ABSTRACT
Introduction
Acute-on-chronic failure (ACLF) is a recognized syndrome in patients with chronic liver disease and is characterized by acute decompensation, organ failure(s), and a high short-term mortality. ACLF is often triggered by ongoing alcohol consumption, gastrointestinal bleeding and/or infections, and is pathophysiologically characterized by uncontrolled systemic inflammation coupled with paradoxical immunoparesis. Patients with ACLF require prompt and early recognition. Management requires extensive utilization of clinical resources often including escalation to intensive care.
Areas covered
Currently, there are no specific targeted treatments for established ACLF, and management revolves around treating underlying precipitants and providing organ support. In this article, we review the epidemiology and pathophysiology of ACLF and summarize recent advances in management strategies of this syndrome, focusing specifically on novel emerging therapies.
Expert commentary
ACLF is a challenging condition with rapid clinical course, high short-term mortality and varying clinical phenotypes. Management of ACLF is broadly focused on supportive care often in an intensive care setting with liver transplantation proving to be an increasingly relevant and effective rescue therapy. This disease has clear pathogenesis and epidemiological burden, thus distinguishing it from decompensated cirrhosis; there is clear clinical need for the development of specific and nuanced therapies to treat this condition.
1. Introduction
Advanced liver fibrosis (traditionally termed liver cirrhosis) represents end-stage liver disease and is associated with disruption of hepatic synthetic function and portal hypertension. Cirrhosis is a continuum ranging from the relatively stable and largely asymptomatic ‘compensated phase’ to the more advanced ‘decompensated phase,’ characterized by the development of ascites, portal hypertensive gastrointestinal bleeding, encephalopathy, and jaundice [Citation1]. The development of a decompensating event increases mortality amongst patients with cirrhosis; median survival falls from 12 years in compensated cirrhosis to 2 years with decompensation [Citation1]. Investigators have defined a distinct syndrome within acutely decompensated cirrhotic patients called Acute-on-Chronic Liver Failure (ACLF) [Citation2] which carries a 90-day mortality in excess of 50% [Citation3–5]. ACLF is characterized by the development of extrahepatic organ failure (OF) in a patient with preexisting cirrhosis [Citation6]; it is associated with a high 28-day mortality [Citation4]. The discovery of ACLF as a distinct syndrome has led to novel insights into disease pathogenesis and has the potential to allow identification of new therapeutic targets to ameliorate the excess mortality seen in this patient population.
2. Defining ACLF
Controversy exists regarding a consensual definition and diagnostic criteria for ACLF with up to 13 noted definitions of ACLF each with variable scope and differing prognostic values [Citation7]. Whilst there are notable differences between definitions by various major societies, the two most widely accepted definitions are the Asian Pacific Association for the Study of the Liver (APASL) criteria and the European Association for the Study of Chronic Liver Failure (EASL-CLIF) criteria. (). APASL defines ACLF as ‘an acute hepatic insult manifesting as jaundice (serum bilirubin ≥5 mg/dL) and coagulopathy (INR [international normalised ratio] ≥1.5) complicated within 4 weeks by ascites and/or encephalopathy in a patient with previously diagnosed or undiagnosed chronic liver disease. It is associated with a high 28-day mortality.’ Patients with prior decompensation and those with acutely decompensated cirrhosis are not included in the definition. Extrahepatic insults such as gastrointestinal hemorrhage and sepsis are considered complications of the syndrome, rather than precipitating events [Citation5]. The EASL criteria require an acute decompensation (ascites, bacterial infection, gastrointestinal bleeding, and/or hepatic encephalopathy) followed by the development of one or more organ failures [Citation4]. The heterogeneity in definition is partly due to regional differences in ACLF precipitants; alcoholic hepatitis for the EASL-CLIF ACLF cohort compared to mainly viral infections in Asia (superadded hepatitis E or reactivation of hepatitis B) [Citation8]. Thus, cirrhosis is not a pre-requisite for the APASL diagnosis of ACLF but remains a key criterion for the EASL-CLIF ACLF diagnosis. Also, there is focus on liver failure by the former and extra-hepatic organ failure by the later definition. This raises the question whether both definitions refer to different time points in the pathophysiological journey of ACLF. The North American Consortium for the Study for End-Stage Liver disease (NACSELD) and the Chinese Group on the Study of Severe Hepatitis (COSSH) have also provided definitions, which although not consistent, lay groundwork for future research [Citation9,Citation10].
Table 1. Definitions of acute on chronic liver failure (ACLF).
Mahmud et al. compared both widely accepted definitions to ascertain ACLF incidence and mortality in a diverse cohort of patients with compensated cirrhosis, in a retrospective study in the US [Citation11]. Unsurprisingly, incidence and prevalence of ACLF varied based on the definitions used with a significant proportion of patients classified as having ACLF by one criterion alone. In total 4296 patients qualified as having any grade of ACLF by the EASL-CLIF criteria and 574 individuals met the APASL ACLF criteria (10.2%). The short-term mortality of those meeting the APASL ACLF criteria was similar to patients with grade 2 EASL-CLIF ACLF in the cohort. Whilst these findings highlight the heterogeneity in defining ACLF around the world, it remains unclear whether nuanced geographical definitions or a cogent universally accepted, international definition would be best for patient care; the latter would certainly help standardize international, multicenter datasets.
3. Epidemiology
Predicting the worldwide prevalence of ACLF is challenging due to the lack of a universal definition. Previously, prevalence of ACLF in hospitalized patients was estimated to be between 24% and 40% [Citation12–14]. A recent systematic review and meta-analysis, constituting the largest epidemiological study on this subject to date, estimated the global prevalence of ACLF to be 35% of hospitalized patients with cirrhosis (95% CI: 33% to 38%) using the EASL-CLIF criteria [Citation15]. The authors found the 90-day mortality rate to be 58% (95% CI: 51% to 64%), with marked regional variation. (ACLF 90-day mortality was 41%, 56%, 68%, and 73% in North America, Europe, South Asia, and South America, respectively). Alcohol was the most frequent etiology worldwide (45%), showing the highest prevalence in Europe. Similar to the European PREDICT trial (Predicting Acute on Chronic Liver Failure) data, bacterial infection, and severe alcoholic hepatitis, either alone or in combination, resulted in acute decompensation and ACLF in 96% of patients included in the analysis [Citation16]. Mezzano et al al confirm that the most frequent ACLF triggers globally were bacterial infections (35%), followed by gastrointestinal bleeding (22%) and alcohol (19%).
4. Animal models of ACLF
Animal models of ACLF aim to mimic human disease with techniques resulting in chronic liver injury, followed by a precipitating event, usually through administration of D- galactosamine/lipopolysaccharide (LPS). These models exhibited a very high short-term mortality and lacked the clinicopathological manifestations of true ACLF; including portal hypertension, ascites, and multiorgan failure [Citation17–20] Recently, Nautiyal et al. reproduced ACLF in mice by intraperitoneal administration of carbon tetrachloride (CCL4) for 10 weeks followed by an acute injury with acetaminophen (APAP) and LPS. This CCL4/APAP/LPS (CALPS) model replicated the clinical, biochemical, and pathological features of ACLF including hepatocellular injury and necrosis, liver failure, jaundice, ascites, and organ dysfunction [Citation21]. This model is the closest one available to human ACLF and could aid in development of stage specific treatments in this dynamic syndrome.
5. Pathophysiology
Portal hypertension, intestinal dysbiosis, and enhanced gut permeability are key cirrhosis phenomenon [Citation22] (); these set the stage for bacterial translocation and drive the subsequent local and systemic iterative inflammation that is characteristic of cirrhosis [Citation23]. Cirrhotic individuals are thus ‘primed’ to enter catastrophic cycles of inflammation.
Figure 1. Pathophysiology of ACLF.
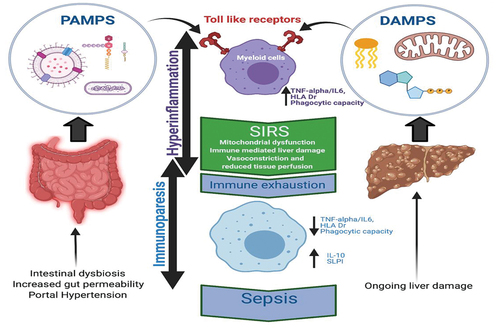
The systemic inflammation hypothesis proposes that the clinical features of acute decompensated cirrhosis and organ failure share a common pathophysiological mechanism. Traditionally, systemic inflammation is known to cause organ dysfunction through stimulating NO production, resulting in worsening of preexisting circulatory dysfunction and activation of immune cells. This then results in tissue damage and impaired organ function [Citation24]. An additional mechanism involving mitochondrial metabolic dysfunction associated with systemic inflammation has recently been proposed [Citation25]. Untargeted blood metabolomics obtained from a large series of patients with AD of cirrhosis, with or without ACLF, alongside immunology data in the context of sepsis [Citation26,Citation27] suggest preferential allocation of circulating nutrients (amino acids, fatty acids, and glucose) to innate immune cells as a result of high metabolic demands. This process is supported by inhibiting nutrient consumption in peripheral organs resulting in decreased mitochondrial energy production and the eventual organ dysfunction/failure seen in individuals with ACLF [Citation28].
