ABSTRACT
The very early perceptional processes that underlie social categorization can be detected with event-related brain potentials (ERPs). Using this methodology, the present work aims to detect differential attentional processing of ingroup and outgroup members based on gender categories. Specifically, three EEG studies tested how factors that enhance social identity relevance, namely gender identification and contextual salience of gender representation, moderate neural gender categorization effects. Study 1 showed that both women (Study 1a) and men (Study 1b) were more likely to show preferential attention to ingroup over outgroup members, but only when they identified strongly with their gender group. Study 2 showed that when gender categories in an intergroup leadership context were made salient (i.e., when women were numerically underrepresented versus equally represented compared to men), women, irrespective of their level of gender identification, showed preferential attention to ingroup over outgroup members. Together, this work provides empirical evidence for (1) the neural gender categorization effect among both men and women as soon as 100 ms after face perception and (2) the moderating role of factors that enhance social identity relevance in early gender categorization.
“We don’t see things as they are. We see things as we are” – Anaïs Nin
People navigate through a complex social world. One of the mechanisms by which people make sense of their social environment is by categorizing themselves and others into groups to which they do (ingroups) and do not belong (outgroups; Turner, Hogg, Oakes, Reicher, & Wetherell, Citation1987). Categorizations can be based on, for example, gender, nationality, and religion and form the basis for a variety of intergroup processes like intergroup bias, conflict, and intragroup helping (Tajfel & Turner, Citation1986).
In the current research, we investigate the early perceptional processes that underlie social categorization, by using event-related brain potentials (ERPs) to detect differential attentional processing of ingroup and outgroup members based on gender (i.e., the categories “women” and “men”). Prior research has extensively shown neural categorization effects in ERPs for ethnic groups (Derks, Stedehouder, & Ito, Citation2015; Dickter & Bartholow, Citation2010; He, Johnson, Dovidio, & McCarthy, Citation2009; Ito & Senholzi, Citation2013; Ito & Tomelleri, Citation2017; Kubota & Ito, Citation2007, Citation2017; Volpert-Esmond, Merkle, & Bartholow, Citation2017; Willadsen-Jensen & Ito, Citation2006, Citation2008, Citation2015). However, the knowledge base for neural categorization effects in ERPs for gender groups is much smaller. Our aim is to contribute to this knowledge base and determine whether and how empirical findings on neural categorization effects for ethnic groups are generalizable to gender groups.
In doing so, we take a social identity perspective (Tajfel & Turner, Citation1986) to theoretically understand how ingroup/outgroup relations affect initial neural responses to other people’s gender, and to empirically establish whether the chronic or contextually induced relevance of a social identity (in this case, the relevance of one’s gender identity as either a man or a woman) influences these attentional processes at early stages of social perception among gender categories. We first focus on a chronic, individual-level factor determining gender identity relevance, namely individual differences in ingroup gender identification. Secondly, we focus on a contextual factor to experimentally induce the relevance of gender identity, namely the salience of underrepresentation of one’s gender in a particular context.
Measuring social categorization with ERPs
ERPs reflect the neural activity associated with various information processing operations and allow us to detect social categorization within the first 300 ms of social perception (e.g., Dickter & Bartholow, Citation2007; Ito & Bartholow, Citation2009). Research established that multiple ERP components are associated with selective attention, each reflecting a different cognitive process (Dickter & Bartholow, Citation2007). The N100 component is a negative frontocentral deflection that occurs around 100 ms after stimulus onset. The P200 is a positive parietal deflection that occurs around 200 ms after stimulus onset. Both components are often interpreted as the orientation toward novel, threatening and distinctive stimuli (e.g., Ito & Bartholow, Citation2009). The anterior N200 is a negative frontocentral deflection that occurs around 250 ms after stimulus onset, and is often interpreted as selective attention to and encoding of individuating information, and deeper levels of attention (Ito & Bartholow, Citation2009; Kubota & Ito, Citation2007; Senholzi & Kubota, Citation2016).
Thus far, empirical evidence for the sensitivity of the P200 and N200 to early attention in social categorization processes has consistently been found for ethnic and racial groups (e.g., Derks et al., Citation2015; Dickter & Bartholow, Citation2007; Ito & Urland, Citation2003). Neural sensitivity to racial categories has also been observed at an even earlier component, namely the N100 (122 ms after stimulus onset), with White participants showing larger amplitudes in response to Black than to White targets (Ito & Urland, Citation2003; 110 ms; Ma et al., Citation2009). These findings show that individuals are able to make a differentiation between racial ingroup and outgroup faces as soon as 100 ms after visual presentation.
Based on the growing body of empirical evidence for racial neural categorization effects described above, the N100, P200, and N200 ERP components are proven to be relevant for early social categorization. Building on this, in the current research we focus on these three ERP components and examine their relevance for neural categorization effects based on gender groups.
A social identity perspective on social categorization with ERPs
Most ERP research on social categorization was built on the assumption that social categorization is an automatic response triggered by social targets. In other words, social categorization was understood as an automated input variable that causes other more downstream effects in perceivers’ attitudes, perceptions, and behaviors. In that sense, an ERP response should only depend on characteristics of the target stimuli that perceivers are confronted with, and not on characteristics of the perceivers themselves (Ito, Citation2013). More recently, however, a complementary view on social categorization emerged that proposes that early social categorizations are more dynamic and can be driven by people’s (social identity) motives or other perceiver characteristics (Blair, Citation2002).
Empirical evidence on ERP research focusing on racial and ethnic categorizations supports this latter view. Specifically, in terms of ingroup/outgroup relations, a sensitivity to racial groups has repeatedly been found with larger N100s and P200s to outgroup than to ingroup targets, and larger N200s to ingroup than to outgroup targets (Dickter & Bartholow, Citation2007; Ito & Bartholow, Citation2009; Ito & Urland, Citation2003, Citation2005; Kubota & Ito, Citation2007, Citation2017; Willadsen-Jensen & Ito, Citation2006, Citation2008). Importantly, this differential processing not only depends on the group membership of the target but also on the group membership of the perceiver. For example, Dickter and Bartholow (Citation2007) found that Black participants (i.e., perceivers) show larger P200s in response to White than to Black target faces and larger N200s in response to Black than to White target faces, while White participants showed the exact opposite pattern in response to Black versus White target faces. In other words, the research by Dickter and Bartholow (Citation2007) demonstrates that ingroup/outgroup differentiation in neural ERP responses depends on people’s own social identities, such that it depended on perceivers’ own racial group membership (i.e., being Black or White) how they processed either White or Black target faces.
