Abstract
Much research has been carried out to understand how human brains make sense of another agent in motion. Current views based on human adult and monkey studies assume a matching process in the motor system biased toward actions performed by conspecifics and present in the observer's motor repertoire. However, little is known about the neural correlates of action cognition in early ontogeny. In this study, we examined the processes involved in the observation of full body movements in 4-month-old infants using functional near-infrared spectroscopy to measure localized brain activation. In a 2 × 2 design, infants watched human or robotic figures moving in a smooth, familiar human-like manner, or in a rigid, unfamiliar robot-like manner. We found that infant premotor cortex responded more strongly to observe robot-like motion compared with human-like motion. Contrary to current views, this suggests that the infant motor system is flexibly engaged by novel movement patterns. Moreover, temporal cortex responses indicate that infants integrate information about form and motion during action observation. The response patterns obtained in premotor and temporal cortices during action observation in these young infants are very similar to those reported for adults. These findings thus suggest that the brain processes involved in the analysis of an agent in motion in adults become functionally specialized very early in human development.
The observation of bodies in motion provides an exceedingly complex and rich set of dynamic information about the actions of other agents. In the cognitive and brain sciences, much research activity has been dedicated to examine the question of how actions are represented and understood (Blake & Shiffrar, Citation2007; Grèzes and Decety, Citation2001; Jeannerod, Citation2001; Prinz, Citation1990; Rizzolatti & Craighero, Citation2004; Rizzolatti & Sinigaglia, Citation2010). This work has shown that different aspects of an observed action are processed and represented within at least two different modes (Jeannerod, Citation2006). On the one hand, observed actions are processed as visual events that can be perceptually described and recognized. On the other hand, actions are also processed as motor events represented in a sequence of motor commands that can be learned and reproduced. These two modes of processing map onto distinct brain regions in humans and monkeys. Although the visual content of an action is mostly represented by high-level visual neurons in the temporal cortex, specifically the superior temporal sulcus (STS), the motor content of the action is analyzed in the frontal cortex, specifically the premotor cortex (Jeannerod, Citation2006). Notwithstanding these differences between the modes of processing the visual content and the motor content of an observed action, the two modes can be engaged simultaneously and information might be exchanged between modes in the service of action recognition, prediction, and understanding (Keysers & Gazzola, Citation2007; Kilner, Friston, & Frith, Citation2007a, Citation2007b).
More specifically, the STS has been found to play a critical role in visual social perception as it is preferentially engaged during the processing of biological motion compared with nonbiological motion (Allison, Puce, & McCarthy, Citation2000). Moreover, the STS is considered a convergence zone between the ventral and dorsal visual streams (Vaina, Solomon, Chowdhury, Sinha, & Belliveau, Citation2001) and has been shown to integrate information about form and motion for the representation of biological percepts and actions (Kourtzi, Krekelberg, & van Wezel, Citation2008). Complementarily, the premotor cortex is an important part of the mirror neuron system (MNS), which refers to inferior parietal and premotor cortical regions in monkeys and humans that are active during both action production and action observation. In humans, considerable research attention has been paid to explore the properties of an extended human MNS that includes superior temporal and lateral occipital cortices. This broader complement of brain regions engaged when perceiving others in motion has been termed the action observation network (AON). The AON has been proposed to enable an observer to understand another agent's action by simulating it in his or her own motor system (Jeannerod, Citation2006; Rizzolatti & Craighero, Citation2004).
The dominant view of the AON posits that brain regions like the premotor cortex respond most robustly to familiar and executable movements that are in some manner “like me” (Rizzolatti & Craighero, Citation2004). The human AON has been shown to respond more strongly to human than animal actions, and more strongly to physically familiar actions than unfamiliar actions (Cross, Hamilton, & Grafton, Citation2006). Thus, the recruitment of the AON is generally thought to indicate the spontaneous simulation of actions that are familiar and present in the observer's motor repertoire. However, contrary to this “like me”-view of the AON, there is work showing that this network is also involved in the processing of non-human actions, suggesting a much greater flexibility and a more general involvement of the motor system in the observation of a wide variety of actions, ranging from familiar to highly unfamiliar (Gazzola, Rizzolatti, Wicker, & Keysers, Citation2007; Ramsey & Hamilton, Citation2010).