ACLF precipitating events vary with geographical location [Citation1] but broadly include infections and GI bleeding [Citation22]. Such triggers initially drive decompensation events such as renal failure or ascites and then hepatic and extrahepatic OFs. Whilst it is clear that an exaggerated inflammatory response is a key feature underlying ACLF pathophysiology, what is less well understood is the associated paradoxical immunoparesis which portends to overwhelming sepsis, multiple OF and poor short-term survival [Citation29]. Inflammation and immunoparesis are thus key pathophysiological features of ACLF ().
6. Pre-ACLF state
The PREDICT study identified three distinct clinical courses in acute decompensated cirrhosis after hospital admission. In this prospective, multicenter, observational study, the investigators noted that the development of systemic inflammation was the key driver of disease progression and stratified patients into three groups with distinct clinical trajectories. The first group defined was the patients with ‘pre-ACLF.’ This cohort developed ACLF within 90 days and had a 3-month and 1-year mortality of 53.7% and 67.4%, respectively. The second group exhibited a clinical course of unstable decompensated cirrhosis, whilst they did not develop full blown ACLF, these patients were noted to have low-level systemic inflammation and portal hypertensive bleeding complications. This group had a 1-year mortality of 35.6%. Finally, the patients with stable decompensated cirrhosis with no systemic inflammation had a 1-year mortality rate of 9.5% and comprised almost two-third of all patients admitted to hospital with AD [Citation16].
7. Management of ACLF
Currently, there are no specific treatments available for patients with ACLF and management centers on supportive treatment; treating precipitating factors; prevention of complications whilst providing organ support and increasingly liver transplantation. Due to both an improved understanding in the pathogenesis of ACLF and increasing commercial interest from pharmaceutical companies, there are currently several novel therapies being trialed in both clinical and purely experimental settings.
7.1. Managing sepsis and novel biomarkers
Bacterial infections are common in ACLF, either as a complication or as a precipitating event [Citation30]. In infection triggered ACLF, the likelihood of death is four times higher than all other precipitating events [Citation31]. Early diagnosis of sepsis is thus vital and can improve prognosis. Conventional laboratory markers such as C-reactive protein (CRP) and procalcitonin (PCT) can help in diagnosis but levels of both markers are noticeably lower in patients with cirrhosis and infections compared to patients without liver disease [Citation32]. It is clear that effective biomarkers for early diagnosis of sepsis in ACLF are needed and a number of these have been proposed. Interleukin-6 (IL-6) is an acute-phase protein that predicts outcome of patients (90-day and 1-year mortality) with cirrhosis comparable to MELD score and better than CRP and white cell count [Citation33,Citation34].
Soluble triggering receptor expressed on myeloid cells-1 (sTREM-1) (immune cell surface marker, elevated in infections and sepsis) [Citation35,Citation36] and Presepsin (novel inflammatory biomarker stimulating monocyte phagocytosis) [Citation37] have been studied as early diagnostic tests in ACLF-associated sepsis [Citation38]. Both biomarkers demonstrated higher diagnostic efficacy in diagnosing sepsis in ACLF patients compared to traditional markers including white cell count, procalcitonin, and CRP. Furthermore, a combination of presepsin with the traditionally used CLIF-SOFA score revealed the highest diagnostic accuracy in diagnosing sepsis in ACLF patient [Citation38].
7.2. Emerging treatments
7.2.1. Inhibition of toll-like receptor 4 (TLR-4)
TLR-mediated immune cell activation plays a pivotal role in the innate immune response [Citation39]. By recognizing pathogen-associated molecular patterns (PAMPs), including LPS (lipopolysaccharides) and Damage-Associated Molecular Protein (DAMPs) () TLRs activate innate immune cells including macrophages and neutrophils thus initiating inflammatory processes critical to host defense [Citation40]. The liver is continually exposed to a high antigen load from the gut, so hepatic TLR distribution and function is designed to fundamentally facilitate tolerance rather than immune induction. This balance is disrupted in cirrhosis. Furthermore, there is an abundance of both PAMPs and DAMPs in cirrhosis due to a breakdown of the gut-blood barrier, intestinal dysbiosis, and ongoing hepatocyte necroptosis [Citation41]. The resultant uncontrolled TLR-driven innate cell activation is instrumental in driving the low- and high-grade inflammation characteristic of ACLF. ()
7.2.2. TAK-242
Rodent models of ACLF (both bile duct ligation and CCL4 [chemokine ligands 4] plus LPS) confirm that TLR-4-mediated signaling is a key step in the pathogenesis of ACLF. TAK-242 inhibits TLR-4 activation [Citation42,Citation43], thus effectively blocking LPS and DAMPs triggered pro-inflammatory cytokine production (). Whilst this therapy is promising [Citation39], human in vivo data are currently lacking. A randomized-controlled trial (RCT) has been assessing efficacy, safety, and pharmacokinetics of TAK-242 in cirrhotic patients with acute alcoholic hepatitis (AH) and a trial in ACLF is being set up. The primary outcome is changed in CLIF-ACLF score at day 8 of treatment. (NCT04620148)
Figure 2. Novel interventions for ACLF.
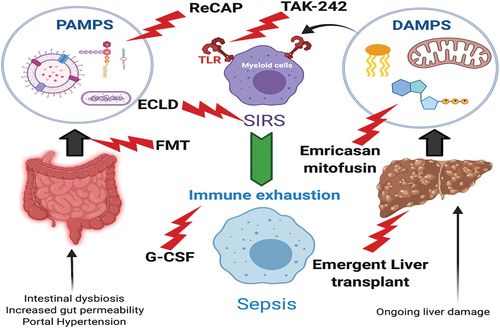
7.2.3. Recombinant alkaline phosphatase
Another novel method of blocking TLR4 is by neutralizing a key PAMP in ACLF; LPS. High serum LPS levels in patients with AH and ACLF are associated with increased risk of death [Citation44] (). LPS is an endotoxin from the cell wall of gram-negative bacteria and can be rendered nontoxic by recombinant alkaline phosphatase (recAP)-mediated dephosphorylation [Citation45,Citation46]. Engelmann et al used a rat model of bile duct ligation with co-administration of LPS to demonstrate that recAP reduced hepatic TLR 4 expression, blunted the inflammatory response and improved organ failures in ACLF [Citation47]. This approach awaits validation in human subjects with ACLF.
7.2.4. gDNA, DAMPs and pan-caspase inhibitor Emricasan
In addition to PAMPs, products from necrotic and apoptotic hepatocyte death (DAMPs) also drive the development of SIRS in the pathogenesis of ACLF [Citation48] (). Whilst end products of apoptotic pathways such as fragmented chromatin, the M-30 component of KRT-18 (keratin) and g-DNA (low molecular weight DNA with size laddering) are seen in patients with ACLF [Citation49], the dominant mode of cell death in ACLF appears to be necrosis. This was confirmed by a subset analysis of 337 patients from the CANONIC dataset by Macdonald et al. [Citation50] Caspase-cleaved keratin 18 (cK18) and Keratin 18(k18) which are derived from apoptotic and necrotic cell death, respectively, were measured in the plasma of patients from this CANONIC subset and the resultant cK18:k18 ratio (apoptotic index) was calculated. There was a statistically significant reduction in cK18:K18 ratio seen in ACLF patients as compared to AD [Citation50], indicating greater hepatocyte necrosis in ACLF. Zheng et al corroborated these findings in chronic HBV (hepatitis B virus) patients. They demonstrated that patients with ACLF had significantly raised levels of both M-30 (derived by caspase-mediated cleavage of Ck18; marker of apoptotic hepatocyte death) and M-65 (marker of total hepatocyte death; necrosis and apoptosis) and decrease in the M30/65 ratio associated with poorer clinical outcomes [Citation51]. Other investigators hypothesize that the dominant mode of cell death driving ACLF is apoptosis with Adebayo et al demonstrating a high apoptosis index (M-30/M-65 ratio) in patients with ACLF. It is important to note, however, that the comparison group was patients with acute liver failure in this study, where far higher levels of necrosis are to be expected [Citation49].
Studies involving the caspase inhibitor Emricasan support necrosis as the dominant cell death modality in ACLF [Citation52] (). Caspase inhibitors block apoptosis and have a hepato-protective effect in rodent models of cholestatic and fatty liver disease [Citation53]. Frenette et al demonstrated that oral administration of Emricasan for 3 months in patients with cirrhosis resulted in improved liver function (MELD score, Child-Pugh score; INR and total bilirubin) compared to placebo [Citation54]; this RCT excluded patients with advanced liver disease (CTP-C) and advanced organ failure, thus effectively excluding ACLF patients [Citation54]. When Emricasan was studied in ACLF (phase 2 multicenter, double-blind, randomized trial) [Citation55], an expected reduction in apoptotic markers was observed but there was no improvement in either the MELD or CLIF-C ACLF/CLIF-C AD scores.
