genetic associations in KC
Over 20 genes have been linked to KC, and it appears that there may be a genetic cause or predisposition to the disease in certain populations [Citation106,Citation107]. KC shows no Mendelian inheritance patterns, but it can follow an autosomal dominant/recessive inheritance mode in some families [Citation12]. A few case studies indicate strong genetic links in KC although monozygotic twin studies have shown that the twins developed different severities of KC, which suggests a combined environmental and genetic etiology [Citation1,Citation3]. Several studies comparing different ethnicities at the same geographical location also provide evidence of a genetic link. For example, Pearson et al. found that Asians in the UK had a four-times greater prevalence compared to Caucasians living in the same area [Citation1,Citation108] and a positive family history for keratoconus in 5% of Caucasians and 25% of a small Asian subgroup in the Dundee University Scottish Keratoconus study [Citation108,Citation109]. Georgiou et al. also noticed an increase in prevalence in the Asian population compared to Caucasians [Citation110]. Different populations may be predisposed to different genetic expressions based on their environments or inherited genes [Citation111]. In this section, several of the most implicated genes associated with KC are discussed.
1.4.1. Lysyl oxidase (LOX)
The most investigated gene in the KC disease is lysyl oxidase (LOX) [Citation112,Citation113], which has been considered a potential biomarker in keratoconus. This gene plays a role in extracellular matrix maintenance by promoting covalent cross-links of collagens and elastins as well as oxidizing lysine residues which makes them insoluble to the extracellular matrix environment [Citation112,Citation113]. LOX is expressed in different parts of the eye including the cornea [Citation112,Citation113]. Genotyping has shown that LOX affects the Iranian [Citation112], European, and Chinese populations [Citation113]. Mok et al. have observed that Koreans have an exceptionally high odds ratio of LOX in KC patients [Citation113]. Lopes et al. found an absence of mutations in LOX in Brazilian patients that were diagnosed with KC [Citation114].
1.4.2. Collagen Type IV Alpha 3 (COL4A3), Collagen Type IV Alpha 4 (COL4A4), Collagen Type V Alpha 1 (COL5A1)
Research has revealed significant insights into the roles of specific collagen types in KC development. Saravani et al. found that the presence of the COL4A3 gene might reduce the risk of developing KC, suggesting a protective effect [Citation115]. On the other hand, COL4A4, important for structural integrity, has been identified as a potential risk factor among Caucasian populations [Citation116,Citation117]. Furthermore, COL5A1, which contributes to the structure of corneal collagen fibrils, is implicated in the process of central corneal thinning observed in KC [Citation118]. Studies document its role across different ethnic groups, with a specific risk allele linked to a reduced central corneal thickness in both Caucasian and Asian populations [Citation119]. This highlights the gene’s universal relevance to KC pathogenesis, particularly in regard to corneal thinning [Citation120].
1.4.3. Transforming growth factor beta (TGF-β)
The secretion and assembly of the extracellular matrix (ECM), vital for corneal structural integrity, is regulated by TGF-β signaling [Citation121]. In KC, the altered regulation of TGF-β signaling in KC progression has been shown [Citation121], as well as an increase in markers associated with the TGF-β pathway in severe KC patients that suggest greater TGF-β activity may be correlated with disease severity [Citation122].
1.4.4. Wingless type 10 a (WNT10A)
WNT10A, a gene expressed in the central corneal epithelium, plays a significant role in corneal health and integrity [Citation123]. Foster et al. revealed that the protein levels of WNT10A were reduced in both the epithelium and Bowman’s layer in individuals with KC [Citation123], and their study suggested a correlation between WNT10A transcript levels and increased keratometry readings [Citation123]. Adding to the understanding of WNT10A’s role in KC, Cuellar-Partida et al. discovered that a specific variant of WNT10A doubled the risk of developing KC in a population in Western Australia [Citation124].
1.4.5. Superoxide dismutase 1 (SOD1)
SOD1 plays a critical role in converting superoxide radicals into molecular oxygen and hydrogen peroxide, acting as a key antioxidant defense mechanism [Citation125,Citation126]. Its role in KC is still being debated. A study by Moschos et al. suggests a potential causative link between SOD1 and the pathogenesis of keratoconus in Greek patients [Citation127], but studies in the Middle Eastern (mostly from Saudi Arabia and Iran) and Brazilian patients have found no mutation in the SOD1 gene in KC [Citation114,Citation128,Citation129]. These studies indicate that the role of SOD1 in KC may vary among different populations.
1.4.6. Visual system homeobox 1 (VSX1)
In the cornea, VSX1 is thought to play a significant role in maintaining cellular differentiation and transparency. Mok et al. discovered two novel missense mutations in the VSX1 gene among Korean patients with KC, suggesting a possible genetic link to the disease in this population [Citation130]. However, this potential connection does not appear to be universal. Studies by Lopes et al. in Brazil [Citation114] and Al-Muammar et al. in southern Iran [Citation128] did not identify any mutations in the VSX1 gene in their respective KC cohorts, indicating variability in the gene’s role across different ethnic groups. Similarly, Moschos et al. investigated a cohort of Greek patients and found no significant association between polymorphisms in the VSX1 gene and keratoconus, further complicating the genetic associations with KC [Citation127].
1.4.7. Diphosphoinositol pentakinase 2 (PPIP5K2)
PPIP5K2 is a bi-functional kinase/phosphatase pyrophosphate [Citation131] that plays a key role in regulating the synthesis and degradation of inositol pyrophosphates, a group of molecules involved in a wide range of cell-signaling pathways. PPIP5K2’s regulation of inositol pyrophosphates suggests a significant role in controlling cellular activities crucial for maintaining corneal health, transparency, and function [Citation131]. Khaled et al. conducted a four-generational family genetic study that identified potential mutations in the phosphatase domain in PPIP5K2 [Citation7,Citation131]. Such mutations could impact the enzyme’s function, potentially leading to dysregulation of cellular signaling in the cornea.
1.4.8. Forkhead box protein O1 (FOXO1)
FOXO1 is part of the Forkhead box (FOX) transcription family and is an important regulator of cellular oxidative stress [Citation117]. Genetic studies have highlighted the association of FOXO1 with KC. FOXO1 is one of the three genes that showed a genome-wide significant association with KC [Citation117] underscoring its potential involvement in the disease’s pathogenesis. Research examining FOXO1 in diverse populations, including Caucasian and Asian groups, has identified several Single Nucleotide Polymorphisms (SNPs) in patients with KC, pointing to genetic variations that may influence susceptibility to the disease. However, studies in Chinese and Arab populations have not found a significant association, indicating that the relationship between FOXO1 and KC may vary among different ethnic groups.
1.5. Environmental/exogenous associations in KC
KC is a multifactorial disease, and environmental factors may play a role in its progression. Chronic eye rubbing is the greatest independent behavioral risk factor in the development of KC [Citation132]. A study conducted by Hassan et al. found that eye rubbing was associated with a 3.09 odds ratio, indicating a significant link between this behavior and the development or progression of KC [Citation133]. The impact of eye rubbing on KC may vary depending on the duration and intensity of the rubbing, as some studies have shown that gentle rubbing may not have the same effect as a more vigorous rubbing [Citation109,Citation134]. A case was reported of a 4-year-old girl who developed bilateral KC secondary to chronic eye rubbing [Citation135], and other research suggests that individuals with KC tend to rub their eyes for a longer duration (between 10 and 180 seconds) compared to those with allergic or infective ocular disorders, whose eye rubbing usually lasts for less than 15 seconds [Citation108,Citation136]. Similarly, there have been multiple case studies of asymmetric KC development and worsening of the corneal curvature attributed to this behavior [Citation119,Citation137–139].
Contact lens wear is another environmental factor that has been linked to the possible progression of KC [Citation140]. It is postulated that contact lens wear causes micro-trauma, potentially leading to keratocyte apoptosis and potentially triggering remodeling of the stromal matrix [Citation141], which may increase the likelihood of developing or worsening KC [Citation142]. It has been demonstrated that both soft hydrogel and RGP contact lenses induce the release of MMP-9 and other inflammatory molecules in the tear film of normal subjects [Citation141], and Lema et al. noted that RGP lenses caused greater increases in IL-6, TNF-alpha, ICAM-1, and VCAM-1 in the tears of patients with KC compared to normal myopic lens wearers [Citation67]. In a study conducted by Bitirgen et al., it was revealed that KC patients who wore contact lenses experienced a significant reduction in basal epithelial cell density compared to those who did not wear them [Citation143]. Additionally, contact lens wear is known to exacerbate dry eye symptoms, which could lead to increased eye rubbing among KC patients [Citation144].