To further address this issue, with two complementary functional magnetic resonance imaging (fMRI) experiments performed with healthy young adults, Cross and colleagues (Citation2011) tested whether activation of the AON depends on familiarity with the form or motion of a given agent. In this study, the authors directly compared brain activation during the observation of human or robotic figures moving in a familiar human-like manner or an unfamiliar robot-like manner, while controlling for low-level differences such as how much each agent moved during any given sequence. This study revealed that, regardless of form, the premotor cortex responds more robustly to unfamiliar robotic compared with familiar human movement, challenging the notion that the motor system is biased toward familiar and executable actions with which the observer has prior motor experience. The authors suggest that these findings might be best explained by an account in which not only the observation of highly familiar actions might lead to strong AON engagement but also the observation of highly novel and less predictable actions, though for different reasons. In the latter context, greater activation of the AON can reflect increased demands to predict and understand atypical motion patterns that cannot easily be assimilated into familiar biological motion representations (for a more complete discussion, see Cross et al., Citation2011).
Another important finding from Cross et al.'s (2011) study was that there was one region in the left STS that showed an interaction between form and motion cues. Namely, this region responded preferentially when the human figure moved like a human and when the robot figure moved like a robot. This suggests that this region might be sensitive to the congruence between the agents' form and motion, supporting the notion that this region plays a critical role in the integration of information processed by the ventral (form) and dorsal (motion) visual pathways. It is important to note that this integration occurred despite the fact that the robot form and motion were both unfamiliar to the observer. All in all, Cross et al.'s (2011) study provides evidence that in human adults, action observation flexibly engages the motor system and the visual system. The different response properties of these two systems indicate that the motor system and the visual system might play different but potentially complementary roles during the observation of action.
A promising and novel way toward understanding the brain systems that are involved in the observation of action is to investigate their roles in development (Gallese, Rochat, Cossu, & Sinigaglia, Citation2009; Marshall & Meltzoff, Citation2011). Therefore, it is of particular interest to investigate action observation in infancy, the earliest period of postnatal development, during which new perceptual and motor skills are acquired. So far, previous research on infants' perception of full body motion, as an important element of action observation, has primarily been based on methods examining their looking behavior. This line of research has revealed that infants are sensitive to biological motion when compared with nonbiological motion from very early in ontogeny. For example, newborn infants prefer to look at the display of a walking point-light chicken compared with a scrambled and inverted walking point-light chicken (Simion, Regolin, & Bulf, Citation2008). Furthermore, at the age of 3 months, infants discriminate between biologically possible and impossible displays of human point-light walkers (Bertenthal, Proffitt, & Kramer, Citation1987). At the same age, infants distinguish between biologically possible and impossible moving point-light spiders and cats (Pinto, Citation1994). This behavioral work shows that infants develop the basic but important ability to discriminate between biological and nonbiological forms of movement.
In this study, we examined the brain basis of action observation in young infants. In a 2 × 2 design, we presented 4-month-old infants with human or robotic figures moving in a smooth, familiar human-like manner (henceforth called human-like motion), or in a rigid, unfamiliar robot-like manner (henceforth called robot-like motion), adapting Cross et al.'s (2011) design. This age group was chosen because, from our extensive experience in conducting electroencephalography (EEG) and functional near-infrared spectroscopy (fNIRS) experiments with infants, this is the youngest age group that can be successfully tested in a visual experimental procedure of this kind (Grossmann & Johnson, Citation2010; Grossmann, Johnson, Lloyd-Fox, Blasi, Deligianni, Elwell, & Csibra, Citation2008; Grossmann, Parise, & Friederici, Citation2010). Furthermore, at this young age, infants do not have any experience in performing the shown movements themselves; hence, it allowed us to examine the brain processes involved in movement observation of an agent before motor experience with the particular movement exists. This study used fNIRS permitting spatial localization of brain activation by measuring hemodynamic responses (see Lloyd-Fox, Blasi, and Elwell (Citation2010) for a review). fNIRS is better suited for infant research than fMRI because it can accommodate a good degree of movement from the infants, enabling them to sit upright on their parent's lap and behave relatively freely while watching or listening to certain stimuli. Despite its inferior spatial resolution, fNIRS, like fMRI, measures localized patterns of hemodynamic responses, thus allowing for a comparison of infant fNIRS data with adult fMRI data (see Strangman, Culver, Thompson, & Boas, Citation2002).