7.2.5. Mitofusin-2 – a protective target in the liver
Mitochondrial fusion protein 2 (Mfn2) has multiple biological functions including vital effects on apoptosis and autophagy [Citation56]. The balance between the two is mediated via the BNIP3 (BCL2 and adenovirus E1B 19-kDA-interacting protein 3)-mediated signaling pathway [Citation57]. Xue et al conducted a study using an ACLF animal model and a hepatocyte autophagy model by delivering Mfn2 to liver cells using adenovirus and lentivirus. Anti-apoptotic effects of Mfn2 overexpression were demonstrated by increased and decreased Bcl-2 (inhibitor of apoptosis) and Bax (inducer of apoptosis) levels, respectively. Autophagy was triggered with Mfn2 via the P13k/Akt/mTor signaling pathway, signaling a role in alleviating liver injury in ACLF [Citation58]. Whilst necrosis is likely the key driver of ACLF, there is likely a contribution from apoptotic cell death and thus Mitofusin-2 may be part of future combination therapies in these patients ().
7.2.6. Oxysterol sulfates: 25-hydroxycholesterol 3-sulfate (25HC3S)
Oxysterol sulfates are a new class of anti-inflammatory drugs under clinical evaluation and play an important role in lipid metabolism, inflammatory response, and cell survival through epigenetic modification [Citation59]. A phase 2a, open-label study is underway studying the oxysterol sulfate DUR-928 in moderate-severe AH. (NCT03917407) If efficacy is demonstrated, then the role of this in the management of ACLF is likely to be explored.
7.2.7. Statins
Statins are established treatment agents for hypercholesterolemia. In addition, they have antioxidative, antiproliferative, and anti-inflammatory properties [Citation60]. Statins, in studies from the early 2000s, have been shown to increase hepatic nitric oxide release and decrease hepatic resistance in patients with portal hypertension and cirrhosis [Citation61]. Additional hepatoprotective and anti-inflammatory properties were demonstrated in subsequent experimental studies [Citation62,Citation63]. Investigators have also demonstrated that statins decrease portal pressure (measured by hepatic venous pressure gradient) in patients with cirrhosis [Citation64,Citation65], with beneficial effects on survival noted in patients presenting with variceal bleeding [Citation66]. As clinically significant portal hypertension (CSPH), perhaps more specifically rising portal pressures, heralds the onset of ACLF [Citation67], it is unsurprising therefore that the effects of statins in patients with decompensated cirrhosis is an active area of ACLF research. Currently, two RCTs, one comparing Simvastatin (20 mg/day) and Rifaximin (1200 mg/day) with placebo in patients with Child-Pugh B/C cirrhosis to prevent ACLF (NCT03780673), and another examining the effects of Atorvastatin (20 mg per day) on survival and hospitalization in patients with cirrhosis and CSPH (NCT04072601), are underway. These trials will also address the safety concerns surrounding statin use in advanced liver disease; this remains a particularly relevant outcome when examining statin use in liver disease given the early results from the LIVER-HOPE SAFETY trial. This trial revealed that simvastatin 40 mg/day plus rifaximin in patients with decompensated cirrhosis was associated with significant increase in adverse events (rhabdomyolysis being the most common) requiring treatment withdrawal, compared with simvastatin at 20 mg/day plus rifaximin [Citation68].
7.2.8. Granulocyte colony stimulating factor (G-CSF)
Perpetual inflammation and the resultant immune cell exhaustion contribute to the immunoparesis seen in ACLF [Citation69].() G-CSF or Granulocyte colony stimulating factor, with its ability to stimulate proliferation and differentiation of neutrophil progenitor cells, thereby potentially resetting a paralyzed immune system is an attractive proposition in ACLF. () G-CSF is hypothesized to drive hepatic regeneration in ACLF in a stem cell-dependent mechanism; in animal models of liver failure G-CSF enhanced mobilization of hematopoietic stem cells and then proliferation of hepatic progenitor cells [Citation70]. The results in human cirrhosis are however less convincing; small RCTs’ in patients with stable decompensated cirrhosis have generated different outcomes and drawing firm conclusions is challenging due to heterogeneity of the studies [Citation71–74], and this point is further highlighted in a recent meta-analysis which revealed conflicting outcomes between European and Asian studies [Citation75].
G-CSF therapy in patients with HBV-associated ACLF improved liver function and 3-month survival (48.1% compared to 21.4% in the control group; P = 0.018; n = 28) [Citation76]. Survival benefits have also been noted with G-CSF in alcoholic cirrhosis and concomitant biopsy-proven alcoholic steatohepatitis (ASH) [Citation77]. In a double-blind, randomized, placebo-controlled trial, G-CSF was co-administered with darbepoetin α (GDP group) for 4 weeks (n = 29), or only placebo (n = 26). All patients also received standard medical therapy. At 12 months, higher proportion of patients in the GDP group survived in comparison with controls (68.6% vs 26.9%: 0.003). The GDP group demonstrated reduced liver severity scores (CTP reduction of 48.6% vs 39.1%; p = 0.001, MELD score reduction by 40.4% vs 33%; p = 0.03) and developed less ACLF [Citation78].
More recently, G-CSF was evaluated in patients with ACLF. In a prospective, open-label phase II study 176 patients with ACLF (EASL-CLIF criteria) were randomized to receive either G-CSF plus standard medical therapy (SMT) (n = 88) or SMT alone. G-CSF in comparison with SMT had no significant effect on 90-day transplant-free survival (34.1% vs 37.5%: HR1.05; 95% CI:0.71–1.55; p = 0.805). G-CSF also did not improve liver function scores and occurrence of infections [Citation79]. At present, there is therefore insufficient evidence to recommend use of G-CSF to treat patients with decompensated cirrhosis or ACLF.
7.2.9. Mesenchymal stem cells (MSCs) transplantation
MSCs are multipotent cells with ability to regenerate and differentiate into various type of cells, including hepatocytes [Citation80], and possess immunomodulatory properties [Citation81]. Studies have shown bone marrow-derived MSCs ameliorate liver fibrosis and protect against fulminant hepatic failure in mice [Citation82,Citation83]. Umbilical-cord derived MSCs in Hepatitis B associated ACLF were given in an open-label-controlled study. The results showed improvement in liver function tests and decreased MELD score indicating increased survival rates [Citation84]. A systematic review on stem MSC transplantation in ACLF due to hepatitis B (Three studies, 198 patients [91 treated with MSC and 107 on standard medical therapy]) concluded a significant reduction in the mortality rate and bilirubin at three months with a good safety profile [Citation85]. Another systematic review and meta-analysis assessing clinical performance of MSC therapy in ACLF (4 RCT and 6 non-randomized controlled trials) showed short-term improvement in liver function tests and MELD scores [Citation86]. In conclusion, the use of MSC therapy in ACLF in real-world settings requires more studies as current evidence confines the use to research and experimental use.
7.2.10. N-Acetylcysteine (NAC)
NAC is a glutathione precursor and well-established treatment option in paracetamol-induced ALF [Citation87]. NAC has the effect of scavenging free radicals and antioxidation, helping mitochondrial function, inhibit inflammation and improve hepatic function and promote repair of hepatocytes [Citation88,Citation89]. Wang et al studied the effect of NAC treatment on HBV-related ACLF in a retrospective study in a total of 90 patients (42 and 48 patients in NAC treated and control group, respectively). NAC treatment improved intrahepatic cholestasis, coagulation function, and liver biochemistry [Citation90].
7.2.11. Albumin
Albumin use in decompensated cirrhosis is well established in an acute/short-term setting to address hypovolemia and associated complications. It is universally recommended in treating spontaneous bacterial peritonitis (SBP), hepatorenal syndrome (HRS), and for patients undergoing large volume paracentesis (LVP) [Citation91]. Recent data have highlighted the non-oncotic properties of albumin, making it a biologically plausible treatment agent in decompensated cirrhosis. These functional features include homeostatic effects, antioxidation by scavenging-free radicals, immunomodulation, endothelial stabilization, and binding toxic metabolites including bile acids [Citation92]. Use of albumin in the setting of decompensated cirrhosis and ACLF has evolved dramatically over the last decade albeit without a consensus on dosage, duration, and frequency of administration. Long-term albumin administration in the setting of decompensated cirrhosis has generated interest, resulting in three large RCT’s in the last 3 years [Citation93–95].
The ATTIRE trial (Albumin to prevent infection in chronic liver failure) was published recently [Citation94]. This multicenter RCT included 777 adult patients with cirrhosis, hospitalized for acute decompensation with or without ACLF and hypoalbuminemia (serum albumin <30 g/L). The mean MELD score was 20, and 66% of patients were admitted because of new-onset or worsening ascites. Patients were randomized to receive 20% human albumin (median 200 g; IQR 140–280 g) to target albumin level ≥30 g/L, as compared to standard of care with control group receiving 20 g of albumin (IQR 0–120 g). The composite primary endpoint was new infection, renal dysfunction, or death between days 3 and 16 after initiation of treatment. The percentage of patients with primary end-point event did not differ significantly between targeted albumin group (29.7%) and standard-care group (30.2%); p = 0.87.
