ABSTRACT
Background
Little is known about right ventricular dysfunction in non-advanced idiopathic pulmonary fibrosis (IPF) patients without hypoxemia at rest. We evaluated it at rest and during exercise.
Research design and methods
123 IPF patients were evaluated, and 27 met all the following criteria: Gender-Age-Physiology Index score ≤5, modified Medical Research Council dyspnea score ≤3, peripheral oxygen saturation ≥92% at rest, and no history of oxygen therapy. They were submitted to two-dimensional speckle-tracking echocardiography at rest and during cardiopulmonary exercise to analyze right ventricular global longitudinal strain.
Results
Abnormal speckle-tracking echocardiography findings were identified in 10/27 patients (37%), indicating right ventricular (RV) dysfunction. No patients had abnormalities observed in conventional echocardiographic parameters. Significant differences in mPAP were observed between patients with RV dysfunction and those without dysfunction (at rest: 26.0 ± 4.8 vs. 19.1 ± 4.2 mmHg, p = 0.001; during exercise: 51.3 ± 6.4 vs. 36.9 ± 14.7 mmHg, p = 0.002).
Conclusions
RV dysfunction was detected in 37% of non-advanced IPF patients and early recognition was only possible using speckle-tracking echocardiography. Special attention should be given to these patients as RV dysfunction is suggestive of worse prognosis. These patients could benefit from new specific drugs or even oxygen therapy for transitory hypoxia.
1. Introduction
Idiopathic pulmonary fibrosis (IPF) has a higher prevalence with increasing age [Citation1]. IPF generally manifests from age 60 to 70 years and usually has a progressive course, leading to mortality. The mean survival for IPF varies from 2.5 to 3.5 years after diagnosis [Citation1,Citation2]. One of the main predictors of mortality in IPF patients is World Health Organization group III pulmonary hypertension (PH), which is associated with a greater reduction in life expectancy [Citation1,Citation3].
The prevalence of PH in IPF patients varies and depends on IPF severity. In early stages of IPF, PH affects <10% of patients [Citation4]. However, as the disease progresses, its prevalence can range from 32% to 85% [Citation2,Citation3,Citation5].
PH remains poorly investigated among patients in the early stages of IPF because cardiac catheterization – the gold standard for definitive diagnosis of changes in pulmonary circulation and its repercussions in the right chamber [Citation6] – is not usually performed for clinical follow-up. Furthermore, the clinical signs and symptoms are insufficient for a diagnosis of PH in such patients [Citation7,Citation8].
Although accurate assessment of PH relies on invasive methods, changes suggestive of this complication can be noninvasively assessed. The use of transthoracic echocardiography (TTE) allows for conventional analysis of right ventricular (RV) performance. Functional analysis of this chamber has traditionally been performed by measuring tricuspid annular plane systolic excursion (TAPSE), Doppler tissue imaging S′ wave, and RV systolic function based on the fractional area change (FAC) [Citation9]. However, TTE can be inaccurate because the complex RV anatomy can present diverse anatomical characteristics when subjected to different loading conditions [Citation10].
Nevertheless, RV systolic function assessment with TTE can be more accurate using speckle-tracking echocardiography (STE) [Citation10]. This technique can noninvasively and accurately measure subtle myocardial dysfunctions resulting from systolic abnormalities [Citation11], allowing alterations to be perceived even with minor changes in acute post-loading [Citation12].
Currently, information on the early stages of RV dysfunction and its progression to IPF is limited. D’Andrea et al. [Citation13] detected changes in RV contractile function using the STE technique. Nevertheless, this study did not mention the criteria used to define early IPF stages and included patients with severe hypoxemia. In their sample, the mean peripheral oxyhemoglobin saturation (SpO2) at rest was 83%. It is well known that PH gradually emerges with the development of chronic hypoxia. For this reason, it is important to study patients at rest before severe hypoxemia develops in order to elicit any potential influence of other mechanisms, including those that could be treated with available drugs. Moreover, Han et al. [Citation14] evaluated IPF patients treated with sildenafil and showed the benefit of this drug in a subgroup of IPF patients with RV dysfunction. Based on these data, we may suggest that early recognition of ventricular dysfunction is particularly important.