First, in this study, we addressed the question of whether the motor portion of the AON (specifically the premotor cortex) is involved during the observation of action in young infants. There is already some evidence from older infants suggesting that this might be the case during the observation of reaching actions (Shimada & Hiraki, Citation2006; Southgate, Johnson, El Karoui, & Csibra, Citation2010; Southgate, Johnson, Osborne, & Csibra, Citation2009). For example, Southgate and colleagues (Citation2009) showed that 9-month-old infants display a reduction in EEG mu activity over sensorimotor cortex, indexing motor activation, during performing and observing grasping actions. But, importantly, our study goes beyond this question by examining whether activity in the motor system depends on familiarity with motion and/or form of the agent. That prior experience and familiarity with an action plays a role in the recruitment of the motor system has been shown by Stapel and colleagues (2010) using EEG, who found that 12-month-old infants showed greater motor activation (larger reduction of mu activity) during the observation of unfamiliar actions (e.g., cup to ear) than familiar actions (e.g., cup to mouth). However, it is important to note that, in another study, 14- to 16-month-old infants exhibited greater motor activation during the observation of a familiar (crawling) actions when compared with less familiar (walking) actions (van Elk, van Schie, Hunnius, Vesper, & Bekkering, Citation2008). Although these prior infant EEG studies seem to have yielded conflicting patterns of results, they are in line with recent adult fMRI work discussed above (Cross et al., Citation2011). These authors adopt a Bayesian perspective to explain such findings (after Kilner et al., Citation2007a, Citation2007b), suggesting that observation of highly familiar actions leads to strong activation of the motor system; this is due to small deviations from extremely precise motor priors (gained by extensive physical or visual experience). Conversely, observation of highly unusual or unfamiliar actions can also lead to robust motor system activity, but in this case, activity is being driven by a lack of motor priors, and the fact that sensorimotor cortices are highly engaged when trying to process (or predict or learn from) actions with which the observer has no prior experience.
Second, we investigated the role of the infants' temporal cortex in processing of action. Prior infant fNIRS work has shown that 5-month-old infants showed increased activation in response to biological human motion (eye, mouth, and hand movements) in posterior superior temporal cortex when compared with nonbiological motion (clips of machine cogs and pistons and moving mechanical toys) (Lloyd-Fox et al., Citation2009; see Lloyd-Fox, Blasi, Everdell, Elwell, and Johnson (Citation2011) for more information concerning the cortical selectivity for eye, mouth, and hand movements). Here, we asked whether young infants are able to integrate visual information about form and motion while processing an agents' action and whether this depends on familiarity. Critically, because our infant fNIRS study is closely modeled after a previous adult fMRI study (Cross et al., Citation2011), it allowed for a developmental comparison of action processing between infants and adults.
MATERIALS AND METHODS
Participants
Fifteen 4-month-old infants were included in the final sample (8 girls, range 113–129 days, M = 122 days). Ten additional infants were tested but not included in the final sample because of fussiness (N = 5) or too many motion artifacts (N = 5). All infants watched a minimum of five trials per experimental condition. The required minimum number of artifact-free trials per condition was three (see data analysis for artifact treatment). Note that an attrition rate at this level is within the normal range for an infant fNIRS study (Lloyd-Fox et al., Citation2010). All were full-term infants (37–42 weeks gestation) and with normal birth weight (>2500 g). All parents gave informed consent before the study.
Stimuli and procedure
A subset of four videos from the Cross et al.'s (2011) study was used as stimuli for this study. Each video was 7.7 s in length and fell into one cell of the two-by-two factorial design, with form and motion as factors and robotic and human motions as the levels of each factor. Two videos featured a professional break-dancer dancing in a familiar, natural, free-style manner (see Supplementary Video 1) or in an unfamiliar, rigid, robotic manner, known as “dancing the robot” (Supplementary Video 2). Importantly, the videos were not altered in any way—the dancer was simply instructed to dance naturally and dance robotically. The dancer performed to music that was of equivalent tempo across both dance styles. The remaining two videos were created with a Lego Bionicle™ action figure (model 7117, name: Gresh) and stop-motion animation, using Frame-by-Frame software. (http://web.mac.com/philipp.brendel/Software/FrameByFrame.html). The videos were made by matching the Lego figure's limbs to the positions of the human dancer's limbs. This matching process was performed by overlaying real-time video of the Lego figure onto the prerecorded video of the human dancer. The original videos of the human dancer were advanced frame by frame, and the Lego figure's posture was adjusted to match the human's for each video frame. As the human videos were recorded at a rate of 25 frames per second, this resulted in a total of 193 static images of the Lego figure, which, when played back at the rate of 25 frames per second, precisely matched the human videos in duration. This resulted in two frame-matched videos featuring the Lego form: one with the Lego form moving in a natural human dance style (Supplementary Video 3) and one with the Lego form moving in a robotic dance style (Supplementary Video 4).
Importantly, it should be noted that while the videos feature both biological (human) and nonbiological (Lego robot) agents performing, the movements themselves are not designed to be perfectly biological and nonbiological. Instead, we emphasize that the smooth, human-like movement represents a more familiar type of action, whereas the rigid, robot-like movement represents a more unfamiliar type of action.