Building on this work, we rely on social identity theory (SIT; Tajfel & Turner, Citation1986) to argue that ERP responses in early gender categorization not only depend on mere group membership, but are also contingent upon how meaningful social identities are, in terms of the relevance of the perceivers’ gender identity. Specifically, we aim to understand for whom (individual differences) and when (social context) early social categorization responses along gender lines are more likely to occur.
Social identity relevance moderates ERP responses in early social categorization
SIT posits that group memberships (e.g., being a man or a woman) give meaning to our self-concepts and, at least in part, determine how we respond to our social environment. People for whom a particular group membership is highly important and self-relevant (i.e., high ingroup identification), tend to feel more connected and committed to their ingroup (Ellemers, Spears, & Doosje, Citation1997), and are more willing to protect it (Ellemers, Spears, & Doosje, Citation2002). By contrast, people for whom a particular group membership is less important (i.e., low ingroup identification), are less concerned with their ingroup and are less inclined to categorize themselves and others based on it.
SIT further posits that people are driven to maintain a positive image of their group memberships (Tajfel & Turner, Citation1986). This goal is attained when the ingroup is evaluated more positively (i.e., has a higher status) than other relevant outgroups. If this positive ingroup status is undermined (e.g., due to negative group stereotyping or underrepresentation), it leads to social identity threat. This, in turn, triggers coping responses such as discrimination toward outgroups (Branscombe & Wann, Citation1994) and efforts to improve ingroup status (Ouwerkerk, De Gilder, & De Vries, Citation2000).
The notion that the relevance of social identity, both on an individual level and on a contextual level, affects neural social categorization was recently supported empirically based on ERP responses to ethnic group categories (Derks et al., Citation2015). In their Study 1, Derks et al. (Citation2015) showed that differential ERP responses in relation to ethnic ingroup and outgroup target faces were moderated by individual differences in ethnic identification. Specifically, non-Muslim participants showed larger N200s in response to non-Muslim (ingroup) than to Muslim (outgroup) faces, only when they identified strongly with their ethnic ingroup. Thus, self-relevance of one’s own ethnic identity affects early neural differentiation between ethnic ingroup and outgroup targets. Furthermore, in Study 2, Derks et al. (Citation2015) showed that an experimental manipulation of social identity threat (versus control) resulted in differential ERP responses to ethnic categories, such that Muslim participants showed larger N200s to non-Muslim (outgroup) than to Muslim (ingroup) faces, but only when they were asked to think of an incident in which they had experienced discrimination or disrespect due to their religion (i.e., high social identity threat). Together, these findings provide first empirical evidence for the moderating role of (individual or contextual) social identity relevance for perceivers’ ERP responses to ingroup and outgroup target stimuli.
Neural categorization of gender
Compared to racial and ethnic categories, the evidence-base for neural categorizations based on gender is much smaller. Particularly the study of social identity relevance in ERP responses to gender categories is new. The question is whether the findings for ethnic categories discussed above (Derks et al., Citation2015) can be extrapolated to gender categories as well. On the one hand, one could argue that, since gender and racial categories are both highly physically and visually distinct (Stangor, Lynch, Duan, & Glass, Citation1992; Taylor, Fiske, Etcoff, & Ruderman, Citation1978) comparable ERP responses can be expected. On the other hand, gender categories differ in important ways from racial and ethnic categories, for example, in terms of intergroup dynamics (e.g., mutual interdependence, physical proximity, romantic/reproductive function). Also, people’s experience with outgroup members is much higher when it comes to gender outgroups than ethnic outgroups (Ito & Urland, Citation2003); men and women have a lot of contact that is often positive in nature. Thus, one could also argue that there may be differences in the ERP responses between gender and racial categories, and the degree to which they are moderated by group membership.
Thus far, the empirical research on ERP responses in gender categorizations served different purposes than establishing ingroup/outgroup effects. For example, research focused on the effect of frequent versus infrequent exposure to male and female target faces (visual mismatch detection; Kecskés-Kovács, Sulykos, & Czigler, Citation2013), the facial structural encoding of male and female target faces (Mouchetant-Rostaing, Giard, Bentin, Aguera, & Pernier, Citation2000), sexual facial morphing (Freeman, Ambady, & Holcomb, Citation2010), sex differences in the face encoding of perceptual – lower level – and social – higher level – features (Sun, Gao, & Han, Citation2010), attractive versus unattractive opposite-gender faces only (Van Hooff, Crawford, & Van Vugt, Citation2011) and neural categorization of multiple social categories at the same time, for example, the intersection between gender and age (young/old) categories (Mouchetant-Rostaing & Giard, Citation2003) or gender and ethnicity (Dickter & Bartholow, Citation2007; Ito & Urland, Citation2003).
Importantly, the majority of studies on ERP responses to gender categories did not include participant gender (i.e., group membership of the perceiver) as part of the research paradigm, likely because this was not part of their research goal, and/or because the sample size was too small for the research design to allow for an (additional) empirical test of the effects of gender of the perceiver. When only varying the gender of target stimuli without taking perceiver’s own gender identity into account, it is not possible to establish the presence of ingroup/outgroup effects. Therefore, while gender has proven to be relevant in ERP research on neural categorization, from the earlier work we cannot yet determine whether differential ERP responses are due to the fact that participants are presented with two different target categories or because people process ingroup information differently than outgroup information.
Two ERP studies that did take participant gender into account both showed differentiation between male and female targets on the P200 and N200, however with mixed results (Dickter & Bartholow, Citation2007; Ito & Urland, Citation2003). Ito and Urland (Citation2003) found for both male and female perceivers, that male targets elicited larger P200 amplitudes than female targets, and female targets elicited larger N200 amplitudes than male targets. However, Dickter and Bartholow (Citation2007) found the opposite pattern; for both male and female perceivers, female targets elicited larger P200 amplitudes than male targets, and male targets elicited larger N200 amplitudes than female targets. In both studies, neural categorization effects on target gender were not affected by perceiver gender.
All in all, this means that despite the increasing number of theoretical and experimental contributions to the study of ERP responses in gender categorization, there is as of yet no unambiguous evidence that neural gender categorization is determined by group membership of the perceiver per se. Moreover, a social identity perspective on neural gender categorization, and particularly an investigation of the relevance of gender identity of the perceiver at both the individual and contextual level is to our knowledge – absent in the current empirical research.
Adding to the current knowledge base, in this research, we aim to gain insight into whether individuals process gender ingroup information differently than gender outgroup information. We expect that differential attentional processing of gender categories in early ERP responses is contingent upon self-relevance of ingroup membership. Specifically, the degree to which perceivers categorize between male and female targets in their early perception depends on the level of psychological importance people attribute to their gender identity, or gender identification, and the relevance that this gender identity has within the context.