Exercise-induced PH is widely known to precede resting PH [Citation15]. For this reason, in this study, the authors found it relevant to combine the evaluation of RV dysfunction with noninvasive pulmonary artery pressure measures during exercise and cardiopulmonary testing (CPT), which measures the values of oxygen extraction (VO2) and the ratio of expired volume/CO2 output (VE/VCO2).
Compared with patients in very advanced IPF stages, patients with mild or moderate IPF may have variable pressure loads because of their broad spectrum of daily life activities. Different daily activity profiles may influence the pressure load due to transient hypoxemia, which is notably variable in IPF patients over a 24-h period [Citation16] or due to differential pressure changes during exercise. In addition, the presence of tissue mediators [Citation17] may contribute to premature PH in IPF patients. To provide additional information on this issue, this study hypothesized the occurrence of RV dysfunction in non-advanced IPF patients without severe hypoxemia at rest.
2. Patients and methods
This prospective cross-sectional study was conducted at University Hospital of Brasília-Brazil in a pulmonology referral center for diagnosis and treatment of interstitial lung diseases. The study included 123 consecutive IPF patients. The official criteria were used for diagnosis [Citation18].
We included patients meeting the following criteria: SpO2 at rest and in ambient air ≥92%; no history of oxygen therapy; Gender-Age-Physiology Index score ≤5, which corresponds to mild or moderate disease [Citation19]; and modified Medical Research Council (mMRC) dyspnea scale score ≤3 [Citation20]. We excluded patients presenting with left cardiomyopathy, locomotor disease, another severe comorbidity (e.g., stroke, lung cancer, pulmonary thromboembolism), emphysema, or other pulmonary diseases related to IPF. All patients signed an informed consent form. The study was approved by Ethics Committee of Brasília University School of Medicine and the institutional approved number is CAAE: 71022817.2.0000.5558.
2.1. Transthoracic Doppler echocardiography at rest
The examination was performed at rest using Vivid I equipment (GE Healthcare, Milwaukee, WI, USA) with a 2.5–3.5 MHz variable frequency transducer. Doppler analysis was performed in real time, and measurements were obtained from a mean of 3 to 5 beats. Conventional echocardiographic examination was performed to evaluate RV function based on the FAC% (abnormal, <35%), TAPSE (abnormal, <17 mm), and tricuspid annulus (S′) (abnormal, <9.5 cm/s) measured by tissue Doppler [Citation10]. The STE of the RV was considered normal when values were less than −20% [Citation9].
The modified Bernoulli equation was applied to calculate the systolic pulmonary artery pressure (sPAP) from tricuspid regurgitation. A noninvasive estimate of the mean pulmonary artery pressure (mPAP) was calculated using the following formula: 0.61 × sPAP + 2 mmHg [Citation21]. sPAP was assumed to be equal to the RV systolic pressure in the absence of RV outflow tract obstruction or pulmonary stenosis [Citation22]. sPAP values <37 mmHg were considered normal [Citation23].
After measuring the pressure gradient between the RV and right atrium, the estimated right atrial pressure, based on the inferior vena cava inspiratory collapsibility index, was added to the parameter [Citation9,Citation24]. Images were obtained to optimize the best RV visualization position (i.e., apical four-chamber view, the same for RV diameter measurement), grayscale for STE analysis (50–90 frames/s), and sector depth for better resolution.
2.2. Two-dimensional echocardiography using the STE technique to evaluate RV function
Images were analyzed offline with Echo PAC software version 201; (GE Vingmed, Horten, Norway), and the STE technique was used to measure two-dimensional strain [Citation25].
The endocardial region of the RV free wall was manually outlined at the end of the systole and automatically adjusted to include the entire myocardium. Systole in the cardiac cycle was defined using the event-timing feature of the software, which allowed the beginning and end of the RV systole to be delimited.
RV longitudinal strain (RVLS) was evaluated at the basal, medial, and apical portions of the free wall (), and the final result was expressed as the mean value of the three segments. Global longitudinal strain is defined as the percentage of myocardial thickening versus the original fiber length and is conventionally represented as a negative value [Citation25].