Infants sat in a special seat that supports body posture and reduces leg movements on their parent's lap while watching the stimuli on a 17″ computer monitor at a distance of 80 cm within an acoustically shielded, dimly lit room. The experimental session consisted of 7-s-long trials. The four experimental conditions were distributed pseudo-randomly over the session with no more than two trials of the same condition occurring in a row. We controlled the stimulus presentation such that only when the infant was sitting still and attending to the screen the experimenter would then press a key to play an alerting sound and start the video stimulus. This procedure allowed us to ascertain that the infants were paying attention to the stimuli and it also reduced the amount of movement at stimulus onset. The intertrial interval varied randomly between 8 s and 12 s. Nonsocial moving visual stimuli (abstract screen savers) were presented during the intertrial interval to keep infants' attention.
Data acquisition and analysis
The fNIRS method relies on the optical determination of changes in hemoglobin concentrations in cerebral cortex that result from increased regional cerebral blood flow. Cortical activations were measured using a Hitachi ETG-4000 NIRS system. Two wavelengths were set at 695 nm and 830 nm for all the recording channels. Using an EEG Easycap (www.easycap.de), two custom-built arrays consisting of nine optodes (five sources, four detectors) in a 12-channel (source- detector pairs) arrangement with an inter-optode separation of 20 mm were placed over temporal and frontal brain regions on each hemisphere (see for channel layout with reference to the EEG electrodes). The EEG cap was then placed on the infants' head on the basis of the commonly used anatomical landmarks (nasion and inion). This resulted in a relatively broad coverage of the frontal and temporal regions in both hemispheres by the 24-NIRS channels (see ). fNIRS data were continuously sampled at 10 Hz. After calculation of the oxyHb and deoxyHb concentration changes, pulse-related signal changes and overall trends were eliminated by low-pass filtering (Butterworth, 5th order, lower cutoff 0.5 Hz). Movement artifacts were corrected by an established procedure (see Koch, Steinbrink, Villringer, & Obrig, Citation2006; Wartenburger et al., Citation2007), which allows marking of artifacts and then padding the contaminated data segments by linear interpolation. This padding of movement-contaminated data was only used for artifact removal during the baseline period and the first 2 s after trial onset (video onset). If there was a movement artifact during the experimental trial, then the trial was not included in the analysis. After visual inspection of the time course of the concentration changes, a time window around the peak of hemodynamic response (between 4 s and 8 s after stimulus onset) was chosen for statistical analysis (see Figure S1 for time courses of the hemodynamic responses for selected channels). Cortical responses were assessed by comparing average concentration changes (oxyHb) within trials for this time window between experimental conditions. None of the deoxyHb changes reached statistical significance. In a recent review of the infant fNIRS literature (Lloyd-Fox et al., Citation2010), it has been shown that the majority of infant studies used oxyHb as the main measure for cortical activation, probably because of a better signal-to-noise ratio (SNR) for the oxyHb when compared with the deoxyHb results. The fact that we did not find any significant decreases in deoxyHb that accompanied the increase in oxyHb, as one would expect on the basis of adult work (Obrig & Villringer, Citation2003), is in line with some of the prior infant fNIRS work. Namely, a considerable number of infant fNIRS studies (for detailed information, see Lloyd-Fox et al., Citation2010) either failed to find a significant decrease or even observed an increase in deoxyHb concentration. Although a variety of factors such as the choice of wavelengths used or the physiological immaturity of the infant brain have been suggested to explain this difference between infant and adult studies, the exact nature of this difference remains an open question (for a discussion, see Lloyd-Fox et al., Citation2010).
Figure 1. Hemodynamic brain responses (oxyHb in mmol/L) measured in 4-month-old infants during action observation. Regions of interest (ROIs) used for our analysis are marked on the schematic infant head model (ANT = anterior ROI, POS = posterior ROI, INF = inferior ROI, SUP = superior ROI). This graph depicts mean oxygenated hemoglobin concentration changes (±SEM) in anterior ROI-premotor (a and b) and the inferior ROI-temporal (d and e) brain regions during the four experimental conditions (form: human vs. robot; motion: human vs. robot). Channels that were summarized to regions of interest and used to calculate the mean oxygenated concentration changes are marked on the head model (c) for each hemisphere.