Therefore, in the first step, we will focus on spontaneous gender categorization of both male and female perceivers in response to both ingroup and outgroup faces. We expect men/women (respectively) to process ingroup (male/female) target faces differently than outgroup (female/male) target faces, especially among those for whom gender identification is high. In our argumentation, we rely on the previous findings on social identity relevance in ERP responses (Derks et al., Citation2015) to test whether for gender groups too, early attentional social categorization processes are predicted by individual differences in gender identification. We expect that, among both women and men, high identifiers differentiate more strongly between ingroup and outgroup members than low identifiers, resulting in differences in amplitudes on the N100, P200, and N200 in response to ingroup and outgroup targets (Study 1; Hypothesis 1).
Secondly, we expect that when gender identities become highly contextually salient or relevant, this also results in stronger differentiation between ingroup and outgroup targets in ERP responses. Here we focus specifically on women in a situation in which their gender identity is potentially threatened, for example, because they find themselves in a context in which women are underrepresented or devalued relative to men (i.e., low relative ingroup status). Prior work in the social identity tradition already demonstrated that contextual cues that signal the low-status position of women relative to men indeed result in stronger ingroup/outgroup differentiation (e.g., Kaiser & Spalding, Citation2015). In translating these outcomes to the detection of early neural responses to gender categories, we hypothesize that women differentiate more strongly between ingroup (i.e., women) and outgroup (i.e., men) members when they are confronted with low status and underrepresentation in a leadership context, thus resulting in differences in amplitudes on the N100, P200, and N200 in response to ingroup and outgroup targets (Study 2; Hypothesis 2).
Overview
We performed three studies to investigate how individual differences in gender identification (Study 1a and b) and contextual variations in gender representation (Study 2) predict spontaneous gender categorization at early stages of information processing (ERPs). We measured gender identification among women (Study 1a; N = 28) and men (Study 1b; N= 27) and recorded continuous electroencephalograms (EEG) while participants performed an evaluative priming task in which they were presented with faces of male and female targets (Fazio, Jackson, Dunton, & Williams, Citation1995). In Study 2 (N= 80) we also tested whether neural gender categorizations are moderated by contextual gender representation. Specifically, we experimentally manipulated an intergroup context where female participants were awarded a leadership position that was typically only given to men (i.e., underrepresentation) or as often to men as to women (i.e., equal representation).
All participants were recruited from a Dutch university and received course credits or monetary payment in return for their efforts. All studies were conducted with the approval of the Ethics Committee of the Faculty of Social and Behavioral Sciences of Utrecht University filed under number FETC18-117 (Derks).
Study 1
Study 1a and b had a similar study design, but with a female (Study 1a) and a male (Study 1b) sample. Both studies were treated as separate because they were conducted in two consecutive years, by different lab assistants and in different labs, which theoretically can lead to confounds in interpretation of effects. Moreover, we did not hypothesize differential effects for male and female participants, in the sense that we did not expect to find a Participant Gender X Target Gender X Gender Identification effect. Therefore, collapsing the two studies was also not necessary from an empirical viewpointFootnote1
Method
Participants and design
Both studies had a 1 (between: gender identification, standardized, continuous) x 2 (within: target gender, male/female) x 6 (within: electrode) design. Power analyses based on an alpha of 0.5, power of 0.80, and an expected effect size of 0.24 on the hypothesized two-way interaction between gender identification and target gender (based on earlier ERP categorization research by Derks et al., Citation2015, Study, p. 1) resulted in a required sample size of N = 24. The data of 28 female (Study 1a; Mage = 21.71, SD = 1.24)Footnote2 and 27 male (Study 1b; Mage = 22.19, SD = 1.92)Footnote3 students were included for analyses.
Procedure
After explaining the general procedure and obtaining consent according to the Declaration of Helsinki (Citation1991; p. 1194), EEG electrodes were applied. Participants then filled in a paper-and-pencil questionnaire, supposedly for a separate study, measuring their student (filler) and gender identification using nine items on 7-point Likert scales (1 = strongly disagree; 7 = strongly agree), e.g., “Being a student/woman/man is important to me” and “I identify with other students/women/men” (Cameron, Citation2004; Doosje, Ellemers, & Spears, Citation1995; Ellemers, Kortekaas, & Ouwerkerk, Citation1999). The scale reliability was high (female sample: α = .90; M = 5.44, SD = 0.81; male sample: α = .91, M = 5.34, SD = 0.88).
Subsequently, the second study was introduced as examining the automaticity of word comprehension and face recognition in which participants performed an evaluative priming task (Fazio et al., Citation1995) adapted to the context of gender categorization and gender role stereotypes. Specifically, the three-phase task measured automatic activation of evaluations when career and family words were primed with female and male facesFootnote4 In phase 1 (32 trials), participants categorized unprimed words as career (8; e.g., business) or family (8; e.g., home). All words were presented twice in random order. In phase 2 (96 trials), participants categorized the same career and family words, but now each word was preceded by a male or female target face (prime). Pictures from eight Caucasian male and eight Caucasian female target faces, matched on attractiveness and with a neutral facial expression, were taken from the Radboud Faces Database (Langner et al., Citation2010). Pictures were equal in background, size, and grayscale. Each combination of face (female/male) and word (family/career) was presented three times, in random order. Each trial started with a fixation screen displaying three asterisks (1000 ms), followed by a female or male target face (315 ms; measuring stimulus-locked ERPs in response to the faces), a blank screen (135 ms) followed by a career or family word (presented until the participant responded; maximum 1750 ms). Phase 3 merely served to support the cover story (i.e., face recognition). Participants were presented with 16 faces from phase 2 and 14 new faces (taken from the Radboud Faces Database) and were asked to indicate whether they had seen the faces before.
Finally, the EEG electrodes were removed and participants were debriefed, thanked and rewarded for participation.