The ANSWER study, a multicentric, open-label study enrolled patients with stable decompensated cirrhosis and uncomplicated ascites. The 18-month survival (primary endpoint) was significantly higher in patients receiving SMT and albumin (77%) as opposed to SMT alone (66%; p = 0.028). Long-term albumin reduced the need for paracentesis (54%) and the incidence of refractory ascites (57%). In addition, rates of SBP, renal dysfunction, HRS type 1, hepatic encephalopathy (grade 3 or 4) and potential diuretics-induced side-effects were significantly reduced by 30–67.5% in patients receiving albumin and SMT. These results were further validated in a prospective, non-randomized study enrolling 70 patients with refractory ascites. Patients receiving SMT and albumin (half the dose of ANSWER trial at 20 g/week) had a lower 24-month mortality (41.6%) vs patients receiving SMT alone (65.5%; p = 0.032). This was coupled with a lower risk of emergency hospitalization from SBP, non-SBP infections, and HE [Citation96].
MACHT trial (midodrine and albumin for cirrhosis patients in the waiting list for liver transplantation) had contrasting results showing no survival benefit or probability of developing complications (primary endpoint) for long-term albumin use in decompensated cirrhosis patients, listed for liver transplantation [Citation95]. These contradictory results can be explained by smaller dosage (40 g every 15 days vs 40 g every week in the ANSWER trial) and duration of albumin therapy (mean duration 80 days vs 18 months). Moreover, a loading dose was used in the ANSWER trial resulting in a significant increase of serum albumin 0.6–0.8 g/dL to a median value of nearly 4 g/dL.
There were considerable differences in study design, baseline patient characteristics, dosing and timing of albumin and length of follow-up between the aforementioned three studies. The recent American College of Gastroenterology (ACG) guidelines on ACLF recommend against daily infusion of albumin to maintain albumin >3 g/dL to improve mortality, prevention of renal dysfunction, or infection [Citation97].
PRECOCIA pilot and INFECIR-2 studies by Fernández et al also provide novel insights into the effect of prolonged administration of Human albumin (HA) in patients with decompensated cirrhosis [Citation98]. The PRECOCIA pilot study evaluated the efficacy of long-term HA treatment in prevention of ACLF and mortality. The aim was to identify the HA dose that could normalize serum albumin concentration during 12 weeks of treatment, and then investigate the effects of administration of this albumin dosage for 12 weeks on hypoalbuminemia, cardiocirculatory dysfunction, portal pressure, and systemic inflammation. Out of 18 patients recruited, 10 patients received 1 g/kg body weight of albumin every 2 weeks (low-albumin dose (LA1bD) group which failed to normalize serum albumin (SA) concentration in majority of the patients. As a result, the HA dosage and frequency was increased to 1.5 g/kg body weight every week (high-albumin dose (HA1bD) group). All six patients of HA1bD group presenting with baseline hypoalbuminemia normalized SA concentration (P < 0.001). HA1bD group also showed significant improvement in LV function which is an important contributory mechanism in the cardiocirculatory dysfunction seen in decompensated cirrhosis. These findings also highlighted the dose and frequency required to normalize serum albumin concentration is much higher than that used by other therapeutic trials so far performed. Furthermore, the HA1bD group (not LA1bD) induced a reduction of >20% of IL-6 concentration. This finding prompted extension of the investigation to 13 additional pro-inflammatory cytokines which were suppressed in the HA1bd group. To confirm these results, the investigators also analyzed the blood samples collected in the INFECIR-2 study [multicenter, randomized clinical trial assessing the effect of short-term albumin concentration (1.5 g/kg at diagnosis and 1 g/kg on the third day) in addition to antibiotic therapy, in patients with cirrhosis and acute bacterial infections unrelated to SBP]. A significant reduction in circulating cytokines alongside renin concentration was observed only in patients receiving HA plus antibiotics compared to the antibiotics alone group. Both studies provide useful insights on the dose and frequency of albumin administration to attenuate systemic inflammation and improve cardiocirculatory dysfunction. Implementation of this in real-world settings will be challenging given the cost and utilization of clinical services.
Use of albumin in ameliorating paracentesis-induced circulatory dysfunction (PICD) in setting of ACLF was studied in a RCT. In total, 80 patients undergoing <5 L paracentesis were randomized to receive albumin (8 g/dL, n = 40) or no albumin (n = 40). Non-albumin group experienced higher PICD (l70% v 40%; p = 0.001), higher incidence of hepatic encephalopathy (50% v 27.5%; p = 0.04), hyponatremia (67.5% v 22.5%; p < 0.001), acute kidney injury (62.5% v 30%; p = 0.001), and inpatient mortality (62.5% v 27.5%; p = 0.003) [Citation99].
Additional clinical trials are needed to identify patient groups who would benefit most from long-term albumin administration. To this effect, the ASIA trial is currently underway assessing efficacy of albumin with SMT as compared to SMT in improving patients’ survival and immune modulation in ACLF. (NCT03754400)
7.3. Role of gut microbiome in ACLF
The gut microbiota plays a cardinal role in development of complications associated with cirrhosis [Citation100]. Increased gut permeability, release of products such as endotoxins, small bowel bacterial overgrowth and translocation predispose individuals to infections ultimately leading to complications of end-stage liver cirrhosis such as spontaneous bacterial peritonitis, hepatic encephalopathy, and ACLF [Citation101] Figurse(). The gut microbiome is altered in patients with liver cirrhosis with quantitative metagenomics analyses demonstrating 75,234 distinct microbial genes in cirrhosis in comparison t healthy controls [Citation102]. Manipulation of the gut microbiota to alleviate gut dysbiosis and immune dysfunction by fecal microbiota transplantation (FMT) from a healthy donor may influence the course of liver disease. To this effect, FMT has been evaluated in severe AH (alcoholic hepatitis), PSC (primary sclerosing cholangitis), NAFLD (nonalcoholic fatty liver disease), and HBV infection [Citation103–107]. In a randomized trial by Bajaj et al., FMT was shown to be associated with short-term reduction in alcohol craving and alcohol misuse-related events over six months [Citation108]. An open-label randomized controlled trial by Ahmad et al evaluating FMT as an adjunctive therapy with antiviral therapy (tenofovir) vs antiviral therapy alone in patients with ACLF (defined by the APASL criteria as MELD>18 with <2 organ failures) due to reactivation of HBV, demonstrated significantly improved transplant-free survival in the FMT + tenofovir group (75% vs 37.5%; P = 0.01) [Citation109]. More recently, the efficacy and safety of FMT was studied in patients with severe alcoholic hepatitis (SAH) ACLF in an open-label clinical trial. Thirty-three patients (13 in the FMT arm; 20 in the standard of care (SOC) arm) with SAH-ACLF were administered FMT and followed on days 7, 28, and 90. FMT improved survival at 28 days (100% v 60%; p = 0.01) and 90 days (53.8% v 25%; p = 0.02) [Citation110]. Larger trials assessing safety and efficacy of FMT in ACLF including other settings of chronic liver diseases are required.
CarbaliveTM is a novel-engineered microporous carbon bead, administered orally, and is designed to absorb and clear LPS and other toxins from the gut thus preventing translocation into blood and liver. The goal being to attenuate LPS-driven immune overactivity and eventually paresis. Preliminary results from an RCT assessing the safety, tolerability, and efficacy have shown promising data but official results are awaited (www.carbalive.eu).
7.4. Emergent transplantation for ACLF
Whilst super-urgent transplantation in acute liver failure with multiorgan failure is an established therapy [Citation111,Citation112], this has not been universally accepted standard of care for critically ill patients with cirrhosis (ACLF patients) for a variety of reasons. Diminished physiological reserve, higher recipient age, concerns about recidivism in those with ethanol as an etiology, and the presence of comorbidities are commonly seen amongst patients with end-stage liver disease. Superadded critical illness in this cohort (i.e. additional hit of ACLF) has traditionally thus been viewed as a contraindication to emergent transplantation due to poor survival.
Emerging data challenge this concept as multiple studies have now demonstrated good post-transplant outcomes in ACLF patients (). 4.9% and 15% of patients from the CANONIC study received liver transplantation for ACLF within 28 and 90 days of admission. The survival for ACLF-2 and ACLF-3 was around 20% without liver transplantation and 80% with transplantation [Citation4]. Further data from studies have consistently shown that one-year survival rates after transplantation for ACLF are above 70% [Citation113,Citation114]. Interrogation of the UNOS database by American investigators confirms findings from the CANONIC experience; Sundaram et al. demonstrated improved survival odds in ACLF-3 patients when transplanted within 30 days of placement to the transplant waiting list, whilst Thuluvath et al found that even in the presence of six organ failures, transplantation resulted in an 81% survival chance at 1 year [Citation115]. Building upon these retrospective analyses that likely included super-selective patients in interested centers, Leary et al prospectively studied around 2800 patients admitted with cirrhosis in a multi-center North American study and found that outcomes after transplantation were identical between stable or decompensated cirrhosis and those that were critically ill with ACLF [Citation116].