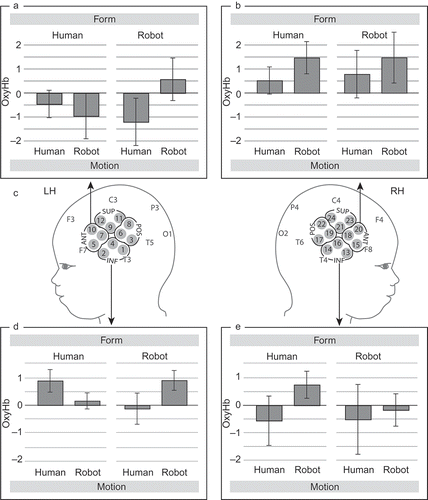
An omnibus repeated measure ANOVA with within-subjects factor—region of interest (ROI) (anterior, posterior, inferior, superior) × hemisphere (left, right) × form (human, robot) × motion (human, robot)—were used in order to compare between conditions (see for channel groupings included in the four regions of interest (ROIs)). In addition, repeated measure ANOVAs with within-subjects factors—hemisphere (left, right) × form (human, robot) × motion (human, robot)—were performed for each ROI separately. The ROIs were chosen to best capture the premotor (anterior ROI in our analysis) and superior temporal regions (inferior ROI in our analysis) that were found to be differentially involved during action observation in the prior fMRI study with adults (see Cross et al., Citation2011) that our infant fNIRS study was based upon. Importantly, the chosen anterior ROI also approximately corresponds to a region identified in a prior fNIRS study with 6- to 7-month-old infants (Shimada & Hiraki, Citation2006), in which activation during infants' own movements was obtained. Note that this selection of ROIs is constrained by the geometry and number of channels available from the fNIRS probes of the HITACHI ETG 4000 system (in our case, 12 channels in each hemisphere) and that such a clustering of channels has been utilized in prior fNIRS research with infants (Gervain, Macagno, Cogoi, Pena, & Mehler, Citation2008; Pena et al., Citation2003). Moreover, the clustering of channels was performed so that a small but equal number of channels, i.e., three channels, were included in each predefined ROI.
RESULTS
Effect of type of motion
Our omnibus analysis revealed a significant interaction between the two factors ROI and motion, F (3, 39) = 3.588, p = .022. The region-specific analysis showed that this interaction was explained by an effect at the anterior ROI (corresponding to the premotor cortex). In this region, regardless of the form of the agent, activation was significantly increased in response to unfamiliar robot-like motion when compared with the familiar human-like motion, F (1, 13) = 4.806, p = .047 (see ; for fNIRS data from the posterior and superior ROIs; see Figure S2 in the supplementary material). For the anterior ROI, there was no interaction between the factors motion and hemisphere, F (1, 13) = 0.193, p = .529, and thus no evidence for a lateralization of the observed effect of motion.
Interaction between form and motion
Moreover, the analysis for the inferior ROI (corresponding to the superior temporal cortex including the STS) revealed a significant three-way interaction between the factors hemisphere, form, and motion, F (1, 13) = 6.458, p = .025. Specifically, only the left hemisphere showed a significant interaction between form and motion, F (1, 14) = 6.108, p = .027. As shown in , this region in the left hemisphere showed significantly increased activation for congruent pairings (a human figure moving in a familiar human-like manner and a robot figure moving in an unfamiliar robot-like manner) when compared with incongruent pairings of form and motion (a human figure moving in an unfamiliar robot-like manner and a robot figure moving in a familiar human-like manner). This effect can also be seen as a main effect of congruence between form and motion, such that when a repeated measures ANOVA is conducted with the within-subject factor congruence then a main effect of congruence, F (1, 14) = 6.108, p = .027, is obtained with the same statistical values as for the interaction reported above.
DISCUSSION
In this study, we investigated the processes involved in the observation of action in 4-month-old infants using fNIRS. Our results show that, already by the age of 4 months, infants differentially engage temporal as well as frontal (premotor) cortical regions when processing an agent's action. Specifically, the pattern of infants' brain responses indicates that they are sensitive to the form and motion characteristics of an agent. Importantly, the response patterns obtained in premotor and temporal cortices during action observation in these young infants are strikingly similar to those found with fMRI in adults using the identical stimulus material (Cross et al., Citation2011). These findings thus suggest that the brain regions involved in the perceptual and motor analysis of an action in adults become functionally specialized very early in human development.