EEG recording
ERPs were derived from continuous EEG signals recorded simultaneously from 18 scalp sites (F3, F7, Fz, F4, F8, C3, Cz, C4, P3, P7, Pz, P4, P8, O1, Oz, O2, T7, T8), using the ActiveTwo BioSemi system (BioSemi, Amsterdam, the Netherlands) and electrodes placed into an ECI electrocap. Four additional electrodes were placed next to the right eye and next, above and below the left eye to monitor eye blinking and vertical and horizontal eye movements. Two additional electrodes were placed over the left and right mastoids. During EEG-recording, the ground electrodes were formed by the Common Mode Sense active electrode and the Driven Right Leg passive electrode. EEG activity was sampled at 2048 Hz using ActiView software (BioSemi), and processed and analyzed using Brain Vision Analyzer software (Brain Products). Offline, data were referenced to a computed average of the left and right mastoids, band-pass filtered with a high-pass filter of 0.1 Hz and a low-pass filter of 30 Hz, and corrected for ocular artifacts (Gratton, Coles, & Donchin, Citation1983). Data were segmented into epochs from 200 ms before stimulus onset to 450 ms after stimulus onset. Separate ERP averages were computed for the male and female target faces. Epochs exceeding a 100 µV amplitude difference, a voltage step difference of 50 μV between sample points or with activity smaller than 0.5 µV were excluded from these averages. The resulting averages were baseline corrected for the 200 ms before stimulus onset ( and for the female and male samples, respectively).
Figure 1. ERP grand average waveforms at frontal (F3, Fz, F4), central (C3, Cz, C4), and parietal (P3, Pz, P4) electrodes for male (black line) and female (gray line) target faces (Study 1a: female participants).
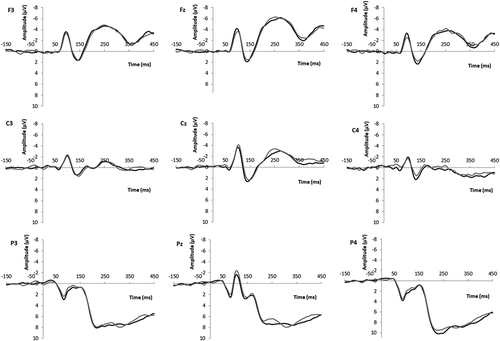
Figure 2. ERP grand average waveforms at frontal (F3, Fz, F4), central (C3, Cz, C4), and parietal (P3, Pz, P4) electrodes for male (black line) and female (gray line) target faces (Study 1b: male participants).
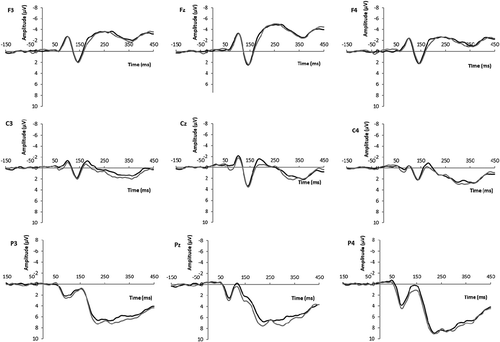
The N100 was quantified as the mean amplitude within the 80–120 ms time frame at the electrodes F3, Fz, F4, C3, Cz, and C4. The P200 was quantified as the mean amplitude within the 120–170 ms time frame at the electrodes C3, Cz, C4, P3, Pz, and P4. Finally, the N200 was quantified as the mean amplitude within the 200–320 ms time frame at the electrodes F3, Fz, F4, C3, Cz, and C4.
Mean amplitudes were analyzed using Repeated Measures General Linear Model (GLM) with between-subjects variable gender identification (standardized, continuous), and within-subjects factors target gender (male/female) and electrode.
Results
ERP data
N100
Among female respondents (Study 1a), analyses showed no main effect of target gender. Importantly, in support of Hypothesis 1, analyses showed a significant Gender Identification X Target Gender effect, F(1,26) = 14.14, p = .001, partial η2 = .35. Simple slope analysis for high (+1 SD) and low (−1 SD) identifiers separately (Aiken & West, Citation1991; Preacher, Curran, & Bauer, Citation2006) demonstrated that women with a relatively high gender identification showed preferential attention to ingroup (i.e., female; M = −2.31, SE = 0.49) over outgroup (i.e., male; M = −1.58, SE = 0.47) faces, F(1,26) = 6.48, p= .017, partial η2 = .20, while women with a relatively low gender identification showed preferential attention to outgroup (i.e., male; M= −2.41, SD= 0.47) over ingroup (i.e., female; M = −1.60, SD= 0.49) faces, F(1,26) = 7.96, p = .009, partial η2 = .23 (). Analyses per target gender (female/male) showed no significant main effect of gender identification for both target groups (p = .323/.234, respectively). Analyses also showed a significant main effect of electrode, F(5,22) = 10.65, p < .001, partial η2 = .71, with the largest N100s on Fz and Cz.
Figure 3. Mean amplitude responses (average F3, Fz, F4, C3, Cz, and C4 electrodes) on the N100 component to male and female target faces for female respondents with a relatively low (−1 SD) and high (+1 SD) gender identification (Study 1a). More negative numbers indicating higher amplitudes. Error bars represent standard errors.
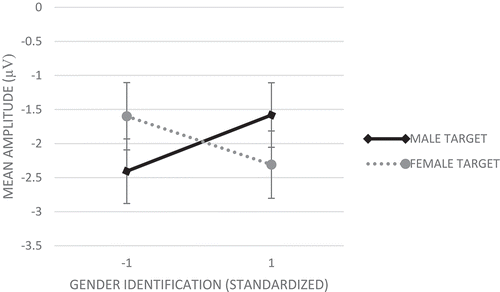
Similarly, among male respondents (Study 1b), analyses showed no main effect of target gender. Importantly, in support of Hypothesis 1, analyses showed a Gender Identification X Target Gender effect, F(1,25) = 9.00, p = .006, partial η2 = .27. Similar to Study 1a, simple slope analysis () demonstrated that men with a relatively high gender identification showed preferential attention to ingroup (i.e., male; M = −1.32, SE = 0.33) over outgroup (i.e., female; M = −0.62, SE = 0.35) faces, F(1,25) = 8.69, p= .007, partial η2 = .26. However, men with a relatively low gender identification showed no preferential attention for either category, F(1,25) = 1.78, p= .194, partial η2 = .07. Analyses per target gender showed a significant gender identification effect on female targets, F(1,25) = 5.57, p = .026, partial η2 = .18, but not on male targets, F(1,25) = 0.14, p = .711, partial η2 = .01. Analyses also showed a significant main effect of electrode, F(5,21) = 17.31, p < .001, partial η2 = .81, with the largest N100s on Fz.
Figure 4. Mean amplitude responses (average F3, Fz, F4, C3, Cz, and C4 electrodes) on the N100 component to male and female target faces for male respondents with a relatively low (−1 SD) and high (+1 SD) gender identification (Study 1b). More negative numbers indicating higher amplitudes. Error bars represent standard errors.