2.3. Cycle ergometer CPT associated with dynamic TTE measurements
Using a cycle ergometer (CG-04; Inbramed®, Porto Alegre, RS, Brazil), all patients underwent an incremental ramp protocol with progressively increasing load. After 1-min rest to allow patients to adapt to the cycle ergometer, CPT was started at 60 rotations/min without load (0 W). Subsequently, the load was steadily increased at 5–30 W/min. Thus, the calculated value of the maximum predicted V’O2 (O2 consumed) and patient age, dyspnea level during exercise, and exercise capacity were used to provide an incremental period of 8–12 min, according to ATS recommendations [Citation26]. Expiratory gas measurements were recorded from all patients at each breath for the analysis of ventilatory and cardiovascular variables (Quark PFT; COSMED, Rome, Italy).
The V’O2, V’CO2, V’E, and final expiratory O2 and CO2 pressures were recorded as means over 15 s. Continuous heart rate monitoring and electrocardiography were performed, and pulse oximetry (iPod®; Nonin Medical Inc., Plymouth, MN, USA) measurements were continuously recorded. Systolic and diastolic blood pressures were measured using the auscultatory method at each load increase. The anaerobic threshold was noninvasively estimated using the gas exchange method. The slope of V’O2 versus V’CO2 graph was analyzed on equal scales (V-slope technique) and confirmed using the ventilatory technique, which assessed the behavior of ventilatory equivalents (V’E/V’CO2 and V’E/V’O2) and their respective final expiratory pressures. Dyspnea and muscle fatigue sensations were also evaluated throughout the CPT using the Borg effort perception scale [Citation27].
Echocardiographic images were acquired during CPT in the region of the ventricular apex along the left midclavicular line, which allowed for better tricuspid regurgitation image acquisition. During the test period, images were saved each minute for offline analysis to estimate the sPAP value throughout the test. During the recovery period, tricuspid regurgitation spectral traces were recorded to obtain measurements for 3 min after exercise.
2.4. Statistical analysis
Normally distributed continuous variables are expressed as mean ± standard deviation or as range. Categorical variables are expressed as percentages. Means were compared using the t-test for independent samples. Analyses between mPAP measures and CPT variables were calculated using Pearson correlations. Results were considered statistically significant when p < 0.05. Data were analyzed using SPSS for Mac OS X© (version 25.0.0; SPSS Inc., Chicago, IL, USA).
3. Results
Of the 123 IPF patients evaluated from October to December 2018, 38 were selected for baseline TTE. Five patients were excluded due to the presence of the following comorbidities: endocardial alterations caused by coronary disease (n = 2); dynamic left ventricular outflow tract obstruction (n = 1); and dilated cardiomyopathy with left ventricular dysfunction, left atrial enlargement, and signs of increased left ventricular filling pressure (n = 2). In addition to the five patients excluded following TTE, six refused to participate in the study. Thus, 27 patients completed the study and underwent CPT ().
Of the 27 patients who underwent CPT, 16 were men (59.3%). The mean age was 71.5 ± 8.5 years. Eighteen patients (66.7%) took antifibrotic medication; of these patients, 14 (51.8%) and four (14.8%) took pirfenidone and nintedanib, respectively. During patient enrollment, nine (33.3%) patients were not undergoing antifibrotic therapy.
Dyspnea was classified as mMRC grade 0 in two (7.4%), grade 1 in 12 (44.4%), grade 2 in eight (29.6%), and grade 3 in five (18.5%) patients. Lung function was assessed by mean forced vital capacity as a percentage of the predicted value for the whole sample and by mean DLCO as a percentage of the predicted value, which were 67.3 ± 14 and 51.3 ± 17%, respectively. Data are presented in .
Table 1. Demographic data and functional characteristics of the patient sample
Echocardiographic data analysis revealed that 17 (63%) patients had normal RVLS values, whereas 10 (37%) patients had abnormal RVLS values at rest. STE analysis showed no significant differences in RV dysfunction related to sex or age. Among patients with normal RVLS, 64% were male, whereas the proportion of male patients among those with RV dysfunction was 50% (p = 0.45). The mean age of patients without RV dysfunction (normal RVLS) was 70 ± 8 years, whereas that of patients with RV dysfunction was 73 ± 9 years (p = 0.41). There were no significant differences in spirometry variables between the groups with and without RV dysfunction. Furthermore, no significant differences in SpO2 values at rest (94.2% ± 1.5% vs. 95.1% ± 1.7%; p = 0.18) and DLCO (47% ± 14% vs. 53% ± 19%; p = 0.42) were observed between patients with and without RV dysfunction. We also performed subgroup analyses by further restricting the criteria for hypoxemia. The patients were divided into two groups: SpO2 ≥ 95% and SpO2 < 95% at rest. The proportion of patients with RV dysfunction was 26.7% and 50.0%, respectively, and the difference was not significant (p = 0.21).