A common theme emerging from these studies is how traditional liver disease scores such UKELD, MELD and CTP lack the robustness required to prognosticate patients with ACLF. The CLIF-ACLF score which incorporates the SOFA score arguably provides the most dynamic measurement system to predict outcome in ACLF [Citation3], but again needs validation in wider cohorts of patients. Other critical points that need addressing through further study is timing of transplantation in patients with ACLF and the definition of futility. It is clear that decisions with regard to transplantation need to be taken early but what is perhaps less apparent is what exactly constitutes ‘early’ and what the specific contraindications may be. Artru et al excluded advanced pulmonary and circulatory failure, as well as active sepsis and GI bleeding [Citation113]; other groups have noted better outcomes if patients on renal replacement therapy were excluded. Furthermore, the CANONIC investigators demonstrate that the final grade of ACLF within 81% of ACLF patients occurred between the 3rd and 7th day post-diagnosis [Citation117]; suggesting this may be the best time to take such decision. Ethical debates that address how best to prioritize these patients on national waiting lists whilst maximizing transplant utility and benefit overall also need to take place. Ultimately a rapid but bespoke assessment within an MDT setting that considers patient and disease specific nuances will result in the best overall care, organ allocation, and resource utilization. Recently, a UK wide service evaluation of transplantation for patients with ACLF-2 and −3 has started. As of 8 November 2021, patients have been enrolled in the scheme with seven patients receiving a transplant and six surviving to the last follow up. Further data from around the world should help define futility criteria and lead to clear inclusion and exclusion criteria for the use of this precious resource.
7.5. Artificial liver devices (ECLD)
Liver failure and the resultant OF is in part due to accumulating toxins (including ammonia, urea, bile acids, branch chain aromatic amino acids) and the pro-inflammatory cytokines that DAMPS and PAMPS elicit including cytokines from the Tumor Necrosis Factor (TNF) and Interleukin families (). These cytokines and substances can be removed by artificial liver support systems (). Thus, replacing or complementing the work of a failing liver using an artificial device in ACLF has generated a sizable amount of interest and research [Citation118]. Given the worldwide shortage of organ donors, there has been an understandable focus on possible utility of these devices. However, there is little concrete evidence for recommending these devices in ACLF currently [Citation119]. summarizes the most comprehensively studied ECLD therapies in ACLF.
Table 2. Artificial extracorporeal liver support devices used in ACLF*.
Whilst there is strong evidence to suggest use of plasma exchange in ALF to improve transplant free survival, there is lack of robust evidence to use plasma exchange in ACLF. Qin et al reported the use of plasma exchange compared to SMT in a prospective controlled trial in patients with HBV-associated ACLF [Citation135]. Plasma exchange had improved 90-day (60% v 47%; p = 0.016) and 5-year (43% v 31%; p = 0.013) mortality compared to SMT. The study used the Chinese definition of ACLF which does not require cirrhosis of multiorgan failure as a pre-requisite hence the studied population was highly heterogeneous. (52% patients were non-cirrhotic) A systematic review of plasma exchange in ACLF showed improved 30- and 90-day survival in non-transplanted individuals but further well designed RCTs are required to ascertain the optimal duration and amount of plasma exchange required in the setting of ACLF [Citation136]. APACHE trial is a phase III, multicenter, randomized, open-label trial in ACLF, aimed to determine whether plasma exchange with 5% albumin improves 90-day survival, in comparison with standard medical therapy. (NCT03702920)
Thus, replacing or complementing the work of a failing liver using an artificial device in ACLF has generated a sizable amount of interest and research [Citation118]. Given the worldwide shortage of organ donors, there has been an understandable focus on possible utility of these devices. However, there is little concrete evidence for recommending these devices in ACLF currently [Citation119]. summarizes the most comprehensively studied ECLD therapies in ACLF.
8. Conclusion
ACLF remains a challenge in modern hepatology with no universally accepted definition, devastating consequences, and limited treatment strategies. ACLF is a dynamic syndrome with systemic inflammation, immune paralysis, and multi-organ failure being the hallmarks. Treatment is based on organ support, prevention, and management of complications. Extracorporeal liver support devices have failed to reduce mortality in ACLF patients. Prognostication is challenging given the dynamic course of ACLF and requires day-to-day assessment. Novel therapies targeting the various pathophysiological mechanisms in development of ACLF are desperately needed.
9. Expert opinion
ACLF is a major cause of mortality in patients with cirrhosis and chronic liver disease worldwide. An international consensus definition for ACLF is currently lacking and thus there is heterogeneity in how these patients are identified and subsequently managed in different settings. Whilst it is clear that early diagnosis, prevention of precipitating factors, and aggressive ICU care with organ support improves prognosis; treatment for ACLF is currently broadly restricted essentially to supportive care. There are no target-specific therapies against heightened systemic inflammation or resultant immunoparesis – key pathophysiological drivers of ACLF. The novel therapies outlined in this review illustrate potentially how rampant inflammation and immune cell paresis can be both minimized and modulated. Artificial liver devices to date have failed to replace endogenous hepatic function but do offer a potential for future research. Reliable biomarkers are needed for early detection and prognostication of ACLF and if used in conjunction with existing ACLF scores, may provide robust predictors of both the trajectory of ACLF and markers of response to gauge early intervention for a precision medicine-based approach. A multidisciplinary approach between hepatologists, allied health staff, intensive-care teams and palliative-care physicians remains paramount however in providing personalized care to patients with ACLF. The role of emergent liver transplant in settings where it is available needs to be comprehensively defined and validated with a particular focus on what constitutes futility. Current organ allocation systems clearly disadvantages patients with ACLF and national or even international strategies are required to prioritize ACLF patients with inherent high mortality for earlier access to transplantation. The role of liver transplantation in the management of this cohort is gaining traction, with increased acceptance of the benefits that can be derived by employing this judiciously. With improving understanding of pathophysiology, increased physician awareness and interest from pharmaceutical companies, a bright future to in management of this devastating syndrome look increasingly near.
Article highlights
ACLF is a frequency complication in hospitalized patients with cirrhosis and carries high mortality.
Heterogenous definitions of ACLF are proposed in different regions of world, leading to difficult to interpret epidemiological data.
There are no targeted treatments against heightened systemic inflammation and resultant immunoparesis; hallmarks and key drivers of ACLF.
Recognising and managing sepsis is key and reliable biomarkers are needed for early diagnosis.
Gut microbiota contributes to systemic inflammation and decompensation, hence role of FMT in ACLF needs further trials.
Liver transplantation as a last resort remains a viable option; ACLF being a recognized indication for liver transplantation in many countries.
Declaration of interests
A Chauhan is recipient of a Wellcome trust clinical fellowship and an NIHR clinical lectureship. A Chauhan has received consultation fees from Principia biopharma (now part of Sanofi). The authors have no other relevant affiliations or financial involvement with any organization or entity with a financial interest in or financial conflict with the subject matter or materials discussed in the manuscript. This includes employment, consultancies, honoraria, stock ownership or options, expert testimony, grants or patents received or pending, or royalties.
Reviewer disclosures
Peer reviewers on this manuscript have no relevant financial or other relationships to disclose.
Additional information
Funding
References
- D’Amico G, Garcia-Tsao G, Pagliaro L. Natural history and prognostic indicators of survival in cirrhosis: a systematic review of 118 studies. J Hepatol. 2006;44(1):217–231.
- Jalan R, and Williams R. Acute-on-chronic liver failure: pathophysiological basis of therapeutic options. Blood Purif. 2002;20(3):252–261.
- Jalan R, Saliba F, and Pavesi M, et al. Development and validation of a prognostic score to predict mortality in patients with acute-on-chronic liver failure. J Hepatol. 61(5): 1038–1047. 2014.
- Moreau R, Jalan R, and Gines P, et al. Acute-on-chronic liver failure is a distinct syndrome that develops in patients with acute decompensation of cirrhosis. Gastroenterology. 144(7): 1426–1437, 1437.e1–9. 2013.
- Sarin SK, Kedarisetty CK, and Abbas Z, et al. Acute-on-chronic liver failure: consensus recommendations of the Asian Pacific Association for the Study of the Liver (APASL) 2014. Hepatol Int. 8(4): 453–471. 2014.
- Olson JC. Acute-on-chronic liver failure: management and prognosis. Curr Opin Crit Care. 2019;25(2):165–170.
- Wlodzimirow KA, Eslami S, and Abu-Hanna A, et al. A systematic review on prognostic indicators of acute on chronic liver failure and their predictive value for mortality. Liver Int Off J Int Assoc Study Liver. 33(1): 40–52. 2013.
- Mahtab M, Rahman S, Khan M, et al. Hepatitis E virus is a leading cause of acute-on-chronic liver disease: experience from a tertiary centre in Bangladesh. Hepatobiliary Pancreat Dis Int HBPD INT. 2009;8(1):50–52.
- Bajaj JS, O’Leary JG, and Reddy KR, et al. Survival in infection-related acute-on-chronic liver failure is defined by extrahepatic organ failures. Hepatology. 60(1): 250–256. 2014.
- Wu T, Li J, Shao L, et al. Development of diagnostic criteria and a prognostic score for hepatitis B virus-related acute-on-chronic liver failure. Gut. 67(12): 2181–2191. 2018.
- Mahmud N, Kaplan DE, and Taddei TH, et al. Incidence and mortality of acute-on-chronic liver failure using two definitions in patients with compensated cirrhosis. Hepatology. 69(5): 2150–2163. 2019.
- Bajaj JS, Heuman DM, Hylemon PB, et al. Altered profile of human gut microbiome is associated with cirrhosis and its complications. J Hepatol. 2014;60(5):940–947.