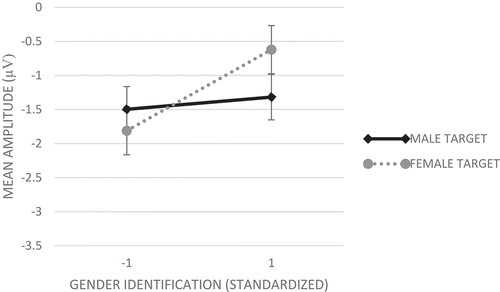
P200
Among female respondents, analyses showed no significant effects of gender identification, target gender or their interaction on P200 amplitudes.
Among male respondents, analyses showed a main effect of target gender, F(1,24) = 4.88, p = .037, η2 = .17 (larger amplitudes to female [outgroup] targets than to male [ingroup] targets, Mfemale = 1.54, SD = 0.39; Mmale = 1.13, SD = 0.44). No other significant effects of gender identification, target gender or their interaction on P200 amplitudes.
N200
Among both female and male respondents, analyses showed no significant effects of gender identification, target gender or their interaction on N200 amplitudes.
Discussion
We found a main effect of target gender for male respondents, but only on the P200. In line with research on ethnic groups, men showed preferential attention to outgroup over ingroup members. While no direct evidence could be obtained that in general, women and men differentiate between ingroup and outgroup target faces on the N100 and N200 components, we found that this neural categorization effect was conditional upon gender identification (Hypothesis 1) among both female (Study 1a) and male (Study 1b) respondents. Specifically, on the N100, we found that both women and men with a relatively high gender identification spontaneously showed preferential attention to ingroup over outgroup members, while women with a relatively low gender identification spontaneously showed preferential attention to outgroup over ingroup members and men with a relatively low gender identification did not show preferential attention to either group. Thus, those who identify highly with their gender identity as well as women who identify low with their gender identity showed differential spontaneous neural categorization between ingroup and outgroup members at this early stage of information processing. These findings provide empirical support that differential neural responses in gender categorizations are contingent upon individual differences in social identity relevance (i.e., gender identification).
In a second step, Study 2 was set out to investigate a contextual factor inducing social identity relevance, namely the numerical representation of the ingroup category, in addition to individual differences in social identity relevance (i.e., gender identification).
Study 2
When social categories are salient, people become more prone to how they as group members are different from other groups (Wilder, Citation1984). Being dissimilar from others can be especially unpleasant when the salient social category is associated with negative stereotypes or low status (Van Veelen, Derks, & Endedijk, Citation2019; Veldman, Meeussen, Van Laar, & Phalet, Citation2017). In this study, we, therefore, focus specifically on female participants and manipulated gender identity salience based on numerical underrepresentation (versus equal representation) of women in leadership, a role that is stereotypically perceived as more fitting with men, and hence, also more often occupied by men (Eagly & Karau, Citation2002; Eagly, Makhijani, & Klonsky, Citation1992; Heilman, Citation2001; Schyns & Schilling, Citation2011).
Method
Participants and design
The study had a 2 (between: gender representation equal/under) x 1 (between: gender identification, standardized, continuous) x 2 (within: target gender, male/female) x 6 (within: electrode) design. Power analyses based on an alpha of 0.5, power of 0.80, and an expected effect size of 0.16 on the hypothesized two-way interaction between gender representation and target gender (based on earlier ERP categorization research by Derks et al., Citation2015, Study, p. 2) resulted in a required sample size of N = 72. The data of 80 female students (Mage = 21.25, SD = 2.71) were included for analysesFootnote5 Apart from deviations indicated below, the procedure was identical to Study 1. Student (filler) and gender identification was measured with five items (α = .88; M = 5.27, SD = 0.91).
Procedure
Manipulation of gender representation in leadership
Following Kaiser and Spalding (Citation2015) experimental procedure, participants were told that they would work together on a group task, ostensibly with two other participants. A work profile, including photograph, was made of each participant to send to their workgroup members. Participants were then presented with (bogus) profiles of a male and female workgroup member and were told that one of the three of them would be assigned the role of team manager. Ostensibly to determine who would be given this role, all group members were asked to take a “management aptitude test”, which included a creative thinking task (i.e., “think of as many uses for a tin can”) and a leadership style test (task versus relation oriented based on the “Managerial Grid” of Blake & Mouton, Citation1985). Afterward, each participant was informed that they scored best on the test and that they were therefore selected to be the team manager.
Participants were then randomly assigned to an underrepresentation (n = 41) or equal representation (n = 41) condition. In the underrepresentation condition, participants were given a very oversized t-shirt with “manager” written on the front (giving the clear impression that this shirt was meant to be worn by a man) to wear during the rest of the experiment. Another picture was taken to make participants even more aware of the large t-shirt. They were asked to sign a “Manager Roster”, which ostensibly listed the names of the managers in previous sessions. Ninety percent of the names were male (emphasizing underrepresentation of female participants in the leadership role). In the equal representation condition, participants were given a t-shirt in their own size (S, M, L, XL) with “manager” written on the front to wear during the rest of the experiment. Then another picture was taken, and they were asked to sign a “Manager Roster”, which listed 50% male and 50% female names (Kaiser & Spalding, Citation2015).
Participants then performed phase 1 (16 trials) and 2 (96 trials) of the evaluative priming task (Fazio et al., Citation1995). The words presented were adapted to the context of leadership (leadership [8; e.g., boss] or subordinate position [8; e.g., assistant]).
Manipulation check
Participants were asked to estimate the percentage of male/female names on the manager rosterFootnote6
EEG recording
EEG was recorded, processed and scored in the same way as in Study 1 (see ).
Results
Manipulation check
The manipulation worked as we intended. Compared to the equal representation condition, participants in the underrepresentation condition reported a higher percentage of male names (M = 65.40, SD = 14.86 versus M =46.46, SD = 16.81; t(92) = −5.79, p < .001), and a lower percentage of female names (M = 34.50, SD = 16.24 versus M = 52.15, SD = 18.33; t(92) = 4.95, p < .001).
ERP data
Mean amplitudes were analyzed using Repeated Measures GLM with between-subjects variable gender identification (standardized, continuous) and factor gender representation (equal/under), within-subjects factors target gender (male/female) and electrode. Following our hypotheses, all main effects and two-way interactions were analyzed, as well as the three-way interaction between gender representation, target gender, and gender identification.