External environmental factors such as UV exposure and pollution have been found to have associations with KC [Citation108,Citation145]. However, further research is needed to establish a conclusive link particularly in the case of UV light [Citation108]. While the precise mechanism by which pollution influences KC remains uncertain, there is speculation that particulate matter in highly polluted areas might aggravate ocular allergy symptoms and the propensity for eye rubbing, thereby increasing the prevalence of KC [Citation145]. Preliminary studies suggest a potential correlation between exposure to certain airborne pollutants and increased KC prevalence. Jurkiewicz and Marty showed that fine particulate matter may be a possible risk factor for KC although more extensive research in diverse populations is necessary [Citation145]. Interestingly, two independent studies have found a negative correlation or no association between cigarette smoking and KC [Citation146,Citation147]. The authors proposed that the by-products of cigarette smoke could potentially induce a beneficial cross-linking effect in the cornea [Citation147].
1.6. Systemic conditions associated with KC
In understanding the ocular and systemic biomarkers that have been linked to KC, it may also be useful to consider them in the context of the several associated comorbidities such as Down syndrome [Citation148], Ehlers–Danlos disease [Citation149], Leber congenital amaurosis [Citation150], obstructive sleep apnea [Citation151–153], as well as several inflammatory-based diseases. KC can also be influenced by transient systemic conditions such as pregnancy, which can contribute to the progression of KC [Citation154–158]. Many of these disease associations are thought to be genetically and/or behaviorally linked to KC, but the associations are challenging to delineate.
Down syndrome (DS) is the most common chromosomal anomaly [Citation159] that has been reported to frequently present with ophthalmic manifestations [Citation160]. Current literature suggests that 75% of the patients with DS tend to have corneal morphological features indicative of KC [Citation161]. However, the prevalence of KC in DS patients has been reported to vary greatly between 0% and 71% [Citation148]. It has been suggested that this association occurs as a result of central corneal thickness, genetics, and vigorous eye rubbing clinically described in DS patients [Citation7]. Central corneal thickness (CCT) is decreased in patients with KC [Citation162], and Evereklioglu et al. reported that the mean CCT in DS was significantly less (488.39 ± 39.87 µm) than that in the healthy control subjects (536.25 ± 20.70 µm) [Citation163]. Several chromosomal regions have been linked with CCT variations and the potential risk of KC development [Citation164]. COL6A1 and COL6A2, which encode for type VI collagen [Citation165], are on chromosome 21 and may play a role in the pathological connection between KC and DS [Citation166].
KC has been associated with other congenital connective tissue disorders and collagen abnormalities, such as Ehlers–Danlos syndrome (EDS) [Citation167]. There are several subtypes of this condition, many of which are associated with mutations in genes that produce fibrillar collagens or enzymes that modify these proteins [Citation168]. A study done by Fransen et al. was the first to demonstrate a consistent role of genetic variants in Ehlers−Danlos genes in the etiology of KC [Citation149]. The study identified significant associations between KC and variations in the COL5A1 and ZNF469 genes, as well as in two other genes related to EDS – COL12A1 and TNXB – and also in the COL2A1 gene, which codes for a component of type II collagen [Citation149].
Leber congenital amaurosis (LCA) has been reported to be associated with KC. LCA is a family of congenital retinal dystrophy that leads to visual imparity [Citation169]. A common manifestation of LCA is Franceschetti’s oculo-digital sign, which is a result of repeated poking, pressing, and rubbing of the eyes [Citation170] observed in up to 30% of the patients with KC [Citation171]. Varying results have been reported on the association of LCA patients with AIPL1 mutations and KC. Dharmaraj et al. reported that 26% of the 19 LCA patients presented with the mutation and KC and cataracts [Citation172]. Whereas McMahon et al. found no association between AIPL1 and KC [Citation150]. The study did suggest that patients with LCA with a CRB1 mutation may have susceptibility to develop KC [Citation150].
Obstructive Sleep Apnea (OSA) is a common sleep disorder marked by repeated interruptions in breathing due to upper airway collapse during sleep [Citation173]. The relationship between OSA and KC has been in question for decades. In a late 1990s investigation, Mojon et al. found that 2.3% of the patients with OSA were also diagnosed with KC [Citation174]. Since then, there have been many inquiries into the relationship between OSA and KC. In two separate studies, Gupta et al. and Saidel et al. found that the prevalence of OSA in patients with KC was 18% [Citation151] and 19.6% [Citation175], respectively. A 2020 meta-analysis concluded that there is an association with OSD and KC [Citation152], but there is no clear pathophysiologic connection. The underlying connection between KC and OSA is likely complex, and both are also associated with highly prevalent developed world conditions such as obesity [Citation151,Citation175]. In a prospective case–control study of 50 patients with KC, Pihlblad and Schaefer found almost 25% had OSA (12/50) and that over 50% (26/50) were obese [Citation153]. There could also be a common factor in sleep position; it has been suggested that people who sleep with their hands near their face could be at greater risk of both KC and sleep apnea [Citation151,Citation176]. Proposed theories including chronic intermittent hypoxia (a hallmark of OSA) may influence corneal collagen structure and metabolism, potentially contributing to KC. Additionally, OSA can cause oxidative stress, which is believed to play a role in KC development. Also, individuals with OSA may often rub their eyes at night, a known risk factor for KC [Citation134,Citation177]. It has also been suggested that a common (dysregulated) MMP pathway could be the connection between KC and OSA [Citation153]. Researchers continue to investigate the link between these two diseases.
Although controversial, one factor that may protect from this disease is diabetes. The hypothesis is that chronic high blood glucose levels lead to the glycosylation of the corneal stromal fibrils, providing natural collagen crosslinking (CXL), strengthening the corneal tissue, and thus reducing the risk of developing KC [Citation1,Citation178,Citation179]. Kuo et al. performed two different studies investigating diabetes in KC. The first study focused on evaluating diabetes mellitus (DM) prevalence in patients with and without KC. This study found that there was no difference in the prevalence of DM in patients with or without KC. Study 2 focused on evaluating KC severity in patients with and without DM. This study found that the severity of KC was negatively associated with the presence of DM [Citation180]. The relationship between Type 2 Diabetes Mellitus (T2DM) and KC is the subject of extensive discussion and debate. Some studies have stated that T2DM provides a protective effect against KC [Citation180–183], while other studies have stated that T2DM has a positive association with KC [Citation184,Citation185].
The association of KC with several inflammatory diseases has been documented but is not well understood. A retrospective study in Israel found increased odds for the following diseases in KC: rheumatoid arthritis (OR: 8.1), ulcerative colitis (OR: 12.1), autoimmune chronic active hepatitis (OR: 6), and irritable bowel syndrome (OR: 5) [Citation186]; other associated inflammatory diseases include Hashimoto’s thyroiditis, asthma, vernal keratoconjunctivitis, environmental allergies, and atopy [Citation187–190]. However, there is controversy [Citation109,Citation191] and some indication that the behavior of eye rubbing may drive the associations, particularly with allergic conditions [Citation186]. In less obvious correlations, such as those with rheumatoid arthritis and ulcerative colitis, an underlying autoimmune link is suspected, though its specific nature remains to be clarified. These associated diseases might also lead to heightened inflammation on the ocular surface, potentially predisposing a patient to the development of KC [Citation186]. It has been suggested that atopy may be a common factor underlying both KC and certain inflammatory conditions, such as irritable bowel syndrome [207]. However, research in some populations has not established a clear link between atopy and the development of KC [Citation186]. Moreover, conditions like leukocytoclastic vasculitis (LVC) characterized histopathologically by immune complex-mediated vasculitis of the dermal capillaries and venules have been occasionally associated with KC, indicating a potential interplay of vascular inflammation in its pathogenesis [Citation192]. However, a direct causative relationship between KC and LCV has not been established [Citation193]. While direct causality between systemic inflammatory diseases and KC remains an area of ongoing investigation, reported associations between KC and autoimmune systemic diseases lend support to the current understanding of multifactorial etiology in KC that exists with several genetic and environmental contributing factors.