The sPAP estimated by echocardiography was ≥37 mmHg in six patients and <37 mmHg in 21 patients. Data for the mPAP, sPAP, RV function parameters measured by two-dimensional echocardiography and tissue Doppler and its relationships with RV function at rest measured by RVLS are summarized in .
Table 2. Baseline echocardiographic parameters and their relationships with RV function using the speckle-tracking strain technique
Of these variables, only mPAP and sPAP showed significantly higher values in patients with abnormal RVLS. This difference persisted after only analyzing patients with normal sPAP at rest (sPAP <37 mmHg). Of these patients, five had abnormal RVLS and 16 had normal RVLS. The mean mPAP values in patients with and without RV dysfunction were 23.0 ± 0.4 and 18.8 ± 4.1 mmHg (p = 0.001), respectively. Four patients from our sample (14.8%) achieved an sPAP of >40 mmHg at rest; three of them showed RV dysfunction.
During exercise, mPAP differences between patients with and without RV dysfunction became more apparent ( and ). All patients with RV dysfunction showed significantly higher mPAP values during exercise. At peak effort, a mean mPAP value of 51.3 ± 6.4 mmHg was reached in patients with RV dysfunction compared with that of 36.9 ± 14.7 mmHg reached in patients without RV dysfunction (p = 0.002) (). During exercise, mPAP differences between patients with and without RV dysfunction were also apparent when only analyzing patients with normal sPAP at rest ().
Figure 3. Estimated mPAP during exercise between patients with and without RV dysfunction in the entire cohort. mPAP, mean pulmonary artery pressure; RV, right ventricular
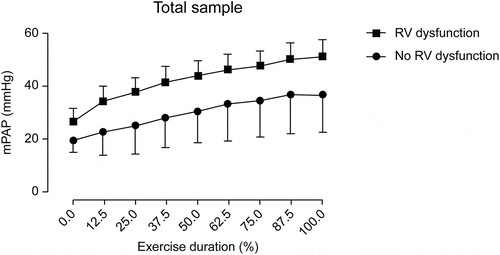
Figure 4. Estimated mPAP during exercise between patients with and without RV dysfunction in normal sPAP subgroup. The normal sPAP subgroup consisted of patients with normal sPAP at rest. mPAP, mean pulmonary artery pressure; RV, right ventricular; sPAP, systolic pulmonary artery pressure; PH, pulmonary hypertension
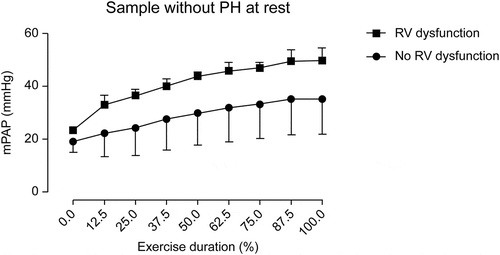
Table 3. CPT data related to RV function
No significant differences in CPT variables were observed between patients with and without RV dysfunction (). The differences in CPT variables remained nonsignificant when subgroup analyses based on more restrictive criteria for hypoxemia were performed ().
Table 4. CPT data related to RV function according to subgroups with SpO2 ≥ 95% and SpO2 < 95%
Sixteen (59.3%) patients had VO2 below the lower limit (<83% of the predicted value). Of these patients, seven (43.8%) patients had RV dysfunction. Of the 11 patients with normal VO2, only three (27.3%) had RV dysfunction (p = 0.38).
Correlation coefficients between mPAP and CPT variables can be seen in . Moderate and significant correlations were achieved between mPAP at peak effort and O2 pulse (r = −0.444; p = 0.020), V’E/V’CO2 volume expired per minute/CO2 ventilation at anaerobic threshold (r = 0.479; p = 0.011), and respiratory rate (r = 0.408; p = 0.035). In relation to mPAP at rest, there was only one moderate significant correlation with O2 pulse (r = −0.425; p = 0.027).