N100
Analyses showed no main effect of target gender. Importantly, in support of Hypothesis 2, analyses showed a significant Gender Representation X Target Gender effect, F(1,76) = 7.18, p = .009, η2 = .09, indicating that attention to female and male faces was significantly moderated by experimental condition. As shows, in the underrepresentation condition N100s were significantly larger for ingroup (female; M = −1.81, SE = 0.25) than for outgroup (male; M = −1.21, SE = 0.26) faces, F(1,38) = 9.42, p = .004, partial η2 = .20. An opposite, yet non-significant pattern was detected in the equal representation condition (N100s larger for outgroup [male], M = −1.44, SE = 0.33, than for ingroup [female] targets, M= −1.27, SE = 0.32), F(1,38) = 0.54, p = .469, partial η2 = .01.
Figure 6. Mean amplitude responses (average F3, Fz, F4, C3, Cz, and C4 electrodes) on the N100 component to male and female target faces for female respondents in the equal representation and underrepresentation conditions (Study 2). More negative numbers indicating higher amplitudes. Error bars represent standard errors.
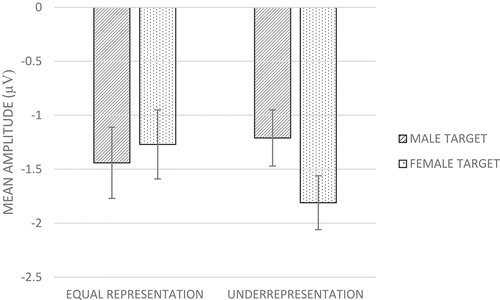
Analyses per target group (female/male) showed no significant main effect of gender representation for both target groups (p = .199/.590, respectively).
The Gender Representation X Target Gender X Gender Identification effect was not significant, F(1,76) = 2.83, p = .097, partial η2 = .04, nor were any other main or interaction effects of gender identification (all p > .097; Hypothesis 2). Analyses did show a significant main effect of electrode, F(5,72) = 23.18, p< .001, partial η2 = .62, with the largest N100s on Fz.
P200
Analyses showed no significant effects of gender identification, target gender, gender representation or their two-way interactions on P200 amplitudes.
N200
Analyses showed a significant main effect of target gender only, F(1,76) = 9.61, p = .003, η2 = .11, with higher N200 amplitudes to ingroup (i.e., female; M = −2.61, SE = 0.33) than to outgroup (i.e., male; M = −2.15, SE = 0.34) faces. Analyses also showed a significant main effect of electrode, F(5,72) = 101.79, p< .001, η2 = .88, with the largest N200s on Fz.
Discussion
We found a main effect of target group, but only on the N200. In line with research on ethnic groups, women showed preferential attention to ingroup over outgroup members. Replicating Study 1, on the N100 we found that neural evidence of gender categorization was qualified by identity relevance (here, contextually induced). Specifically, in support of Hypothesis 2, we found a cross-over interaction, such that women who were underrepresented showed preferential attention to ingroup over outgroup members, while women who were equally represented showed preferential attention to outgroup over ingroup members. No evidence was found for a moderating effect of gender identification in Study 2 (Hypothesis 1).
General discussion
The present research was set out to test whether and how the neural categorization effects found for ethnic groups are generalizable to gender groups and whether factors increasing social identity relevance (e.g., gender identification and contextual gender representation) amplify this process. In three studies we indeed found gender categorization effects on the N100 that are contingent upon factors that enhance social identity relevance – both more chronic individual differences in gender identification and contextually induced increased gender salience stemming from underrepresentation of one’s gender group in a relevant gender context (i.e., leadership).
Specifically, in terms of individual differences in gender identity relevance (Study 1), both women and men were more likely to spontaneously differentiate between ingroup and outgroup and to show preferential attention to ingroup over outgroup members, when they identified relatively high with their gender group. Women, but not men, who identified relatively low with their gender identity showed preferential attention to outgroup over ingroup members. When social identity relevance was contextually induced (Study 2), women, irrespective of their level of gender identification, showed preferential attention to ingroup over outgroup members when they were underrepresented, but not when they were equally represented.
Theoretical implications
These results contribute to the knowledge-base on the neuroscience of social identity in two ways. First, this research further establishes and replicates the finding that social identity relevance (stemming either from strong ingroup identification or contextual salience of social identity; Derks, Citation2013; Derks et al., Citation2015) increases the neural differentiation between ingroup and outgroup members at early stages of information processing. As such, these results provide further evidence for the view that the neural basis for social categorization is a dynamic process, rather than a static input variable (Blair, Citation2002; Dickter & Bartholow, Citation2007). Second, this work replicates and extrapolates previous similar findings in an ethnic context (Derks et al., Citation2015) to a gender context. The current studies are the first to show that early gender categorization, indicated by preferential attention to one’s own gender group over the other, is predicted by factors – both individual-level and contextually induced – that enhance social identity relevance. In particular, such cues of social identity salience unconsciously affect how people perceive their surroundings, that is, in light of their gender identity.
For whom and when does neural differential processing of gender categories occur?
Together these studies emphasize the complex dynamics of the (neural) social categorization process and how it can be driven by perceivers’ social identity motives (e.g., Derks, Citation2013; Derks et al., Citation2015). Gender identification predicted preferential processing of one group over the other when no contextual cues were presented, but not when gender categories were made more salient by contextual cues. Then, preferential processing only depended on the context, suggesting that contextual cues can override the more stable effects of individual differences on neural categorization processes.
These studies do however indicate that chronic, more stable, individual difference variables that induce category salience (i.e., social identification) have larger effects on early social categorization than contextual cues of social category salience (i.e., that make social identities highly relevant). When we compare the size of the effects found in Study 1 and Study 2, the effect size of the interaction effect of the context manipulation in Study 2 was lower than the one for the interaction effect of gender identification in Study 1 (.09 versus .27 and .35). This is in line with the pattern of effect sizes found by previous categorization research on ethnic groups (.16 for context manipulation versus .24 for ethnic identification; Derks et al., Citation2015). We recommend further research to replicate the robustness of these effects for different social categories (e.g., age, gender, race) and different contexts (e.g., work, home, romantic).
In contrast to studies on the intersection between gender and ethnic groups who found differential neural processing on the P200 and N200 (Dickter & Bartholow, Citation2007; Ito & Urland, Citation2003), we found preferential neural processing of gender ingroup over outgroup members as early as on the N100. We also found effects of factors that enhance social identity relevance (i.e., gender identification and gender representation) on this early component. Yet, only in Study 1b, we detected evidence of gender categorization effects on the P200 (i.e., preferential processing of outgroup over ingroup members, in line with studies on ethnic groups), and only in Study 2, we detected evidence of gender categorization effects on the N200 (i.e., preferential processing of ingroup over outgroup members, in line with studies on ethnic groups [Dickter & Bartholow, Citation2007; Ito & Urland, Citation2003]). We furthermore did not detect any evidence of social identity relevance effects on both the P200 and N200.