2. Considering the multi-factorial influences on KC
The pathophysiology of KC is certainly complex, but there can be important insight provided when the various associations of the disease are discussed and considered together. Certainly, it is widely agreed that genetics plays a role and can pre-dispose an individual to developing KC, but it seems for most populations that there are other factors, environmental or otherwise external to the genome, that can drive KC development. Based on the wide multifactorial associations with KC, we propose that patients who develop KC likely have a genetic predisposition toward faulty mechanisms related to ocular surface stress and collagen remodeling, which in the presence of certain hormonal, environmental, and inflammatory settings provide the perfect storm to develop KC. The cornea is the most anterior structure of the anterior chamber and is particularly vulnerable to external influences such as pollution or UV light, and also to behavioral influences like eye rubbing. These factors can trigger ocular surface inflammation including oxidative stress, and in KC, there appears to be a faulty mechanism for responding to stress. Indeed, many of the altered genes in KC (i.e. SOD1, LOX, and gen) are involved in managing oxidative stress on the ocular surface. Oxidative stress can also lead to apoptosis of keratocytes and reduced collagen in KC. The role of hormones and metabolites is intertwined in all physiological processes for example, in oxidative stress responses (estradiol, vitamin D), and while it is not well understood how they contribute to KC, important changes have been discovered. Another imperative variable to consider is that the onset of the disease in most patients occurs at the end of puberty, which could be related to changing hormones that could trigger a susceptible patient to develop KC.
3. Conclusion
KC is a multifaceted disease with a complex interplay of biomechanical, genetic, and environmental factors contributing to its onset and progression. The relationships between KC, oxidative stress, and other systemic diseases are intricate and warrant further research to elucidate the underlying mechanisms and potential therapeutic targets.
4. Expert Opinion
KC is widely accepted as a complex, multifactorial, and challenging disease of the human cornea, especially as it relates to its early detection and treatment. The types of symptoms, and how severe they are, can differ widely from person to person and from geographical region to region. Many different factors can affect the course the disease runs, including the age of diagnosis. KC is also known to affect both males and females, and its sex-specific impact is just emerging as a key piece to the disease's manifestations and could provide details on systemic mechanisms. KC can have numerous comorbidities; however, the reason for those is largely unexplored. Future studies could eventually lead us to targeted therapies that can better treat and relieve the population of this debilitating disease. As it relates to early diagnosis/detection, technological advancements and the development of more sensitive clinical equipment could significantly help these efforts. Patient compliance can also be refined by improving the clinical examinations and specimen collection techniques to be more comfortable for patients. Available treatments need to be developed further. The primary treatments available are collagen crosslinking (CXL) and corneal transplantation, the latter of which introduces an entirely new condition to manage in its post-surgical state. CXL is the most effective and aims to arrest progressive KC, although continued progression has been reported in some cases and there are minor risks associated with the surgical procedure. It is therefore clear that clinical and lab-based research holds a great many promises in advancing our current knowledge and developing future tools and therapies, with the ultimate goal of preventing this disease from occurring in people all across the globe. By understanding KC at a molecular level, we can expect to have a better understanding of the disease and how it progresses before physical manifestations occur. Such information can then be used by ophthalmologists who will have the tools necessary to diagnose the disease earlier and provide effective treatments to slow the progression of KC. A promising research area to explore is the world of extracellular vesicles (EVs). These vesicles may hold clues to the progression of the disease, given their ability to travel far within the body and reach target organs and cells with great precision. Their surface proteins and our ability to detect and analyze them could provide clues about KC pathobiology. It is very possible that this field of research will not reach a definitive end-point due to the imaginative curiosity that scientists hold. Scientists will still be researching KC for many years to come, but hopefully, we will make some advancements in discoveries. Maybe the gene list will be narrowed down to a few candidate genes, and genetics will be once again at the forefront. Artificial Intelligence (AI) may help guide the field with pattern recognition software that can spot patterns that humans do not see when investigating KC. Perhaps, novel biomarkers unique to KC will be able to distinguish KC manifestations among different ethnic and racial groups. As more discoveries are made, we will better understand this disease and develop more effective tools to slow the progression of the disease and maybe one day, stop the progression all together. It has been the ‘dream’ in the KC field, as well as others, that a simple blood test or a drop of tears may hold the clues necessary for early diagnosis. We still have a long road ahead, but if we continue being passionate about this disease, anything is possible.
Article highlights
Keratoconus (KC) is a sight-threatening disease that is multifactorial and shapes the cornea into a cone.
Current KC research is investigating protein and cellular biomarkers as well as metabolic and hormonal biomarkers.
KC may also be impacted by genetic and environmental influences.
Other diseases may play a role in the development of KC such as sleep apnea and down syndrome.
Declaration of interest
The authors have no relevant affiliations or financial involvement with any organization or entity with a financial interest in or financial conflict with the subject matter or materials discussed in the manuscript. This includes employment, consultancies, honoraria, stock ownership or options, expert testimony, grants, or patents received or pending, or royalties.
Reviewer disclosures
Peer reviewers in this manuscript have no relevant financial or other relationships to disclose.
Additional information
Funding
References
- Sharif R, Bak-Nielsen S, Hjortdal J, et al. Pathogenesis of Keratoconus: the intriguing therapeutic potential of Prolactin-inducible protein. Prog Retin Eye Res. 2018 Nov;67:150–167. doi: 10.1016/j.preteyeres.2018.05.002
- Shetty R, D’Souza S, Khamar P, et al. Biochemical markers and alterations in keratoconus. Asia Pac J Ophthalmol (Phila). 2020 Dec;9(6):533–540. doi: 10.1097/APO.0000000000000332
- Ionescu C, Corbu CG, Tanase C, et al. Inflammatory biomarkers profile as microenvironmental expression in keratoconus. Dis Markers. 2016;2016:1243819. doi: 10.1155/2016/1243819
- Stunf Pukl S. Are miRnas dynamic biomarkers in keratoconus? a review of the literature. Genes (Basel). 2022 Mar 25;13(4):588. doi: 10.3390/genes13040588
- Gokhale NS. Epidemiology of keratoconus. Indian J Ophthalmol. 2013 Aug;61(8):382–383. doi: 10.4103/0301-4738.116054
- Crawford AZ, Zhang J, Gokul A, et al. The enigma of environmental factors in keratoconus. Asia Pac J Ophthalmol (Phila). 2020 Dec;9(6):549–556. doi: 10.1097/APO.0000000000000334
- Akoto T, Li JJ, Estes AJ, et al. The underlying relationship between keratoconus and down syndrome. Int J Mol Sci. 2022 Sep 16;23(18):10796. doi: 10.3390/ijms231810796
- Torres Netto EA, Al-Otaibi WM, Hafezi NL, et al. Prevalence of keratoconus in paediatric patients in Riyadh, Saudi Arabia. Br J Ophthalmol. 2018 Oct;102(10):1436–1441. doi: 10.1136/bjophthalmol-2017-311391
- Hashemi H, Khabazkhoob M, Yazdani N, et al. The prevalence of keratoconus in a young population in Mashhad, Iran. Ophthalmic Physiol Opt. 2014 Sep;34(5):519–527. doi: 10.1111/opo.12147
- Reeves SW, Ellwein LB, Kim T, et al. Keratoconus in the medicare population. Cornea. 2009 Jan;28(1):40–42. doi: 10.1097/ICO.0b013e3181839b06
- Mas Tur V, MacGregor C, Jayaswal R, et al. A review of keratoconus: diagnosis, pathophysiology, and genetics. Sur Ophthalmol. 2017 Nov;62(6):770–783. doi: 10.1016/j.survophthal.2017.06.009
- Santodomingo-Rubido J, Carracedo G, Suzaki A, et al. Keratoconus: an updated review. Cont Lens Anterior Eye. 2022 Jan 3;45(3):101559. doi: 10.1016/j.clae.2021.101559
- Hashemi H, Beiranvand A, Khabazkhoob M, et al. Prevalence of keratoconus in a population-based study in Shahroud. Cornea. 2013 Nov;32(11):1441–1445. doi: 10.1097/ICO.0b013e3182a0d014
- Motlagh MN, Moshirfar M, Murri MS, et al. Pentacam(R) corneal tomography for screening of refractive surgery candidates: a review of the literature, part I. Med Hypothesis Discov Innov Ophthalmol. 2019 Fall;8(3):177–203.
- Masiwa LE, Moodley V. A review of corneal imaging methods for the early diagnosis of pre-clinical Keratoconus. J Optom. 2020 Oct;13(4):269–275. doi: 10.1016/j.optom.2019.11.001
- Rogers GJ, Attenborough M. Bilateral superior keratoconus: two case reports. Eye (Lond). 2014 Oct;28(10):1254–1257. doi: 10.1038/eye.2014.146
- Asimellis G, Kaufman EJ. Keratoconus. Treasure Island (FL): StatPearls; 2023.