Contrary to the dominant view, which stipulates that brain regions like the premotor cortex respond preferentially to familiar and executable action (Rizzolatti & Craighero, Citation2004; Rizzolatti & Sinigaglia, Citation2010), our infant fNIRS data show that, consistent with what has been described in a recent study using fMRI with adults (Cross et al., Citation2011), an area of the infant brain likely corresponding to premotor cortex responded stronger to unfamiliar robot-like motion than familiar human-like motion. It is also interesting to note that this increased premotor cortex involvement during the perception of robot-like motion does not depend on whether it was presented in the context of a familiar human or an unfamiliar robot figure, indicating that it is solely the motion patterns that drive the response in the premotor cortex. This kind of robotic motion is neither visually familiar to nor executable by the infant. However, it results in increased activation of the premotor region, suggesting that, contrary to conventional views, activation of the premotor cortex cannot be taken as an indicator for spontaneous simulation of an action present in the observer's motor repertoire. These findings might be best accounted for by the Bayesian framework introduced above in which the observation of highly novel actions imposes greater demands on the motor system to predict or learn from actions with which the observer lacks prior physical experience, thus resulting in more motor system activity when viewing highly unfamiliar compared with relatively familiar actions (Cross et al., Citation2011). This notion is in line with recent empirical findings from 12-month-old infants (Stapel et al., Citation2010) and with theories that implicate the motor system more generally in action prediction (Kilner et al., Citation2007a, Citation2007b; Schubotz, Citation2007) rather than specifically in a process of action mirroring via direct mapping (Avenanti, Sirigu, & Aglioti, Citation2010; Buccino et al., Citation2004; Tai, Scherfler, Brooks, Sawamoto, & Castiello, Citation2004). However, we must caution that such conclusions remain speculative at this stage and will require additional thorough follow-up studies with both developing and adult brains to validate such a generalized theory of premotor involvement in action prediction.
Another intriguing finding from this study is that 4-month-old infants appear to be sensitive to the relationship between the form and the motion of an agent. More specifically, they seem to be able to integrate information about form and motion during action observation, as indicated by their temporal cortex responses. Infants' temporal cortex responses were similar in their response properties, location, and lateralization to what was previously shown in adults (Cross et al., Citation2011). The results suggest that a region in the left hemisphere was preferentially engaged during the processing of congruent form and motion information, that is, during trials when infants saw a human figure moving like a human and a robot figure moving like a robot. The ability to match human form to human motion might be explained by the visual experience that infants have in observing human action. However, it is more challenging to explain the surprising finding that infants appear to be able to detect the congruence between robot form and motion, based on the temporal cortex responses. One possible explanation might be that infants' brain responses are a result of an associative mechanism by which familiar form is associated with familiar motion (human case) whereas an unfamiliar form is likely to be associated with an unfamiliar motion (robot case). This is only a speculative suggestion of what may explain the temporal cortex responses, but more work is needed to clarify the exact mechanism that underlies this phenomenon. Irrespective of the exact nature of the underlying mechanism, infants' temporal brain responses indicate that they are sensitive to the relation between the form and the motion of an agent, which is considered to be an important social perceptual skill. The lateralization of infants' temporal cortex response to the left hemisphere is consistent with findings from adults, demonstrating that this part of the left hemisphere is part of multimodal association area (Decety & Somerville, Citation2003). Another complementary but rather speculative interpretation of this left-lateralized effect in infants might be derived from the postulated links between action observation and language processing in the human brain (Aziz-Zadeh, Maeda, Zaidel, Mazziotta, & Iacoboni, Citation2002; Pulvermueller, Citation2005). According to this framework, the left-lateralized effect seen in infants' action observation might be considered an early precursor to left-lateralized brain functions that are later shared by language and action processing.
The observation of actions provides the infant with a rich set of dynamic information about the behavior of other agents. Our study demonstrates that different aspects of an observed action are represented within at least two different modes in the 4-month-old infants' brain: (1) infants process observed actions as dynamic visual events that are perceptually analyzed in terms of form and motion within the temporal cortex and (2) they also process observed action as motor events that are analyzed in terms of their motor properties and predictability within the frontal (premotor) cortex. These findings support the notion that action observation affords dual processing modes that map onto distinct brain regions (Jeannerod, Citation2006), an insight that so far was mainly based on the work with human adults and monkeys. Critically, the fNIRS data show that infants recruit these processing mechanisms flexibly and in an adult-like manner at an age at which they have little or no experience with the observed actions. In conclusion, using a novel paradigm and a modern optical imaging technique well suited to study infants, we were able to gain new insights into the early development of action cognition and its brain basis, providing evidence for an early specialization of the brain processes engaged during action observation.
696077 supplementary material.zip
Download Zip (13.2 MB)Acknowledgments
This work was supported in part by a Sir Henry Wellcome Fellowship awarded to Tobias Grossmann by the Wellcome Trust (082659/Z/07/Z). The work was conducted at the Max Planck Institute for Human Cognitive and Brain Sciences.
REFERENCES
- Allison , T. , Puce , A. and McCarthy , G. 2000 . Social perception from visual cues: Role of the STS region . Trends in Cognitive Sciences , 4 : 267 – 278 .
- Avenanti , A. , Sirigu , A. and Aglioti , S. M. 2010 . Racial bias reduces empathic sensorimotor resonance with other-race pain . Current Biology , 20 : 1018 – 1022 .