A possible explanation for the categorization effect on the N100, which until now has been mainly interpreted in terms of greater vigilance to outgroup members (i.e., novelty or threat; e.g., Ito & Bartholow, Citation2009), is that the N100 may have a different function (i.e., interpretation) for processing gender groups. Men and women interact a lot with each other in everyday life and therefore do not necessarily perceive each other so much as novel or threatening. It could be that when our gender identity is important to us, we by default focus on our ingroup. Furthermore, differentiation between gender groups might occur differently than differentiation between racial groups, both in regard to whether preferential processing of one group arises at all, and in terms of its time course. We encourage further research to better understand why differential neural social categorization manifests on particular ERP components (e.g., the time-course; interpretation for social perception), for example, depending on the type of social identity that is activated or relevant (e.g., gender, race, age) and the context in which these neural gender categorizations are observed (e.g., work, home, romantic).
Conclusion
The present studies provide empirical evidence for early gender categorization, indicated by preferential processing of one gender group over the other, to occur as soon as 100 ms after face perception. Factors increasing the relevance of social identity, namely gender identification and contextually induced gender category-salience have a moderating role herein and elicit preferential processing of the ingroup. To conclude, individual differences in, and contextual cues of gender category salience make people look at others through the lens of their gender. Who you are and where you are determine your view of the social world.
Revised_Manuscript_SN_Domen_Supplementary_material.docx
Download MS Word (15.9 KB)Disclosure statement
No potential conflict of interest was reported by the authors.
Supplementary material
The supplementary data for this article can be accessed here.
Additional information
Funding
Notes
1 Nevertheless, if we do run the analyses as if this were one study (i.e., including a participant gender between-subjects factor), results on N100 amplitudes still demonstrate the expected Target Group X Gender Identification effect, F(1,51) = 22.95, p < .001, partial η² = .31, and indeed no Participant Gender X Target Group X Gender Identification effect (i.e., no three-way interaction), F(1,51) = 0.97, p = .330, partial η² = .02..
2 Thirty female students participated in the study, but due to missing data (central electrodes could not be scored), the data of two participants were excluded for analyses.
3 Thirty-three male students participated in the study, but due to missing data (electrodes could not be scored; 2) and left-handedness (4), the data of six participants were excluded for analyses.
4 Implicit intergroup bias was also analyzed (see supplementary material), but not the main focus of this investigation.
5 Ninety-four female students participated but due to recording errors of eye movements (12) and electrodes that could not be scored (2), data of 14 participants were excluded for analyses..
6 Following Kaiser and Spalding (Citation2015) paradigm, we measured other variables afterward which were not the focus of this investigation. An overview can be obtained from the first author upon request..
References
- Aiken, L. S., & West, S. G. (1991). Multiple regression: Testing and interpreting interactions. Newbury Park, CA: Sage.
- Blair, I. V. (2002). The malleability of automatic stereotypes and prejudice. Personality and Social Psychology Review, 6(3), 242–261.
- Blake, R., & Mouton, J. (1985). The managerial grid III: The key to leadership excellence. Houston, TX: Gulf Publishing Corporation.
- Branscombe, N. R., & Wann, D. L. (1994). Collective self-esteem consequences of out-group derogation when a valued social identity is on trial. European Journal of Social Psychology, 24, 641–657.
- Cameron, J. E. (2004). A three-factor model of social identity. Self and Identity, 3(3), 239–262.
- Derks, B. (2013). The implicit effects of social identity: Measuring early social categorization with event-related brain potentials. In B. Derks, D. Scheepers, & N. Ellemers (Eds.), Neuroscience of prejudice and intergroup relations (pp. 45–62). London, UK: Psychology Press.
- Derks, B., Stedehouder, J., & Ito, T. A. (2015). Social identity modifies face perception: An ERP study of social categorization. Social Cognitive and Affective Neuroscience, 10(5), 672–679.
- Dickter, C. L., & Bartholow, B. D. (2007). Racial ingroup and outgroup attention biases revealed by event-related brain potentials. Social Cognitive and Affective Neuroscience, 2(3), 189–198.
- Dickter, C. L., & Bartholow, B. D. (2010). Ingroup categorization and response conflict: Interactive effects of target race, flanker compatibility, and infrequency on N2 amplitude. Psychophysiology, 47, 596–601.
- Doosje, B., Ellemers, N., & Spears, R. (1995). Perceived intragroup variability as a function of group status and identification. Journal of Experimental Social Psychology, 31(5), 410–436.
- Eagly, A. H., & Karau, S. J. (2002). Role congruity theory of prejudice toward female leaders. Psychological Review, 109(3), 573–598.
- Eagly, A. H., Makhijani, M. G., & Klonsky, B. G. (1992). Gender and the evaluation of leaders: A meta-analysis. Psychological Bulletin, 111(1), 3–22.
- Ellemers, N., Kortekaas, P., & Ouwerkerk, J. W. (1999). Self-categorisation, commitment to the group and group self-esteem as related but distinct aspects of social identity. European Journal of Social Psychology, 29, 371–389.
- Ellemers, N., Spears, R., & Doosje, B. (1997). Sticking together or falling apart: In-group identification as a psychological determinant of group commitment versus individual mobility. Journal of Personality and Social Psychology, 72, 617–626.
- Ellemers, N., Spears, R., & Doosje, B. (2002). Self and social identity. Annual Review of Psychology, 53, 161–186.
- Fazio, R. H., Jackson, J. R., Dunton, B. C., & Williams, C. J. (1995). Variability in automatic activation as an unobtrusive measure of racial attitudes: A bona fide pipeline? Journal of Personality and Social Psychology, 69(6), 1013–1027.
- Freeman, J. B., Ambady, N., & Holcomb, P. J. (2010). The face-sensitive N170 encodes social category information. NeuroReport, 21(1), 24–28.
- Gratton, G., Coles, M. G. H., & Donchin, E. (1983). A new method for off-line removal of ocular artifacts. Electroencephalography and Clinical Neurophysiology, 55(4), 468–484.
- He, Y., Johnson, M. K., Dovidio, J. F., & McCarthy, G. (2009). The relation between race-related implicit associations and scalp-recorded neural activity evoked by faces from different races. Social Neuroscience, 4(5), 426–442.
- Heilman, M. E. (2001). Description and prescription: How gender stereotypes prevent women’s ascent up the organizational ladder. Journal of Social Issues, 57(4), 657–674.