- Krachmer JH, Feder RS, Belin MW. Keratoconus and related noninflammatory corneal thinning disorders. Surv Ophthalmol. 1984 Jan;28(4):293–322. doi: 10.1016/0039-6257(84)90094-8
- Morishige N, Magome K, Ueno A, et al. Relations among corneal curvature, thickness, and volume in keratoconus as evaluated by anterior segment-optical coherence tomography. Invest Ophthalmol Vis Sci. 2019 Sep 3;60(12):3794–3802. doi: 10.1167/iovs.19-27619
- Lu NJ, Hafezi F, Koppen C, et al. New keratoconus staging system based on OCT. J Cataract Refract Surg. 2023 Nov 1;49(11):1098–1105. doi: 10.1097/j.jcrs.0000000000001276
- Yang XL, Wang Y, Luo BG, et al. Corneal epithelial thickness analysis of forme fruste keratoconus with optical coherence tomography. Int J Ophthalmol. 2021;14(1):89–96. doi: 10.18240/ijo.2021.01.13
- Fontes BM, Ambrosio R Jr., Velarde GC, et al. Ocular response analyzer measurements in keratoconus with normal central corneal thickness compared with matched normal control eyes. J Refract Surg. 2011 Mar;27(3):209–215. doi: 10.3928/1081597X-20100415-02
- Mohammadpour M, Etesami I, Yavari Z, et al. Ocular response analyzer parameters in healthy, keratoconus suspect and manifest keratoconus eyes. Oman J Ophthalmol. 2015 May;8(2):102–106. doi: 10.4103/0974-620X.159255
- Sedaghat MR, Ostadi-Moghadam H, Jabbarvand M, et al. Corneal hysteresis and corneal resistance factor in pellucid marginal degeneration. J Curr Ophthalmol. 2018 Mar;30(1):42–47. doi: 10.1016/j.joco.2017.08.002
- Castro-Luna G, Jimenez-Rodriguez D, Castano-Fernandez AB, et al. Diagnosis of subclinical keratoconus based on machine learning techniques. J Clin Med. 2021 Sep 21;10(18):4281. doi: 10.3390/jcm10184281
- Khanna RK, Catanese S, Emond P, et al. Metabolomics and lipidomics approaches in human tears: A systematic review. Surv Ophthalmol. 2022 Jul;67(4):1229–1243. doi: 10.1016/j.survophthal.2022.01.010
- Jamali H, Heydari M, Masihpour N, et al. Serum androgens and prolactin levels in patients with keratoconus. Clin Exp Optom. 2022 Jun;106(5):484–488. doi: 10.1080/08164622.2022.2081067
- Sharif R, Bak-Nielsen S, Sejersen H, et al. Prolactin-Induced Protein is a novel biomarker for Keratoconus. Exp Eye Res. 2019 Feb;179:55–63. doi: 10.1016/j.exer.2018.10.015
- Stachon T, Stachon A, Hartmann U, et al. Urea, Uric Acid, Prolactin and fT4 Concentrations in Aqueous Humor of Keratoconus Patients. Curr Eye Res. 2017 Jun;42(6):842–846. doi: 10.1080/02713683.2016.1256413
- Asimellis G, Kaufman EJ. Keratoconus. Treasure Island (FL): StatPearls; 2022.
- Romero-Jimenez M, Santodomingo-Rubido J, Gonzalez-Meijome JM. The thinnest, steepest, and maximum elevation corneal locations in noncontact and contact lens wearers in keratoconus. Cornea. 2013 Mar;32(3):332–337. doi: 10.1097/ICO.0b013e318259c98a
- Lawless M, Coster DJ, Phillips AJ, et al. Keratoconus: diagnosis and management. Aust N Z J Ophthalmol. 1989 Feb;17(1):33–60. doi: 10.1111/j.1442-9071.1989.tb00487.x
- Espandar L, Meyer J. Keratoconus: overview and update on treatment. Middle East Afr J Ophthalmol. 2010 Jan;17(1):15–20. doi: 10.4103/0974-9233.61212
- Loh A, Hadziahmetovic M, Dunaief JL. Iron homeostasis and eye disease. Biochim Biophys Acta. 2009 Jul;1790(7):637–649. doi: 10.1016/j.bbagen.2008.11.001
- Grieve K, Ghoubay D, Georgeon C, et al. Stromal striae: a new insight into corneal physiology and mechanics. Sci Rep. 2017 Oct 19;7(1):13584. doi: 10.1038/s41598-017-13194-6
- Sharma VK, Sinha R, Bandopadhyay S. Vogt striae: Demystified. Med J Armed Forces India. 2022. doi: 10.1016/j.mjafi.2022.10.008
- Gungor IU, Beden U, Sonmez B. Bilateral horizontal Vogt’s striae in keratoconus. Clin Ophthalmol. 2008 Sep;2(3):653–655. doi: 10.2147/OPTH.S2573
- Bachmann BO, Matthaei M, Schlereth S, et al. Corneal hydrops - aetiology and advanced therapeutic strategies. Klin Monbl Augenheilkd. 2023 Jun;240(6):795–802. doi: 10.1055/a-2048-6703
- Tuft SJ, Gregory WM, Buckley RJ. Acute corneal hydrops in keratoconus. Ophthalmol. 1994 Oct;101(10):1738–1744. doi: 10.1016/S0161-6420(94)31110-9
- Jovanovic V, Nikolic L, Seiler TG, et al. Acute hydrops followed by corneal perforation five years after corneal cross-linking for keratoconus. Arq Bras Oftalmol. 2022May 9;86(5). doi: 10.5935/0004-2749.20230059
- Tsaousis KT, Chalvatzis N, Symeonidis C, et al. Corneal perforation as a primary manifestation of keratoconus in a patient with underlying rheumatoid arthritis. J Ophthalmic Inflamm Infect. 2012 Mar;2(1):57–60. doi: 10.1007/s12348-011-0048-4
- Asher R. Etiology of Keratoconus: proposed biomechanical pathogenesis. Null Cell Null Cci. 2014;1(1). doi: 10.1186/2196-050X-1-3
- Asher R, Gefen A, Varssano D. Patient-Specific Modeling of the Cornea. In: Amit G, editor. Patient-Specific Modeling in Tomorrow’s Medicine. 1st ed. Vol. 9. Studies in Mechanobiology, Tissue Engineering and Biomaterials. Germany: Springer, Berlin, Heidelberg; 2011. p. 461–483. doi: 10.1007/8415_2011_106
- Gefen A, Shalom R, Elad D, et al. Biomechanical analysis of the keratoconic cornea. J Mech Behav Biomed Mater. 2009 Jul;2(3):224–236. doi: 10.1016/j.jmbbm.2008.07.002
- Meek KM. Corneal collagen-its role in maintaining corneal shape and transparency. Biophys Rev. 2009 Jul;1(2):83–93. doi: 10.1007/s12551-009-0011-x
- Alhayek A, Lu PR. Corneal collagen crosslinking in keratoconus and other eye disease. Int J Ophthalmol. 2015;8(2):407–418. doi: 10.3980/j.issn.2222-3959.2015.02.35
- Elham R, Jafarzadehpur E, Hashemi H, et al. Keratoconus diagnosis using Corvis ST measured biomechanical parameters. J Curr Ophthalmol. 2017 Sep;29(3):175–181. doi: 10.1016/j.joco.2017.05.002
- Niazi S, Jimenez-Garcia M, Findl O, et al. Keratoconus diagnosis: from fundamentals to artificial intelligence: a systematic narrative review. Diagnostics (Basel). 2023Aug 21;13(16):2715. doi: 10.3390/diagnostics13162715
- Vinciguerra R, Ambrosio R Jr., Elsheikh A, et al. Detection of keratoconus with a new biomechanical index. J Refract Surg. 2016Dec 1;32(12):803–810. doi: 10.3928/1081597X-20160629-01
- Fullwood NJ, Tuft SJ, Malik NS, et al. Synchrotron x-ray diffraction studies of keratoconus corneal stroma. Invest Ophthalmol Vis Sci. 1992 Apr;33(5):1734–1741.
- Mathew JH, Goosey JD, Soderberg PG, et al. Lamellar changes in the keratoconic cornea. Acta Ophthalmol. 2015 Dec;93(8):767–773. doi: 10.1111/aos.12811
- Zhou L, Sawaguchi S, Twining SS, et al. Expression of degradative enzymes and protease inhibitors in corneas with keratoconus. Invest Ophthalmol Vis Sci. 1998 Jun;39(7):1117–1124.