- Shi Y, Yang Y, Hu Y, et al. Acute-on-chronic liver failure precipitated by hepatic injury is distinct from that precipitated by extrahepatic insults. Hepatology. 2015;62(1):232–242.
- Li H, Chen L-Y, Zhang -N-N, et al. characteristics, diagnosis and prognosis of acute-on-chronic liver failure in cirrhosis associated to hepatitis B. Sci Rep. 2016;6(1):25487.
- Mezzano G, Juanola A, and Cardenas A, et al., Global burden of disease: acute-on-chronic liver failure, a systematic review and meta-analysis. Gut. 71(1): 148–155. 2022.
- Trebicka J, Fernandez J, Papp M, et al., PREDICT identifies precipitating events associated with the clinical course of acutely decompensated cirrhosis. J Hepatol. 74(5): 1097–1108. 2021.
- Kuhla A, Eipel C, Abshagen K, et al. Role of the perforin/granzyme cell death pathway in d -Gal/LPS-induced inflammatory liver injury. Am J Physiol-Gastrointest Liver Physiol. 2009;296(5):G1069–G1076.
- Li F, Miao L, Sun H, et al. Establishment of a new acute-on-chronic liver failure model. Acta Pharm Sin B. 2017;7(3):326–333.
- Li X, Wang L-K, Wang L-W, et al. Blockade of high-mobility group box-1 ameliorates acute on chronic liver failure in rats. Inflamm Res Off J Eur Histamine Res Soc Al. 2013;62. 703–709.
- Tripathi DM, Vilaseca M, Lafoz E, et al. Simvastatin prevents progression of acute on chronic liver failure in rats with cirrhosis and portal hypertension. Gastroenterology. 2018;155(5):1564–1577.
- Nautiyal N, Maheshwari D, and Tripathi DM, et al., Establishment of a murine model of acute-on-chronic liver failure with multi-organ dysfunction. Hepatol Int. 15(6): 1389–1401. 2021.
- Cullaro G, Sharma R, Trebicka J, et al. Precipitants of acute-on-chronic liver failure: an opportunity for preventative measures to improve outcomes. Liver Transplant Off Publ Am Assoc Study Liver Dis Int Liver Transplant Soc. 2020;26:283–293.
- Albillos A, Lario M, Álvarez-Mon M. Cirrhosis-associated immune dysfunction: distinctive features and clinical relevance. J Hepatol. 2014;61(6):1385–1396.
- Bernardi M, Moreau R, Angeli P, et al. Mechanisms of decompensation and organ failure in cirrhosis: from peripheral arterial vasodilation to systemic inflammation hypothesis. J Hepatol. 2015;63(5):1272–1284.
- Trebicka J, Fernandez J, Papp M, et al. The PREDICT study uncovers three clinical courses of acutely decompensated cirrhosis that have distinct pathophysiology. J Hepatol. 2020 [July/17];73(4):842–854.
- Ganeshan K, Chawla A. Metabolic regulation of immune responses. Annu Rev Immunol. 2014;32(1):609–634.
- Wang A, Luan HH, Medzhitov R. An evolutionary perspective on immunometabolism. Science. 2019;363(6423):eaar3932.
- Zaccherini G, Aguilar F, and Caraceni P, et al., Assessing the role of amino acids in systemic inflammation and organ failure in patients with ACLF. J Hepatol. 74(5): 1117–1131. 2021.
- Amin A, Mookerjee RP. Acute-on-chronic liver failure: definition, prognosis and management. Frontline Gastroenterol. 2020;11(6):458–467.
- Fernández J, Acevedo J, Wiest R, et al. Bacterial and fungal infections in acute-on-chronic liver failure: prevalence, characteristics and impact on prognosis. Gut. 2018;67(10):1870–1880.
- Mücke MM, Rumyantseva T, Mücke VT, et al. Bacterial infection-triggered acute-on-chronic liver failure is associated with increased mortality. Liver Int Off J Int Assoc Study Liver. 2018;38:645–653.
- Lee K, Hong SG, Park YJ, et al. Evaluation of phenotypic screening methods for detecting plasmid-mediated AmpC beta-lactamases-producing isolates of Escherichia coli and Klebsiella pneumoniae. Diagn Microbiol Infect Dis. 2005;53(4):319–323.
- Remmler J, Schneider C, Treuner-Kaueroff T, et al. Increased level of interleukin 6 associates with increased 90-day and 1-year mortality in patients with end-stage liver disease. Clin Gastroenterol Hepatol Off Clin Pract J Am Gastroenterol Assoc. 2018;16:730–737.
- Fischer J, Silva TE, Silva PE SE, et al. From stable disease to acute-on-chronic liver failure: circulating cytokines are related to prognosis in different stages of cirrhosis. Cytokine. 2017;91:162–169.
- Adly AAM, Ismail EA, Andrawes NG, et al. Circulating soluble triggering receptor expressed on myeloid cells-1 (sTREM-1) as diagnostic and prognostic marker in neonatal sepsis. Cytokine. 2014;65(2):184–191.
- Širanović M, Kovač J, Gopčević S, et al. Human Soluble TREM-1: lung and serum levelS in patients with bacterial ventilator associated pneumonia. Acta Clin Croat. 2011;50:6.
- Shozushima T, Takahashi G, Matsumoto N, et al. Usefulness of presepsin (sCD14-ST) measurements as a marker for the diagnosis and severity of sepsis that satisfied diagnostic criteria of systemic inflammatory response syndrome. J Infect Chemother Off J Jpn Soc Chemother. 2011;17(6):764–769.
- Chen J, Huang Z-B, Li H, et al. Early diagnostic biomarkers of sepsis for patients with acute-on-chronic liver failure: a Multicenter Study. Infect Dis Ther. 2021;10(1):281–290.
- Engelmann C, Sheikh M, Sharma S, et al. Toll-like receptor 4 is a therapeutic target for prevention and treatment of liver failure. J Hepatol. 2020;73(1):102–112.
- Mogensen TH. Pathogen recognition and inflammatory signaling in innate immune defenses. Clin Microbiol Rev. 2009;22(2):240–273.
- Takeuchi O, Akira S. Pattern recognition receptors and inflammation. Cell. 2010;140(6):805–820.
- Bhattacharyya S, Wang W, Tamaki Z, et al. Pharmacological inhibition of toll-like receptor-4 signaling by TAK242 prevents and induces regression of experimental organ fibrosis. Front Immunol. 2018;9:2434.
- Matsunaga N, Tsuchimori N, Matsumoto T, et al. TAK-242 (resatorvid), a small-molecule inhibitor of Toll-like receptor (TLR) 4 signaling, binds selectively to TLR4 and interferes with interactions between TLR4 and its adaptor molecules. Mol Pharmacol. 2011;79(1):34–41.
- Gustot T, and Jalan R. Acute-on-chronic liver failure in patients with alcohol-related liver disease. J Hepatol. 2019;70(2):319–327.
- Peters E, Masereeuw R, Pickkers P. The potential of alkaline phosphatase as a treatment for sepsis-associated acute kidney injury. Nephron Clin Pract. 2014;127(1–4):144–148.
- Bentala H, Verweij WR, der Vlag AH-V, et al. Removal of phosphate from lipid a as a strategy to detoxify lipopolysaccharide. Shock. 2002;18(6):561–566.
- Engelmann C, Adebayo D, Oria M, et al. Recombinant alkaline phosphatase prevents acute on chronic liver failure. Sci Rep. 2020;10(1):389.
- Zhang T, Wang Y, Huang L, et al. Disruption of the gut-liver axis in the pathogenesis of acute-on-chronic liver failure. Eur J Gastroenterol Hepatol. 2018;30(2):130–135.
- Adebayo D, Morabito V, Andreola F, et al. Mechanism of cell death in acute-on-chronic liver failure: a clinico-pathologic-biomarker study. Liver Int. 2015;35(12):2564–2574.
- Macdonald S, Andreola F, Bachtiger P, et al. Cell death markers in patients with cirrhosis and acute decompensation. Hepatology. 2018;67(3):989–1002.
- Zheng S-J, Liu S, Liu M, et al. Prognostic value of M30/M65 for outcome of hepatitis B virus-related acute-on-chronic liver failure. WJG. 2014;20(9):2403–2411.
- Schwabe RF, Luedde T. Apoptosis and necroptosis in the liver: a matter of life and death. Nat Rev Gastroenterol Hepatol. 2018;15(12):738–752.
- Witek RP, Stone WC, Karaca FG, et al. Pan-caspase inhibitor VX-166 reduces fibrosis in an animal model of nonalcoholic steatohepatitis. Hepatology. 2009;50(5):1421–1430.
- Frenette CT, Morelli G, Shiffman ML, et al. Emricasan improves liver function in patients with cirrhosis and high model for end-stage liver disease scores compared with placebo. Clin Gastroenterol Hepatol Off Clin Pract J Am Gastroenterol Assoc. 2019;17:774–783.e4.
- Mehta G, Rousell S, Burgess G, et al. A placebo-controlled, multicenter, double-blind, phase 2 randomized trial of the pan-caspase inhibitor emricasan in patients with acutely decompensated cirrhosis. J Clin Exp Hepatol. 2018;8(3):224–234.