- Aziz-Zadeh , L. , Maeda , F. , Zaidel , E. , Mazziotta , J. and Iacoboni , M. 2002 . Lateralization in motor facilitation during action observation: A TMS study . Experimental Brain Research , 144 : 127 – 131 .
- Bertenthal , B. I. , Proffitt , D. R. and Kramer , S. J. 1987 . Perception of biomechanical motions by infants - Implementation of various processing constraints . Journal of Experimental Psychology – Human Perception and Performance , 13 : 577 – 585 .
- Blake , R. and Shiffrar , M. 2007 . Perception of human motion . Annual Reviews in Psychology , 58 : 47 – 73 .
- Buccino , G. , Lui , F. , Canessa , N. , Patteri , I. , Lagravinese , G. , Benuzzi , F. … and Rizzolatti , G. 2004 . Neural circuits involved in the recognition of actions performed by nonconspecifics: An fMRI study . Journal of Cognitive Neuroscience , 16 : 114 – 126 .
- Cross , E. S. , Hamilton , A. F. and Grafton , S. T. 2006 . Building a simulation de novo: Observation of dance by dancers . NeuroImage , 31 : 1257 – 1267 .
- Cross , E. S. , Liepelt , R. , Hamilton , A. F. , Parkinson , J. , Ramsey , R. , Stadler , W. Prinz , W. 2011 . Robotic actions preferentially engage the human action observation network . Human Brain Mapping , doi: 10.1002/hbm.21361
- Decety , J. and Somerville , J. A. 2003 . Shared representations between self and other. A social cognitive neuroscience view . Trends in Cognitive Sciences , 7 : 527 – 533 .
- Gallese , V. , Rochat , M. , Cossu , G. and Sinigaglia , C. 2009 . Motor cognition and its role in phylogeny and ontogeny of action understanding . Developmental Psychology , 45 : 103 – 113 .
- Gazzola , V. , Rizzolatti , G. , Wicker , B. and Keysers , C. 2007 . The anthropomorphic brain: The mirror neuron system responds to human and robotic actions . NeuroImage , 35 : 1674 – 1684 .
- Gervain , J. , Macagno , F. , Cogoi , S. , Pena , M. and Mehler , J. 2008 . The neonate brain detects speech structure . Proceedings of the National Academy of Sciences of the United States of America , 105 : 14222 – 14227 .
- Grèzes , J. and Decety , J. 2001 . Functional anatomy of execution, mental simulation, observation, and verb generation of actions: A meta-analysis . Human Brain Mapping , 12 : 1 – 19 .
- Grossmann , T. and Johnson , M. H. 2010 . Selective prefrontal cortex responses to joint attention in early infancy . Biology Letters , 6 : 540 – 543 .
- Grossmann , T. , Johnson , M. H. , Lloyd-Fox , S. , Blasi , A. , Deligianni , F. , Elwell , C. and Csibra , G. 2008 . Early cortical specialization for face-to-face communication in human infants . Proceedings of the Royal Society B: Biological Sciences , 275 : 2803 – 2811 .
- Grossmann , T. , Parise , E. and Friederici , A. D. 2010 . The detection of communicative signals directed at the self in infant prefrontal cortex . Frontiers in Human Neuroscience , 4 : 201
- Jeannerod , M. 2001 . Neural simulation of action: A unifying mechanism for motor cognition . NeuroImage , 14 : S103 – S109 .
- Jeannerod , M. 2006 . Motor cognition: What actions tell the self , Oxford , , UK : Oxford University Press .
- Keysers , C. and Gazzola , V. 2007 . Integrating simulation and theory of mind: From self to social cognition . Trends in Cognitive Sciences , 11 : 194 – 196 .
- Kilner , J. M. , Friston , K. J. and Frith , C. D. 2007a . Predictive coding: An account of the mirror neuron system . Cognitive Processing , 8 : 159 – 166 .
- Kilner , J. M. , Friston , K. J. and Frith , C. D. 2007b . The mirror neuron system: A Bayesian perspective . NeuroReport , 16 : 619 – 623 .
- Koch , S. P. , Steinbrink , J. , Villringer , A. and Obrig , H. 2006 . Synchronization between background activity and visually evoked potential is not mirrored by focal hyperoxygenation: Implications for the interpretation of vascular brain imaging . Journal of neuroscience , 26 : 4940 – 4948 .
- Kourtzi , Z. , Krekelberg , B. and van Wezel , R. 2008 . Linking form and motion in the primate brain . Trends in Cognitive Sciences , 12 : 230 – 236 .
- Lloyd-Fox , S. , Blasi , A. and Elwell , C. E. 2010 . Illuminating the developing brain: The past, present and future of functional near infrared spectroscopy . Neuroscience & Biobehavioral Reviews , 34 : 269 – 284 .