- Ito, T. A. (2013). Imaging the pictures in our heads: Using ERPs to inform our understanding of social categorization. In B. Derks, D. Scheepers, & N. Ellemers (Eds.), Neuroscience of prejudice and intergroup relations (pp. 25–44). London, UK: Psychology Press.
- Ito, T. A., & Bartholow, B. D. (2009). The neural correlates of race. Trends in Cognitive Sciences, 13(12), 524–531.
- Ito, T. A., & Senholzi, K. B. (2013). Us versus them: Understanding the process of race perception with event-related brain potentials. Visual Cognition, 21(9–10), 1096–1120.
- Ito, T. A., & Tomelleri, S. (2017). Seeing is not stereotyping: The functional independence of categorization and stereotype activation. Social Cognitive and Affective Neuroscience, 12(5), 758–764.
- Ito, T. A., & Urland, G. R. (2003). Race and gender on the brain: Electrocortical measures of attention to the race and gender of multiply categorizable individuals. Journal of Personality and Social Psychology, 85(4), 616–626.
- Ito, T. A., & Urland, G. R. (2005). The influence of processing objectives on the perception of faces: An ERP study of race and gender perception. Cognitive, Affective and Behavioral Neuroscience, 5(1), 21–36.
- Kaiser, C. R., & Spalding, K. E. (2015). Do women who succeed in male-dominated domains help other women? The moderating role of gender identification. European Journal of Social Psychology, 45, 599–608.
- Kecskés-Kovács, K., Sulykos, I., & Czigler, I. (2013). Is it a face of a woman or a man? Visual mismatch negativity is sensitive to gender category. Frontiers in Human Neuroscience, 7, 532.
- Kubota, J. T., & Ito, T. A. (2007). Multiple cues in social perception: The time course of processing race and facial expression. Journal of Experimental Social Psychology, 43(5), 738–752.
- Kubota, J. T., & Ito, T. A. (2017). Rapid race perception despite individuation and accuracy goals. Social Neuroscience, 12(4), 468–478.
- Langner, O., Dotsch, R., Bijlstra, G., Wigboldus, D. H. J., Hawk, S. T., & van Knippenberg, A. (2010). Presentation and validation of the Radboud Faces Database. Cognition and Emotion, 24(8), 1377–1388.
- Ma, Y., Ge, J., Xu, X., Fan, Y., Yang, S., & Han, S. (2009). Asymmetric neurocognitive representation of ethnic in-group/out-group faces. Chinese Science Bulletin, 54, 2076–2081.
- Mouchetant-Rostaing, Y., & Giard, M. H. (2003). Electrophysiological correlates of age and gender perception on human faces. Journal of Cognitive Neuroscience, 15(6), 900–910.
- Mouchetant-Rostaing, Y., Giard, M. H., Bentin, S., Aguera, P., & Pernier, J. (2000). Neurophysiological correlates of face gender processing in humans. European Journal of Neuroscience, 12, 303–310.
- Ouwerkerk, J. W., De Gilder, D., & De Vries, N. (2000). When the going gets tough, the tough get going: Social identification and individual effort in intergroup competition. Personality and Social Psychology Bulletin, 26(12), 1550–1559.
- Preacher, K. J., Curran, P. J., & Bauer, D. J. (2006). Computational tools for probing interaction effects in multiple linear regression, multilevel modeling, and latent curve analysis. Journal of Educational and Behavioral Statistics, 31, 437–448.
- Schyns, B., & Schilling, J. (2011). Implicit leadership theories: Think leader, think effective? Journal of Management Inquiry, 20(2), 141–150.
- Senholzi, K. B., & Kubota, J. T. (2016). The neural mechanisms of prejudice intervention. In J. R. Absher & J. Cloutier (Eds.), Neuroimaging Personality, Social Cognition, and Character (pp. 337–354). Cambridge, MA: Academic Press.
- Stangor, C., Lynch, L., Duan, C., & Glass, B. (1992). Categorization of individuals on the basis of multiple social features. Journal of Personality and Social Psychology, 62(2), 207–218.
- Sun, Y., Gao, X., & Han, S. (2010). Sex differences in face gender recognition: An event-related potential study. Brain Research, 1327, 69–76.
- Tajfel, H., & Turner, J. C. (1986). The social identity theory of intergroup behavior. In S. Worchel & W. G. Austin (Eds.), The Psychology of intergroup relations (pp. 7–24). Chicago IL: Nelson-Hall.
- Taylor, S. E., Fiske, S. T., Etcoff, N. J., & Ruderman, A. J. (1978). Categorical and contextual bases of person memory and stereotyping. Journal of Personality and Social Psychology, 36, 778–793.
- Turner, J. C., Hogg, M. A., Oakes, P. J., Reicher, S. D., & Wetherell, M. S. (1987). Rediscovering the social group: A self-categorization theory. New York, NY: Basil Blackwell.
- Van Hooff, J. C., Crawford, H., & Van Vugt, M. (2011). The wandering mind of men: ERP evidence for gender differences in attention bias towards attractive opposite sex faces. Social Cognitive and Affective Neuroscience, 6(4), 477–485.
- Van Veelen, R., Derks, B., & Endedijk, M. D. (2019). Double trouble: How being outnumbered and negatively stereotyped threatens career outcomes of women in STEM. Frontiers in Psychology, 10(150), 1–18.
- Veldman, J., Meeussen, L., Van Laar, C., & Phalet, K. (2017). Women (do not) belong here: Gender-work identity conflict among female police officers. Frontiers in Psychology, 89(130), 1–12.
- Volpert-Esmond, H. I., Merkle, E. C., & Bartholow, B. D. (2017). The iterative nature of person construal: Evidence from event-related potentials. Social Cognitive and Affective Neuroscience, 12(7), 1097–1107.
- Wilder, D. A. (1984). Perceptions of belief homogeneity and similarity following social categorization. British Journal of Social Psychology, 23, 323–333.
- Willadsen-Jensen, E. C., & Ito, T. A. (2006). Ambiguity and the timecourse of racial perception. Social Cognition, 24(5), 580–606.
- Willadsen-Jensen, E. C., & Ito, T. A. (2008). A foot in both worlds: Asian Americans’ perceptions of Asian, White, and racially ambiguous faces. Group Processes & Intergroup Relations, 11(2), 182–200.
- Willadsen-Jensen, E. C., & Ito, T. A. (2015). The effect of context on responses to racially ambiguous faces: Changes in perception and evaluation. Social Cognitive and Affective Neuroscience, 10, 885–892.
- World Medical Association Declaration of Helsinki. (1991). Law, Medicine and Health Care, 19(3–4), 264–265.