- Davidson AE, Hayes S, Hardcastle AJ, et al. The pathogenesis of keratoconus. Eye (Lond). 2014 Feb;28(2):189–195. doi: 10.1038/eye.2013.278
- Meek KM, Tuft SJ, Huang Y, et al. Changes in collagen orientation and distribution in keratoconus corneas. Invest Ophthalmol Vis Sci. 2005 Jun;46(6):1948–1956. doi: 10.1167/iovs.04-1253
- Soiberman U, Foster JW, Jun AS, et al. Pathophysiology of keratoconus: what do we know today. Open Ophthal J. 2017;11(Suppl–1, M9):252–261. doi: 10.2174/1874364101711010252
- Chwa M, Atilano SR, Reddy V, et al. Increased stress-induced generation of reactive oxygen species and apoptosis in human keratoconus fibroblasts. Invest Ophthalmol Vis Sci. 2006 May;47(5):1902–1910. doi: 10.1167/iovs.05-0828
- Naderan M, Jahanrad A, Balali S. Histopathologic findings of keratoconus corneas underwent penetrating keratoplasty according to topographic measurements and keratoconus severity. Int J Ophthalmol. 2017;10(11):1640–1646. doi: 10.18240/ijo.2017.11.02
- Chang AY, Purt B. Biochemistry, Tear Film. Treasure Island (FL): StatPearls; 2022.
- Ghosh A, Zhou L, Ghosh A, et al. Proteomic and gene expression patterns of keratoconus. Indian J Ophthalmol. 2013 Aug;61(8):389–391. doi: 10.4103/0301-4738.116056
- Acera A, Vecino E, Rodriguez-Agirretxe I, et al. Changes in tear protein profile in keratoconus disease. Eye (Lond). 2011 Sep;25(9):1225–1233. doi: 10.1038/eye.2011.105
- Balasubramanian SA, Mohan S, Pye DC, et al. Proteases, proteolysis and inflammatory molecules in the tears of people with keratoconus. Acta Ophthalmol. 2012 Jun;90(4):e303–9. doi: 10.1111/j.1755-3768.2011.02369.x
- Pastori V, Tavazzi S, Lecchi M. Lactoferrin-loaded contact lenses counteract cytotoxicity caused in vitro by keratoconic tears. Cont Lens Anterior Eye. 2019 Jun;42(3):253–257. doi: 10.1016/j.clae.2018.12.004
- Balasubramanian SA, Pye DC, Willcox MD. Levels of lactoferrin, secretory IgA and serum albumin in the tear film of people with keratoconus. Exp Eye Res. 2012 Mar;96(1):132–137. doi: 10.1016/j.exer.2011.12.010
- Pahuja N, Kumar NR, Shroff R, et al. Differential molecular expression of extracellular matrix and inflammatory genes at the corneal cone apex drives focal weakening in keratoconus. Invest Ophthalmol Vis Sci. 2016 Oct 1;57(13):5372–5382. doi: 10.1167/iovs.16-19677
- Saijyothi AV, Fowjana J, Madhumathi S, et al. Tear fluid small molecular antioxidants profiling shows lowered glutathione in keratoconus. Exp Eye Res. 2012 Oct;103:41–46. doi: 10.1016/j.exer.2012.07.010
- Galvis V, Sherwin T, Tello A, et al. Keratoconus: an inflammatory disorder? Eye (Lond). 2015 Jul;29(7):843–859. doi: 10.1038/eye.2015.63
- Lema I, Duran JA, Ruiz C, et al. Inflammatory response to contact lenses in patients with keratoconus compared with myopic subjects. Cornea. 2008 Aug;27(7):758–763. doi: 10.1097/ICO.0b013e31816a3591
- Pannebaker C, Chandler HL, Nichols JJ. Tear proteomics in keratoconus. Mol Vis. 2010 Oct 2;16:1949–1957.
- Shetty R, Ghosh A, Lim RR, et al. Elevated expression of matrix metalloproteinase-9 and inflammatory cytokines in keratoconus patients is inhibited by cyclosporine a. Invest Ophthalmol Vis Sci. 2015 Feb 3;56(2):738–750. doi: 10.1167/iovs.14-14831
- Lalgudi VG, Shetty R, Nischal KK, et al. Biochemical and molecular alterations and potential clinical applications of biomarkers in keratoconus. Saudi J Ophthalmol. 2022 Jan;36(1):7–16. doi: 10.4103/SJOPT.SJOPT_203_21
- Malfeito M, Regueiro U, Perez-Mato M, et al. Innate immunity biomarkers for early detection of keratoconus. Ocul Immunol Inflamm. 2019;27(6):942–948. doi: 10.1080/09273948.2018.1511813
- Goncu T, Akal A, Adibelli FM, et al. Tear film and serum prolidase activity and oxidative stress in patients with keratoconus. Cornea. 2015 Sep;34(9):1019–1023. doi: 10.1097/ICO.0000000000000510
- Oltulu R, Katipoglu Z, Gundogan AO, et al. Evaluation of inflammatory biomarkers in patients with keratoconus. Eur J Ophthalmol. 2022 Jan;32(1):154–159. doi: 10.1177/11206721211000644
- Bozkurt E, Ucak T. Serum inflammation biomarkers in patients with keratoconus. Ocul Immunol Inflamm. 2021 Aug 18;29(6):1164–1167. doi: 10.1080/09273948.2020.1741648
- Sobrino T, Regueiro U, Malfeito M, et al. Higher expression of toll-like receptors 2 and 4 in blood cells of keratoconus patiens. Sci Rep. 2017 Oct 11;7(1):12975. doi: 10.1038/s41598-017-13525-7
- Karamichos D, Zieske JD, Sejersen H, et al. Tear metabolite changes in keratoconus. Exp Eye Res. 2015 Mar;132:1–8. doi: 10.1016/j.exer.2015.01.007
- Nishtala K, Pahuja N, Shetty R, et al. Tear biomarkers for keratoconus. Eye Vis (Lond). 2016;3(1):19. doi: 10.1186/s40662-016-0051-9
- Kahan IL, Varsanyi-Nagy M, Toth M, et al. The possible role of tear fluid thyroxine in keratoconus development. Exp Eye Res. 1990 Apr;50(4):339–343. doi: 10.1016/0014-4835(90)90134-G
- Thanos S, Oellers P, Meyer Zu Horste M, et al. Role of thyroxine in the development of keratoconus. Cornea. 2016 Oct;35(10):1338–1346. doi: 10.1097/ICO.0000000000000988
- El-Massry A, Doheim MF, Iqbal M, et al. Association between keratoconus and thyroid gland dysfunction: a cross-sectional case-control study. J Refract Surg. 2020 Apr 1;36(4):253–257. doi: 10.3928/1081597X-20200226-03
- Awad EA, Torky MA, Bassiouny RM, et al. Thyroid gland dysfunction and vitamin D receptor gene polymorphism in keratoconus. Eye (Lond). 2023 Jun;37(8):1602–1607. doi: 10.1038/s41433-022-02172-6
- Aslan MG, Findik H, Okutucu M, et al. Serum 25-hydroxy vitamin D, vitamin B12, and folic acid levels in progressive and nonprogressive Keratoconus. Cornea. 2021 Mar 1;40(3):334–341. doi: 10.1097/ICO.0000000000002475
- Akkaya S, Ulusoy DM. Serum Vitamin D levels in patients with keratoconus. Ocul Immunol Inflamm. 2020 Apr 2;28(3):348–353. doi: 10.1080/09273948.2019.1604002
- Sozer O, Ozalp O, Atalay E, et al. Comparison of blood levels of vitamin B12, folic acid, riboflavin, and homocysteine in keratoconus and healthy subjects. J Cataract Refract Surg. 2023 Jun 1;49(6):589–594. doi: 10.1097/j.jcrs.0000000000001160
- Navel V, Malecaze J, Pereira B, et al. Oxidative and antioxidative stress markers in keratoconus: a systematic review and meta-analysis. Acta Ophthalmol. 2021 Sep;99(6):e777–e794. doi: 10.1111/aos.14714
- Arnal E, Peris-Martinez C, Menezo JL, et al. Oxidative stress in keratoconus? Invest Ophthalmol Vis Sci. 2011 Nov 4;52(12):8592–8597. doi: 10.1167/iovs.11-7732
- Atilano SR, Lee DH, Fukuhara PS, et al. Corneal oxidative damage in keratoconus cells due to decreased oxidant elimination from modified expression levels of SOD Enzymes, PRDX6, SCARA3, CPSF3, and FOXM1. J Ophthalmic Vis Res. 2019 Jan;14(1):62–70. doi: 10.4103/jovr.jovr_80_18
- Balmus IM, Alexa AI, Ciuntu RE, et al. Oxidative stress markers dynamics in keratoconus patients’ tears before and after corneal collagen crosslinking procedure. Exp Eye Res. 2020 Jan;190:107897. doi: 10.1016/j.exer.2019.107897
- Kenney MC, Chwa M, Atilano SR, et al. Increased levels of catalase and cathepsin V/L2 but decreased TIMP-1 in keratoconus corneas: evidence that oxidative stress plays a role in this disorder. Invest Ophthalmol Vis Sci. 2005 Mar;46(3):823–832. doi: 10.1167/iovs.04-0549
- Kilic R, Bayraktar AC, Bayraktar S, et al. Evaluation of serum superoxide dismutase activity, malondialdehyde, and zinc and copper levels in patients with keratoconus. Cornea. 2016 Dec;35(12):1512–1515. doi: 10.1097/ICO.0000000000001018
- Nita M, Grzybowski A. The role of the reactive oxygen species and oxidative stress in the pathomechanism of the age-related ocular diseases and other pathologies of the anterior and posterior eye segments in adults. Oxid Med Cell Longev. 2016;2016:3164734. doi: 10.1155/2016/3164734
- Toprak I, Kucukatay V, Yildirim C, et al. Increased systemic oxidative stress in patients with keratoconus. Eye (Lond). 2014 Mar;28(3):285–289. doi: 10.1038/eye.2013.262
- Shetty R, Sathyanarayanamoorthy A, Ramachandra RA, et al. Attenuation of lysyl oxidase and collagen gene expression in keratoconus patient corneal epithelium corresponds to disease severity. Mol Vis. 2015;21:12–25.