- Lloyd-Fox , S. , Blasi , A. , Everdell , N. , Elwell , C. E. and Johnson , M. H. 2011 . Selective cortical mapping of biological motion processing in young infants . Journal of Cognitive Neuroscience , 23 : 2521 – 2532 .
- Lloyd-Fox , S. , Blasi , A. , Volein , A. , Everdell , N. , Elwell , C. E. and Johnson , M. H. 2009 . Social perception in infancy: A near infrared spectroscopy study . Child Development , 80 : 986 – 999 .
- Marshall , P. J. and Meltzoff , A. N. 2011 . Neural mirroring systems: Exploring the EEG mu rhythm in human infancy . Developmental Cognitive Neuroscience , 1 : 110 – 123 .
- Obrig , H. and Villringer , A. 2003 . Beyond the visible—Imaging the human brain with light . Journal of Cerebral Blood Flow and Metabolism , 23 : 1 – 18 .
- Pena , M. , Maki , A. , Kovacic , D. , Dehaene-Lambertz , G. , Koizumi , H. , Bouquet , F. Mehler , J. 2003 . Sounds and silence: An optical topography study of language recognition at birth . Proceedings of the National Academy of Sciences of the United States of America , 100 : 11702 – 11705 .
- Pinto , J. 1994 . Human infants' sensitivity to biological motion in pointlight cats . Infant Behavior and Development , 17 : 871
- Prinz , W. 1990 . “ A common coding approach to perception and action ” . In Relationships between perception and action , Edited by: Neumann , O. and Prinz , W. 167 – 201 . Berlin : Springer .
- Pulvermueller , F. 2005 . Brain mechanisms linking language and action . Nature Reviews Neuroscience , 6 : 576 – 582 .
- Ramsey , R. and Hamilton , A. F. C. 2010 . Triangles have goals too: Understanding action representation in the left aIPS . Neuropsychologia , 48 : 2773 – 2776 .
- Rizzolatti , G. and Craighero , L. 2004 . The mirror-neuron system . Annual Reviews in Psychology , 27 : 169 – 192 .
- Rizzolatti , G. and Sinigaglia , C. 2010 . The functional role of the parieto-frontal mirror circuit: Interpretations and misinterpretations . Nature Reviews Neuroscience , 11 : 264 – 274 .
- Schubotz , R. I. 2007 . Prediction of external events with our motor system: Towards a new framework . Trends in Cognitive Sciences , 11 : 211 – 218 .
- Shimada , S. and Hiraki , K. 2006 . Infants' brain responses to live and televised action . NeuroImage , 32 : 930 – 939 .
- Simion , F. , Regolin , L. and Bulf , H. 2008 . A predisposition for biological motion in the newborn baby . Proceedings of the National Academy of Sciences of the United States of America , 105 : 809 – 813 .
- Southgate , V. , Johnson , M. H. , El Karoui , I. and Csibra , G. 2010 . Motor system activation reveals infants' online prediction of others' goals . Psychological Science , 21 : 355 – 359 .
- Southgate , V. , Johnson , M. H. , Osborne , T. and Csibra , G. 2009 . Predictive motor activation during action observation in human infants . Biology Letters , 5 : 769 – 772 .
- Stapel , J. C. , Hunnius , S. , van Elk , M. and Bekkering , H. 2010 . Motor activation during observation of unusual versus ordinary actions in infancy . Social Neuroscience , 5 : 451 – 460 .
- Strangman , G. , Culver , J. P. , Thompson , J. H. and Boas , D. A. 2002 . A quantitative comparison of simultaneous BOLD fMRI and NIRS recordings during functional brain activation . NeuroImage , 17 : 719 – 731 .
- Tai , Y. F. , Scherfler , C. , Brooks , D. J. , Sawamoto , N. and Castiello , U. 2004 . The human premotor cortex is ‘mirror’ only for biological actions . Current Biology , 14 : 117 – 120 .
- Vaina , L. M. , Solomon , J. , Chowdhury , S. , Sinha , P. and Belliveau , J. W. 2001 . Functional neuroanatomy of biological motion perception in humans . Proceedings of the National Academy of Sciences of the United States of America , 98 : 11656
- van Elk , M. , van Schie , H. T. , Hunnius , S. , Vesper , C. and Bekkering , H. 2008 . You'll never crawl alone: Neurophysiological evidence for motor resonance in infancy . NeuroImage , 43 : 808 – 814 .
- Wartenburger , I. , Steinbrink , J. , Telkemeyer , S. , Friedrich , M. , Friederici , A. D. and Obrig , H. 2007 . The processing of prosody: Evidence of interhemispheric specialization at the age of four . NeuroImage , 34 : 416 – 425 .