- Hall AR, Burke N, Dongworth RK, et al. Mitochondrial fusion and fission proteins: novel therapeutic targets for combating cardiovascular disease. Br J Pharmacol. 2014;171(8):1890–1906.
- Sebastián D, Zorzano A. When MFN2 (mitofusin 2) met autophagy: a new age for old muscles. Autophagy. 2016;12(11):2250–2251.
- Xue R, Yang J, Jia L, et al. Mitofusin2, as a protective target in the liver, controls the balance of apoptosis and autophagy in acute-on-chronic liver failure. Front Pharmacol. [Internet]. 2019 [cited 2021 Jan 22];10 DOI:10.3389/fphar.2019.00601.
- Wang Y, Li X, Ren S. Knockout of Arabidopsis thaliana VEP1, Encoding a PRISE (Progesterone 5β-Reductase/Iridoid Synthase-Like Enzyme), Leads to Metabolic Changes in Response to Exogenous Methyl Vinyl Ketone (MVK). Metabolites. [Internet] 2021;12(1):11.
- Bosch J, Gracia-Sancho J, Abraldes JG. Cirrhosis as new indication for statins. Gut. 2020;69(5):953–962.
- Zafra C, Abraldes JG, and Turnes J, et al. Simvastatin enhances hepatic nitric oxide production and decreases the hepatic vascular tone in patients with cirrhosis. Gastroenterology. 2004;126:749–755.
- Trebicka J, Hennenberg M, Laleman W, et al. Atorvastatin lowers portal pressure in cirrhotic rats by inhibition of RhoA/Rho-kinase and activation of endothelial nitric oxide synthase. Hepatology. 2007;46(1):242–253.
- Marrone G, Maeso-Díaz R, García-Cardena G, et al. KLF2 exerts antifibrotic and vasoprotective effects in cirrhotic rat livers: behind the molecular mechanisms of statins. Gut. 2015;64(9):1434–1443.
- Abraldes JG, Albillos A, and Bañares R, et al. Simvastatin lowers portal pressure in patients with cirrhosis and portal hypertension: a randomized controlled trial. Gastroenterology. 136(5): 1651–1658. 2009.
- Pollo-Flores P, Soldan M, Santos UC, et al. Three months of simvastatin therapy vs. placebo for severe portal hypertension in cirrhosis: a randomized controlled trial. Dig Liver Dis Off J Ital Soc Gastroenterol Ital Assoc Study Liver. 2015;47:957–963.
- Abraldes JG, Villanueva C, Aracil C, et al. Addition of simvastatin to standard therapy for the prevention of variceal rebleeding does not reduce rebleeding but increases survival in patients with cirrhosis. Gastroenterology. 2016;150(5):1160–1170.e3.
- Trebicka J, Reiberger T, Laleman W. Gut-liver axis links portal hypertension to acute-on-chronic liver failure. Visc Med. 2018;34(4):270–275.
- Pose E, Napoleone L, Amin A, et al. Safety of two different doses of simvastatin plus rifaximin in decompensated cirrhosis (LIVERHOPE-SAFETY): a randomised, double-blind, placebo-controlled, phase 2 trial. Lancet Gastroenterol Hepatol. 2020;5(1):31–41.
- Bernsmeier C, van der Merwe S, Périanin A. Innate immune cells in cirrhosis. J Hepatol. 2020;73(1):186–201.
- Theocharis SE, Papadimitriou LJ, Retsou ZP, et al. Granulocyte-colony stimulating factor administration ameliorates liver regeneration in animal model of fulminant hepatic failure and encephalopathy. Dig Dis Sci. 2003;48(9):1797–1803.
- Prajapati R, Arora A, Sharma P, et al. Granulocyte colony-stimulating factor improves survival of patients with decompensated cirrhosis: a randomized-controlled trial. Eur J Gastroenterol Hepatol. 2017;29(4):448–455.
- Newsome PN, Fox R, King AL, et al. Granulocyte colony-stimulating factor and autologous CD133-positive stem-cell therapy in liver cirrhosis (REALISTIC): an open-label, randomised, controlled phase 2 trial. Lancet Gastroenterol Hepatol. 2018;3(1):25–36.
- Verma N, Kaur A, and Sharma R, et al. Outcomes after multiple courses of granulocyte colony-stimulating factor and growth hormone in decompensated cirrhosis: a randomized trial. Hepatology. 68(4): 1559–1573. 2018.
- De A, Kumari S, Singh A, et al. Multiple cycles of granulocyte colony-stimulating factor increase survival times of patients with decompensated cirrhosis in a randomized trial. Clin Gastroenterol Hepatol. 2021;19(2):375–383.e5.
- Marot A, Singal AK, Moreno C, et al. Granulocyte colony-stimulating factor for alcoholic hepatitis: a systematic review and meta-analysis of randomised controlled trials. JHEP Rep. 2020;2(5):100139.
- Duan X-Z, Liu -F-F, Tong -J-J, et al. Granulocyte-colony stimulating factor therapy improves survival in patients with hepatitis B virus-associated acute-on-chronic liver failure. World J Gastroenterol. 2013;19(7):1104–1110.
- Spahr L, Lambert J-F, Rubbia-Brandt L, et al. Granulocyte-colony stimulating factor induces proliferation of hepatic progenitors in alcoholic steatohepatitis: a randomized trial. Hepatology. 2008;48(1):221–229.
- Kedarisetty CK, Anand L, and Bhardwaj A, et al. Combination of granulocyte colony-stimulating factor and erythropoietin improves outcomes of patients with decompensated cirrhosis. Gastroenterology. 148(7): 1362–1370.e7. 2015.
- Engelmann C, Herber A, Franke A, et al. Granulocyte-colony stimulating factor (G-CSF) to treat acute-on-chronic liver failure: a multicenter randomized trial (GRAFT study). J Hepatol. 2021;75(6):1346–1354.
- Pittenger MF, Mackay AM, Beck SC, et al. Multilineage potential of adult human mesenchymal stem cells. Science. 1999;284(5411):143–147.
- Shi M, Liu Z-W, Wang F-S. Immunomodulatory properties and therapeutic application of mesenchymal stem cells. Clin Exp Immunol. 2011;164(1):1–8.
- Kuo TK, Hung S-P, Chuang C-H, et al. Stem cell therapy for liver disease: parameters governing the success of using bone marrow mesenchymal stem cells. Gastroenterology. 2008;134(7):2111–2121, 2121.e1–3.
- Chang Y-J, Liu J-W, Lin P-C, et al. Mesenchymal stem cells facilitate recovery from chemically induced liver damage and decrease liver fibrosis. Life Sci. 2009;85(13–14):517–525.
- Shi M, Zhang Z, Xu R, et al. Human mesenchymal stem cell transfusion is safe and improves liver function in acute-on-chronic liver failure patients. Stem Cells Transl Med. 2012;1(10):725–731.
- Chen B, Wang Y-H, Qian J-Q, et al. Human mesenchymal stem cells for hepatitis B virus-related acute-on-chronic liver failure: a systematic review with meta-analysis. Eur J Gastroenterol Hepatol. 2018;30(10):1224–1229.
- Xue R, Meng Q, Dong J, et al. Clinical performance of stem cell therapy in patients with acute-on-chronic liver failure: a systematic review and meta-analysis. J Transl Med. 2018;16(1):126.
- Smilkstein MJ, Bronstein AC, Linden C, et al. Acetaminophen overdose: a 48-hour intravenous N-acetylcysteine treatment protocol. Ann Emerg Med. 1991;20(10):1058–1063.
- Millea PJ. N-acetylcysteine: multiple clinical applications. Am Fam Physician. 2009;80(3):265–269.
- Otrubová O, Turecký L, Uličná O, et al. Therapeutic effects of N-acetyl-L-cysteine on liver damage induced by long-term CCl4 administration. Gen Physiol Biophys. 2018;37(1):23–31.
- Wang M-L, Yin X-J, X-L L, et al. Retrospective analysis of the clinical efficacy of n-acetylcysteine in the treatment of hepatitis B virus related acute-on-chronic liver failure. Front Med. [Internet]. 2021 cited 2022 May 10;8. Available from: https://www.frontiersin.org/article/10.3389/fmed.2021.724224
- EASL clinical practice guidelines for the management of patients with decompensated cirrhosis. J Hepatol. 2018;69(2):406–460.
- Arroyo V, García-Martinez R, Salvatella X. Human serum albumin, systemic inflammation, and cirrhosis. J Hepatol. 2014;61(2):396–407.
- Caraceni P, Riggio O, Angeli P, et al. Long-term albumin administration in decompensated cirrhosis (ANSWER): an open-label randomised trial. Lancet. 2018;391(10138):2417–2429.
- China L, Freemantle N, Forrest E, et al., A randomized trial of albumin infusions in hospitalized patients with cirrhosis. N Engl J Med. 384(9): 808–817. 2021.
- Solà E, Solé C, Simón-Talero M, et al. Midodrine and albumin for prevention of complications in patients with cirrhosis awaiting liver transplantation. A randomized placebo-controlled trial. J Hepatol. 2018;69(6):1250–1259.