- Buddi R, Lin B, Atilano SR, et al. Evidence of oxidative stress in human corneal diseases. J Histochem Cytochem. 2002 Mar;50(3):341–351. doi: 10.1177/002215540205000306
- Tekin S, Seven E. Assessment of serum catalase, reduced glutathione, and superoxide dismutase activities and malondialdehyde levels in keratoconus patients. Eye (Lond). 2022 Oct;36(10):2062–2066. doi: 10.1038/s41433-021-01753-1
- Elbeyli A, Kurtul BE. Systemic immune-inflammation index, neutrophil-to-lymphocyte ratio, and platelet-to-lymphocyte ratio levels are associated with keratoconus. Indian J Ophthalmol. 2021 Jul;69(7):1725–1729. doi: 10.4103/ijo.IJO_3011_20
- Katipoglu Z, Mirza E, Oltulu R, et al. May monocyte/hdl cholesterol ratio (mhr) and neutrophil/lymphocyte ratio (NLR) Be an Indicator of Inflammation and Oxidative Stress in Patients with Keratoconus? Ocul Immunol Inflamm. 2020 May 18;28(4):632–636. doi: 10.1080/09273948.2019.1611876
- Kilic R, Cumurcu T, Sancaktar E, et al. Systemic prolidase activity and oxidative stress in Keratoconus. Curr Eye Res. 2016;41(1):28–33. doi: 10.3109/02713683.2015.1004717
- Zarei-Ghanavati S, Yahaghi B, Hassanzadeh S, et al. Serum 25-Hydroxyvitamin D, Selenium, Zinc and Copper in Patients with Keratoconus. J Curr Ophthalmol. 2020 Jan;32(1):26–31. doi: 10.1016/j.joco.2019.06.003
- Bamdad S, Owji N, Bolkheir A. Association Between Advanced Keratoconus and Serum Levels of Zinc, Calcium, Magnesium, Iron, Copper, and Selenium. Cornea. 2018 Oct;37(10):1306–1310. doi: 10.1097/ICO.0000000000001661
- Ortak H, Sogut E, Tas U, et al. The relation between keratoconus and plasma levels of MMP-2, zinc, and SOD. Cornea. 2012 Sep;31(9):1048–1051. doi: 10.1097/ICO.0b013e318254c028
- Chwa M, Atilano SR, Hertzog D, et al. Hypersensitive response to oxidative stress in keratoconus corneal fibroblasts. Invest Ophthalmol Vis Sci. 2008 Oct;49(10):4361–4369. doi: 10.1167/iovs.08-1969
- Yildiz E, Aydemir D, Zibandeh N, et al. Investigation of Mitophagy Biomarkers in Corneal Epithelium of Keratoconus Patients. Curr Eye Res. 2022 May;47(5):661–669. doi: 10.1080/02713683.2022.2025846
- Shetty R, Sharma A, Pahuja N, et al. Oxidative stress induces dysregulated autophagy in corneal epithelium of keratoconus patients. PLoS One. 2017;12(9):e0184628. doi: 10.1371/journal.pone.0184628
- Ayilam Ramachandran R, Sanches JM, Robertson DM. The roles of autophagy and mitophagy in corneal pathology: current knowledge and future perspectives. Front Med. 2023;10:1064938. doi: 10.3389/fmed.2023.1064938
- Hardcastle AJ, Liskova P, Bykhovskaya Y, et al. A multi-ethnic genome-wide association study implicates collagen matrix integrity and cell differentiation pathways in keratoconus. Commun Biol. 2021 Mar 1;4(1):266. doi: 10.1038/s42003-021-01784-0
- He W, Han X, Ong JS, et al. Association of Novel Loci with Keratoconus Susceptibility in a Multitrait Genome-Wide Association Study of the UK Biobank Database and Canadian Longitudinal Study on Aging. JAMA Ophthalmol. 2022 Jun 1;140(6):568–576. doi: 10.1001/jamaophthalmol.2022.0891
- Gordon-Shaag A, Millodot M, Shneor E, et al. The genetic and environmental factors for keratoconus. Biomed Res Int. 2015;2015:1–19. doi: 10.1155/2015/795738
- Weed KH, MacEwen CJ, Giles T, et al. The Dundee University Scottish Keratoconus study: demographics, corneal signs, associated diseases, and eye rubbing. Eye (Lond). 2008 Apr;22(4):534–541. doi: 10.1038/sj.eye.6702692
- Georgiou T, Funnell CL, Cassels-Brown A, et al. Influence of ethnic origin on the incidence of keratoconus and associated atopic disease in Asians and white patients. Eye (Lond). 2004 Apr;18(4):379–383. doi: 10.1038/sj.eye.6700652
- Hernandez LM, Blazer DG; Institute of Medicine (US) Committee on Assessing Interactions Among Social, Behavioral, and Genetic Factors in Health. Genes, Behavior, and the Social Environment: Moving Beyond the Nature/Nurture Debate. US: National Academic Press; 2006.
- Gadelha DNB, Feitosa AFB, da Silva RG, et al. Screening for Novel LOX and SOD1 Variants in Keratoconus Patients from Brazil. J Ophthalmic Vis Res. 2020 Apr;15(2):138–148. doi: 10.18502/jovr.v15i2.6730
- Mok JW, So HR, Ha MJ, et al. Association with Corneal Remodeling Related Genes, ALDH3A1, LOX, and SPARC Genes Variations in Korean Keratoconus Patients. Korean J Ophthalmol. 2021 Apr;35(2):120–129. doi: 10.3341/kjo.2020.0138
- Lopes AG, de Almeida GC Jr., Miola MP, et al. Absence of significant genetic alterations in the VSX1, SOD1, TIMP3, and LOX genes in Brazilian patients with Keratoconus. Ophthalmic Genet. 2022 Feb;43(1):73–79. doi: 10.1080/13816810.2021.1992785
- Saravani R, Yari D, Saravani S, et al. Correlation between the COL4A3, MMP-9, and TIMP-1 polymorphisms and risk of keratoconus. Jpn J Ophthalmol. 2017 May;61(3):218–222. doi: 10.1007/s10384-017-0503-3
- Lucas SEM, Zhou T, Blackburn NB, et al. Rare, potentially pathogenic variants in 21 keratoconus candidate genes are not enriched in cases in a large Australian cohort of European descent. PLOS ONE. 2018;13(6):e0199178. doi: 10.1371/journal.pone.0199178
- Rong SS, Ma STU, Yu XT, et al. Genetic associations for keratoconus: a systematic review and meta-analysis. Sci Rep. 2017 Jul 4;7(1):4620. doi: 10.1038/s41598-017-04393-2
- Bykhovskaya Y, Margines B, Rabinowitz YS. Genetics in Keratoconus: where are we? Eye Vis (Lond). 2016;3(1):16. doi: 10.1186/s40662-016-0047-5
- Zadnik K, Steger-May K, Fink BA, et al. Between-eye asymmetry in keratoconus. Cornea. 2002 Oct;21(7):671–679. doi: 10.1097/00003226-200210000-00008
- Li X, Bykhovskaya Y, Canedo AL, et al. Genetic association of COL5A1 variants in keratoconus patients suggests a complex connection between corneal thinning and keratoconus. Invest Ophthalmol Vis Sci. 2013 Apr 12;54(4):2696–2704. doi: 10.1167/iovs.13-11601
- Priyadarsini S, McKay TB, Sarker-Nag A, et al. Keratoconus in vitro and the key players of the TGF-beta pathway. Mol Vis. 2015;21:577–588.
- Engler C, Chakravarti S, Doyle J, et al. Transforming growth factor-β signaling pathway activation in keratoconus. Am J Ophthalmol. 2011 May;151(5):752–759.e2. doi: 10.1016/j.ajo.2010.11.008
- Foster JW, Parikh RN, Wang J, et al. Transcriptomic and immunohistochemical analysis of progressive keratoconus reveal altered WNT10A in epithelium and bowman’s layer. Invest Ophthalmol Vis Sci. 2021 May 3;62(6):16. doi: 10.1167/iovs.62.6.16
- Cuellar-Partida G, Springelkamp H, Lucas SE, et al. WNT10A exonic variant increases the risk of keratoconus by decreasing corneal thickness. Hum Mol Genet. 2015 Sep 1;24(17):5060–5068. doi: 10.1093/hmg/ddv211
- Papa L, Manfredi G, Germain D. SOD1, an unexpected novel target for cancer therapy. Genes Cancer. 2014 Apr;5(1–2):15–21. doi: 10.18632/genesandcancer.4
- Banks CJ, Andersen JL. Mechanisms of SOD1 regulation by post-translational modifications. Redox Biol. 2019 Sep;26:101270. doi: 10.1016/j.redox.2019.101270
- Moschos MM, Kokolakis N, Gazouli M, et al. Polymorphism analysis of VSX1 and SOD1 genes in Greek patients with Keratoconus. Ophthalmic Genet. 2015;36(3):213–217. doi: 10.3109/13816810.2013.843712
- Nejabat M, Naghash P, Dastsooz H, et al. VSX1 and SOD1 Mutation Screening in Patients with Keratoconus in the South of Iran. J Ophthalmic Vis Res. 2017 Apr;12(2):135–140. doi: 10.4103/jovr.jovr_97_16
- Al-Muammar AM, Kalantan H, Azad TA, et al. Analysis of the SOD1 gene in keratoconus patients from Saudi Arabia. Ophthalmic Genet. 2015;36(4):373–375. doi: 10.3109/13816810.2014.889173
- Mok JW, Baek SJ, Joo CK. VSX1 gene variants are associated with keratoconus in unrelated Korean patients. J Hum Genet. 2008;53(9):842–849. doi: 10.1007/s10038-008-0319-6
- Khaled ML, Bykhovskaya Y, Gu C, et al. PPIP5K2 and PCSK1 are candidate genetic contributors to familial keratoconus. Sci Rep. 2019 Dec 18;9(1):19406. doi: 10.1038/s41598-019-55866-5
- Moran S, Gomez L, Zuber K, et al. A case-control study of keratoconus risk factors. Cornea. 2020 Jun;39(6):697–701. doi: 10.1097/ICO.0000000000002283
- Hashemi H, Heydarian S, Hooshmand E, et al. The prevalence and risk factors for keratoconus: a systematic review and meta-analysis. Cornea. 2020 Feb;39(2):263–270. doi: 10.1097/ICO.0000000000002150
- McMonnies CW. Mechanisms of rubbing-related corneal trauma in keratoconus. Cornea. 2009 Jul;28(6):607–615. doi: 10.1097/ICO.0b013e318198384f
- Gunes A, Tok L, Tok O, et al. The youngest patient with bilateral keratoconus secondary to chronic persistent eye rubbing. Semin Ophthalmol. 2015;30(5–6):454–456. doi: 10.3109/08820538.2013.874480
- McMonnies CW, Boneham GC. Keratoconus, allergy, itch, eye-rubbing and hand-dominance. Clinical And Experimental Optometry. 2003 Nov;86(6):376–384. doi: 10.1111/j.1444-0938.2003.tb03082.x
- Diniz CM, Tzelikis PF, Rodrigues Junior A, et al. Unilateral keratoconus associated with continual eye rubbing due to nasolacrimal obstruction–case report. Arq Bras Oftalmol. 2005 Jan;68(1):122–125. doi: 10.1590/S0004-27492005000100022
- Jafri B, Lichter H, Stulting RD. Asymmetric keratoconus attributed to eye rubbing. Cornea. 2004 Aug;23(6):560–564. doi: 10.1097/01.ico.0000121711.58571.8d
- Kandarakis A, Karampelas M, Soumplis V, et al. A case of bilateral self-induced keratoconus in a patient with tourette syndrome associated with compulsive eye rubbing: case report. BMC Ophthalmol. 2011 Sep 21;11(1):28. doi: 10.1186/1471-2415-11-28
- Gasset AR, Houde WL, Garcia-Bengochea M. Hard contact lens wear as an environmental risk in keratoconus. Am J Ophthalmol. 1978 Mar;85(3):339–341. doi: 10.1016/S0002-9394(14)77725-6
- Lopez-de la Rosa A, Gonzalez-Garcia MJ, Calonge M, et al. Tear inflammatory molecules in contact lens wearers: a literature review. CMC. 2020;27(4):523–548. doi: 10.2174/0929867326666190409152921
- Ferdi AC, Nguyen V, Gore DM, et al. Keratoconus natural progression: a systematic review and meta-analysis of 11 529 eyes. Ophthalmol. 2019 Jul;126(7):935–945. doi: 10.1016/j.ophtha.2019.02.029
- Bitirgen G, Ozkagnici A, Malik RA, et al. Evaluation of contact lens-induced changes in keratoconic corneas using in vivo confocal microscopy. Invest Ophthalmol Vis Sci. 2013 Aug 9;54(8):5385–5391. doi: 10.1167/iovs.13-12437
- McMonnies CW, Alharbi A, Boneham GC. Epithelial responses to rubbing-related mechanical forces. Cornea. 2010 Nov;29(11):1223–1231. doi: 10.1097/ICO.0b013e3181d3d660
- Jurkiewicz T, Marty AS. Correlation between Keratoconus and Pollution. Ophthalmic Epidemiol. 2021 Dec;28(6):495–501. doi: 10.1080/09286586.2021.1879173
- Jonas JB, Nangia V, Matin A, et al. Prevalence and associations of keratoconus in rural maharashtra in central India: the central India eye and medical study. Am J Ophthalmol. 2009 Nov;148(5):760–765. doi: 10.1016/j.ajo.2009.06.024
- Spoerl E, Raiskup-Wolf F, Kuhlisch E, et al. Cigarette smoking is negatively associated with keratoconus. J Refract Surg. 2008 Sep;24(7):S737–40.