- Di Pascoli M, Fasolato S, Piano S, et al. Long-term administration of human albumin improves survival in patients with cirrhosis and refractory ascites. Liver Int Off J Int Assoc Study Liver. 2019;39:98–105.
- Bajaj JS, O’Leary JG, and Lai JC, et al. Acute-on-chronic liver failure clinical guidelines. Off J Am Coll Gastroenterol ACG. 2021; 10(14309):ajg.0000000000001595.
- Fernández J, Clària J, Amorós A, et al. Effects of albumin treatment on systemic and portal hemodynamics and systemic inflammation in patients with decompensated cirrhosis. Gastroenterology. 2019;157(1):149–162.
- Arora V, Vijayaraghavan R, Maiwall R, et al. Paracentesis-induced circulatory dysfunction with modest-volume paracentesis is partly ameliorated by albumin infusion in acute-on-chronic liver failure. Hepatology. 2020;72(3):1043–1055.
- Gó I, mez-Hurtado J, Such YS, et al. Gut microbiota-related complications in cirrhosis. World J Gastroenterol. 2014;20(42):15624–15631.
- Garcia-Tsao G, Wiest R. Gut microflora in the pathogenesis of the complications of cirrhosis. Best Pract Res Clin Gastroenterol. 2004;18(2):353–372.
- Qin N, Yang F, Li A, et al. Alterations of the human gut microbiome in liver cirrhosis. Nature. 2014;513(7516):59–64.
- Bajaj JS, Salzman NH, Acharya C, et al. Fecal microbial transplant capsules are safe in hepatic encephalopathy: a phase 1, randomized, placebo-controlled trial. Hepatology. 2019;70(5):1690–1703.
- Philips CA, Phadke N, Ganesan K, et al. Corticosteroids, nutrition, pentoxifylline, or fecal microbiota transplantation for severe alcoholic hepatitis. Indian J Gastroenterol Off J Indian Soc Gastroenterol. 2018;37(3):215–225.
- Philips CA, Pande A, Shasthry SM, et al. Healthy donor fecal microbiota transplantation in steroid-ineligible severe alcoholic hepatitis: a Pilot Study. Clin Gastroenterol Hepatol Off Clin Pract J Am Gastroenterol Assoc. 2017;15:600–602.
- Ren Y-D, Z-S Y, Yang L-Z, et al. Fecal microbiota transplantation induces hepatitis B virus e-antigen (HBeAg) clearance in patients with positive HBeAg after long-term antiviral therapy. Hepatology. 2017;65(5):1765–1768.
- Allegretti JR, Kassam Z, Carrellas M, et al. Fecal microbiota transplantation in patients with primary sclerosing cholangitis: a pilot clinical trial. Am J Gastroenterol. 2019;114(7):1071–1079.
- Bajaj JS, Gavis EA, Fagan A, et al. A randomized clinical trial of fecal microbiota transplant for alcohol use disorder. Hepatology. 2021;73:1688–1700.
- Ahmad J, Kumar M, Sarin SK, et al. PS-163-Faecal microbiota transplantation with tenofovir is superior to tenofovir alone in improving clinical outcomes in acute-on-chronic liver failure due to hepatitis B: an open label randomized controlled trial (NCT02689245). J Hepatol. 2019;70(1):e102.
- Sharma A, Roy A, Premkumar M, et al. Fecal microbiota transplantation in alcohol-associated acute-on-chronic liver failure: an open-label clinical trial. Hepatol Int. 2022;16(2):433–446.
- Mendizabal M, Silva MO. Liver transplantation in acute liver failure: a challenging scenario. World J Gastroenterol. 2016;22(4):1523–1531.
- Liou IW, Larson AM. Role of liver transplantation in acute liver failure. Semin Liver Dis. 2008;28(2):201–209.
- Artru F, Louvet A, Ruiz I, et al. Liver transplantation in the most severely ill cirrhotic patients: a multicenter study in acute-on-chronic liver failure grade 3. J Hepatol. 2017;67(4):708–715.
- Thuluvath PJ, Thuluvath AJ, and Hanish S, et al. Liver transplantation in patients with multiple organ failures: feasibility and outcomes. J Hepatol. 69(5): 1047–1056. 2018.
- Sundaram V, Jalan R, Wu T, et al. Factors associated with survival of patients with severe acute-on-chronic liver failure before and after liver transplantation. Gastroenterology. 2019;156(5):1381–1391.e3.
- O’Leary JG, Bajaj JS, Tandon P, et al. Outcomes after listing for liver transplant in patients with acute-on-chronic liver failure: the multicenter North American consortium for the Study of End-Stage Liver Disease Experience. Liver Transplant Off Publ Am Assoc Study Liver Dis Int Liver Transplant Soc. 2019;25:571–579.
- Perricone G, Jalan R. Acute‐on‐chronic liver failure: a distinct clinical syndrome that has reclassified cirrhosis. Clin Liver Dis. 2019;14(5):171–175.
- Huang K, Ji F, Xie Z, et al. Artificial liver support system therapy in acute-on-chronic hepatitis B liver failure: classification and regression tree analysis. Sci Rep. 2019;9(1):16462.
- Tandon R, Froghi S. Artificial liver support systems. J Gastroenterol Hepatol. [Internet] [cited 2021 Feb 3];36(5):1164–1179.
- Gerth HU, Pohlen M, Thölking G, et al. Molecular adsorbent recirculating system can reduce short-term mortality among patients with acute-on-chronic liver failure-a retrospective analysis. Crit Care Med. 2017;45(10):1616–1624.
- Gerth HU, Pohlen M, Thölking G, et al. Molecular adsorbent recirculating system (Mars) in acute liver injury and graft dysfunction: results from a case-control study. PLOS ONE. 2017;12(4):e0175529.
- Olin P, Hausken J, Foss A, et al. Continuous molecular adsorbent recirculating system treatment in 69 patients listed for liver transplantation. Scand J Gastroenterol. 2015;50(9):1127–1134.
- Cisneros-Garza LE, Del R M-RM, Muñoz-Espinoza LE, et al. The molecular adsorbent recirculating system as a liver support system: summary of Mexican experience. Ann Hepatol. 2014;13(2):240–247.
- Thompson J, Jones N, Al‐Khafaji A, et al. Extracorporeal cellular therapy (ELAD) in severe alcoholic hepatitis: a multinational, prospective, controlled, randomized trial. Liver Transpl. 2018;24(3):380–393.
- Benyoub K, Muller M, Bonnet A, et al. Amounts of bile acids and bilirubin removed during single-pass albumin dialysis in patients with liver failure. Ther Apher Dial Off Peer-Rev J Int Soc Apher Jpn Soc Apher Jpn Soc Dial Ther. 2011;15. 504–506.
- Piechota M, Piechota A, Misztal M, et al., An evaluation of the usefulness of extracorporeal liver support techniques in patients with severe liver dysfunction. Arch Med Sci AMS. 15(1): 99–112. 2019.
- Karvellas CJ, Bagshaw SM, McDermid RC, et al. A Case-Control Study of single-pass albumin dialysis for acetaminophen-induced acute liver failure. Blood Purif. 2009;28(3):151–158.
- Nakae H, Igarashi T, Tajimi K, et al. A case report of hepatorenal syndrome treated with plasma diafiltration (selective plasma filtration with dialysis). Ther Apher Dial Off Peer-Rev J Int Soc Apher Jpn Soc Apher Jpn Soc Dial Ther. 2007;11. 391–395.
- Li M, Li J, Shi Z, et al. Efficacy of various combined blood purification techniques for treating patients with non-viral acute liver failure. Cell Biochem Biophys. 2014;68(3):571–575.
- Fujiwara K, Abe R, Yasui S, et al. High recovery rate of consciousness by high-volume filtrate hemodiafiltration for f ulminant hepatitis. Hepatol Res. 2019;49(2):224–231.
- Kribben A, Gerken G, Haag S, et al. Effects of fractionated plasma separation and adsorption on survival in patients with acute-on-chronic liver failure. Gastroenterology. 2012;142(4):782–789.e3.
- Grodzicki M, Kotulski M, Leonowicz D, et al. Results of treatment of acute liver failure patients with use of the prometheus FPSA system. Transplant Proc. 2009;41(8):3079–3081.
- Oppert M, Rademacher S, Petrasch K, et al. Extracorporeal liver support therapy with prometheus in patients with liver failure in the intensive care unit. Ther Apher Dial Off Peer-Rev J Int Soc Apher Jpn Soc Apher Jpn Soc Dial Ther. 2009;13. 426–430.
- Santoro A, Faenza S, Mancini E, et al. Prometheus system: a technological support in liver failure. Transplant Proc. 2006;38(4):1078–1082.
- Qin G, Shao J-G, Wang B, et al. Artificial liver support system improves short- and long-term outcomes of patients with HBV-associated acute-on-chronic liver failure: a single-center experience. Medicine (Baltimore). 2014;93(28):e338.
- Tan E-X-X, Wang M-X, Pang J, et al. Plasma exchange in patients with acute and acute-on-chronic liver failure: a systematic review. World J Gastroenterol. 2020;26(2):219–245.