- Kristianslund O, Drolsum L. Prevalence of keratoconus in persons with Down syndrome: a review. BMJ Open Ophthalmol. 2021;6(1):e000754. doi: 10.1136/bmjophth-2021-000754
- Fransen E, Valgaeren H, Janssens K, et al. Resequencing of candidate genes for Keratoconus reveals a role for Ehlers-Danlos Syndrome genes. Eur J Hum Genet. 2021 Dec;29(12):1745–1755. doi: 10.1038/s41431-021-00849-2
- McMahon TT, Kim LS, Fishman GA, et al. CRB1 gene mutations are associated with keratoconus in patients with leber congenital amaurosis. Invest Ophthalmol Vis Sci. 2009 Jul;50(7):3185–3187. doi: 10.1167/iovs.08-2886
- Gupta PK, Stinnett SS, Carlson AN. Prevalence of sleep apnea in patients with keratoconus. Cornea. 2012 Jun;31(6):595–599. doi: 10.1097/ICO.0b013e31823f8acd
- Pellegrini M, Bernabei F, Friehmann A, et al. Obstructive sleep apnea and keratoconus: a systematic review and meta-analysis. Optom Vis Sci. 2020 Jan;97(1):9–14. doi: 10.1097/OPX.0000000000001467
- Pihlblad MS, Schaefer DP. Eyelid laxity, obesity, and obstructive sleep apnea in keratoconus. Cornea. 2013 Sep;32(9):1232–1236. doi: 10.1097/ICO.0b013e318281e755
- McKay TB, Priyadarsini S, Karamichos D. Sex Hormones, Growth Hormone, and the Cornea. Cells. 2022 Jan 11;11(2):224. doi: 10.3390/cells11020224
- Bilgihan K, Hondur A, Sul S, et al. Pregnancy-induced progression of keratoconus. Cornea. 2011 Sep;30(9):991–994. doi: 10.1097/ICO.0b013e3182068adc
- Hoogewoud F, Gatzioufas Z, Hafezi F. Transitory topographical variations in keratoconus during pregnancy. J Refract Surg. 2013 Feb;29(2):144–146. doi: 10.3928/1081597X-20130117-11
- Naderan M, Jahanrad A. Topographic, tomographic and biomechanical corneal changes during pregnancy in patients with keratoconus: a cohort study. Acta Ophthalmol. 2017 Jun;95(4):e291–e296. doi: 10.1111/aos.13296
- Soeters N, Tahzib NG, Bakker L, et al. Two cases of keratoconus diagnosed after pregnancy. Optom Vis Sci. 2012 Jan;89(1):112–116. doi: 10.1097/OPX.0b013e318238c3f2
- Asim A, Kumar A, Muthuswamy S, et al. Down syndrome: an insight of the disease. J Biomed Sci. 2015 Jun 11;22(1):41. doi: 10.1186/s12929-015-0138-y
- Mathan JJ, Simkin SK, Gokul A, et al. Down syndrome and the eye: Ocular characteristics and ocular assessment. Surv Ophthalmol. 2022 Nov;67(6):1631–1646. doi: 10.1016/j.survophthal.2022.03.006
- Alio JL, Vega-Estrada A, Sanz P, et al. Corneal morphologic characteristics in patients with down syndrome. JAMA Ophthalmol. 2018 Sep 1;136(9):971–978. doi: 10.1001/jamaophthalmol.2018.2373
- Romero-Jimenez M, Santodomingo-Rubido J, Wolffsohn JS. Keratoconus: a review. Cont Lens Anterior Eye. 2010 Aug;33(4):157–166; quiz 205.doi: 10.1016/j.clae.2010.04.006
- Evereklioglu C, Yilmaz K, Bekir NA. Decreased central corneal thickness in children with down syndrome. J Pediatr Ophthalmol Strabismus. 2002 Sep;39(5):274–277. doi: 10.3928/0191-3913-20020901-07
- Edwards M, Cn M, Dean S. The genetics of keratoconus. Clin Exp Ophthalmol. 2001 Dec;29(6):345–351. doi: 10.1046/j.1442-9071.2001.d01-16.x
- Cescon M, Gattazzo F, Chen P, et al. Collagen VI at a glance. J Cell Sci. 2015 Oct 1;128(19):3525–3531. doi: 10.1242/jcs.169748
- Loukovitis E, Sfakianakis K, Syrmakesi P, et al. Genetic aspects of keratoconus: a literature review exploring potential genetic contributions and possible genetic relationships with comorbidities. Ophthalmol Ther. 2018 Dec;7(2):263–292. doi: 10.1007/s40123-018-0144-8
- Robertson I. Keratoconus and the Ehlers-Danlos syndrome: a new aspect of keratoconus. Med J Aust. 1975 May 3;1(18):571–573. doi: 10.5694/j.1326-5377.1975.tb111590.x
- De Paepe A, Malfait F. The Ehlers-Danlos syndrome, a disorder with many faces. Clin Genet. 2012 Jul;82(1):1–11. doi: 10.1111/j.1399-0004.2012.01858.x
- Kumaran N, Moore AT, Weleber RG, et al. Leber congenital amaurosis/early-onset severe retinal dystrophy: clinical features, molecular genetics and therapeutic interventions. Br J Ophthalmol. 2017 Sep;101(9):1147–1154. doi: 10.1136/bjophthalmol-2016-309975
- Yoganathan S, Kumar M, John D, et al. Oculodigital sign: a clinical clue for diagnosis. Ann Indian Acad Neurol. 2022 May;25(3):560–561. doi: 10.4103/aian.aian_755_21
- Elder MJ. Leber congenital amaurosis and its association with keratoconus and keratoglobus. J Pediatr Ophthalmol Strabismus. 1994 Jan;31(1):38–40. doi: 10.3928/0191-3913-19940101-08
- Dharmaraj S, Leroy BP, Sohocki MM, et al. The phenotype of Leber congenital amaurosis in patients with AIPL1 mutations. Arch Ophthalmol. 2004 Jul;122(7):1029–1037. doi: 10.1001/archopht.122.7.1029
- Medicine AAo S. International classification of sleep disorders. 3rd ed. Darien (IL): American Academy of Sleep Medicine; 2014.
- Mojon DS, Goldblum D, Fleischhauer J, et al. Eyelid, conjunctival, and corneal findings in sleep apnea syndrome. Ophthalmol. 1999 Jun;106(6):1182–1185. doi: 10.1016/S0161-6420(99)90256-7
- Saidel MA, Paik JY, Garcia C, et al. Prevalence of sleep apnea syndrome and high-risk characteristics among keratoconus patients. Cornea. 2012 Jun;31(6):600–603. doi: 10.1097/ICO.0b013e318243e446
- Carlson AN. Keratoconus. Ophthalmol. 2009 Oct;116(10):2036–2037; author reply 2037-8. doi: 10.1016/j.ophtha.2009.08.026
- Lavinsky F, Lavinsky D, Lavinsky J. Novel perspectives on keratoconus and corneal collagen cross-linking. J Refract Surg. 2016;32(11):728–734.
- Whelchel AE, McKay TB, Priyadarsini S, et al. Association between Diabetes and Keratoconus: A Retrospective Analysis. Sci Rep. 2019 Sep 24;9(1):13808. doi: 10.1038/s41598-019-50095-2
- Ates KM, Estes AJ, Liu Y. Potential underlying genetic associations between keratoconus and diabetes mellitus. Adv Ophthalmol Pract Res. 2021 Nov 1;1(1):100005. doi: 10.1016/j.aopr.2021.100005
- Kuo IC, Broman A, Pirouzmanesh A, et al. Is there an association between diabetes and keratoconus? Ophthalmol. 2006 Feb;113(2):184–190. doi: 10.1016/j.ophtha.2005.10.009
- Naderan M, Naderan M, Rezagholizadeh F, et al. Association between diabetes and keratoconus: a case-control study. Cornea. 2014 Dec;33(12):1271–1273. doi: 10.1097/ICO.0000000000000282
- Seiler T, Huhle S, Spoerl E, et al. Manifest diabetes and keratoconus: a retrospective case-control study. Graefes Arch Clin Exp Ophthalmol. 2000 Oct;238(10):822–825. doi: 10.1007/s004179900111
- Woodward MA, Blachley TS, Stein JD. The association between sociodemographic factors, common systemic diseases, and keratoconus: an analysis of a nationwide health care claims database. Ophthalmol. 2016 Mar;123(3):457–65 e2. doi: 10.1016/j.ophtha.2015.10.035
- Kosker M, Suri K, Hammersmith KM, et al. Another look at the association between diabetes and keratoconus. Cornea. 2014 Aug;33(8):774–779. doi: 10.1097/ICO.0000000000000167
- Moon JY, Lee J, Park YH, et al. Incidence of keratoconus and its association with systemic comorbid conditions: a nationwide cohort study from south korea. J Ophthalmol. 2020;2020:3493614. doi: 10.1155/2020/3493614
- Nemet AY, Vinker S, Bahar I, et al. The association of keratoconus with immune disorders. Cornea. 2010 Nov;29(11):1261–1264. doi: 10.1097/ICO.0b013e3181cb410b
- Claessens JLJ, Godefrooij DA, Vink G, et al. Nationwide epidemiological approach to identify associations between keratoconus and immune-mediated diseases. Br J Ophthalmol. 2022 Oct;106(10):1350–1354. doi: 10.1136/bjophthalmol-2021-318804
- Kocak Altintas AG, Gul U, Duman S. Bilateral keratoconus associated with Hashimoto’s disease, alopecia areata and atopic keratoconjunctivitis. Eur J Ophthalmol. 1999 Apr;9(2):130–133. doi: 10.1177/112067219900900210
- Rahi A, Davies P, Ruben M, et al. Keratoconus and coexisting atopic disease. Br J Ophthalmol. 1977 Dec;61(12):761–764. doi: 10.1136/bjo.61.12.761
- Wajnsztajn D, Solomon A. Vernal keratoconjunctivitis and keratoconus. Curr Opin Allergy Clin Immunol. 2021 Oct 1;21(5):507–514. doi: 10.1097/ACI.0000000000000765
- Lee HK, Jung EH, Cho BJ. Epidemiological association between systemic diseases and keratoconus in a korean population: a 10-year nationwide cohort study. Cornea. 2020 Mar;39(3):348–353. doi: 10.1097/ICO.0000000000002206
- Baigrie D, Goyal A, Crane JS. Leukocytoclastic Vasculitis. Treasure Island (FL): StatPearls; 2023.
- Coco G, Kheirkhah A, Foulks GN. Keratoconus and leukocytoclastic vasculitis. Cornea. 2008;27(4):487–489.