Table 5. Pearson correlation coefficients between CPT data and mPAP at rest or exercise
4. Discussion
This study examined the presence of RV dysfunction in non-advanced IPF patients without severe hypoxemia, excluding those with SpO2 < 92% at rest. Considering that vasoconstriction due to alveolar hypoxia is one of the most important mechanisms of PH, the presence of RV dysfunction in 37% of our sample was an unexpected finding, suggesting that RV changes begin early in the course of IPF, perhaps independently of chronic hypoxia.
Nevertheless, we may not rule out hypoxia as the main cause of PH and RV dysfunction in IPF patients. The presence of subclinical hypoxia may be related to RV impairment in the early stages of IPF. In these stages, vasoconstriction due to alveolar hypoxia may only occur in some areas of the lung, leading to increased localized pulmonary vascular resistance. Although increases in pulmonary resistance caused by a small area with a low ventilation-to-perfusion ratio could imply enlarged pressure load, it would be insufficient in determining clinically relevant hypoxemia. Alternatively, periods of hypoxia associated with daily activities or during sleep could be related to transient increases in mPAP. Such pressure variations could result in subtle changes in RV contractile function, which are only detectable by global longitudinal strain, whereas other conventional parameters measured by TTE would remain within normal ranges.
Rodrigues et al. [Citation16] observed that patients with partial pressures of arterial oxygen (PaO2) >70 mmHg at rest may experience severe hypoxemia (SpO2 < 90%) for a mean of >4 h per day, which would support the periodic occurrence of PH during the day. In agreement with this explanation, a marked increase in mPAP during exercise, which was frequently associated with a concomitant decline in SpO2, was observed in the present study. A previous study reported that mPAP values >40 mmHg were commonly observed during exercise in advanced IPF patients [Citation28].
In addition to vasoconstriction, mechanisms independent of hypoxia may be involved in PH and RV dysfunction, such as fibrosis-induced vascular bed loss or obliteration [Citation29]. Moreover, the presence of mediators of the fibrogenic process may be directly involved in PH development [Citation30].
Values of conventional echocardiography parameters routinely used to evaluate RV function are comparable in groups with and without RVLS -detected RV dysfunction. These parameters lack the sensitivity required to predict RV contractile function changes. Therefore, RVLS calculation is currently the best method to analyze myocardial strain in various clinical situations, allowing for early diagnosis of ventricular dysfunction as also observed by others [Citation31].
RV dysfunction was present in patients with normal sPAP at rest as well as in those with mPAP below the values defining PH. Although mPAP levels at rest were within the normal range, our data revealed significantly higher values for patients with RV dysfunction at rest or during exercise. According to a systematic review [Citation32], the mPAP cutoff value of 25 mmHg used to define PH exceeds the upper limit of normality for cardiac catheterization. In addition, Kimura et al. [Citation33] reported that an mPAP cutoff value of approximately 17 mmHg would significantly discriminate the survival of patients with less advanced IPF stages. Therefore, our findings support the idea that the normal value range used to define the presence or absence of PH is not adequate for this patient group.
In addition, establishing an mPAP cutoff point during maximal exercise can be difficult. Kovacs et al. [Citation32] showed that age is likely an important factor for establishing the normal range. Moreover, there is currently no adequate definition for exercise-induced PH [Citation23].
The RV dysfunction group showed no significant differences in V’O2 or other CPT parameters, but RV dysfunction was subclinical, which suggests that the cardiovascular deficits of these patients were less important than their ventilatory, gas exchange, and muscle function impairments. However, this interpretation contrasts with the findings of Hansen et al. [Citation34]. These divergent results may be due to our small sample size and reduced statistical power.
This study has some limitations. First, the measurement of RV function by two-dimensional STE only allowed RV analysis in the orthogonal plane. Second, we used TTE as a noninvasive approach to evaluate pressure instead of direct catheterization; however, cardiac catheterization is not commonly indicated for non-advanced IPF patients. Finally, our study lacked data on SpO2 during sleep.
There are also issues concerning the interpretation of our findings. First, the detection of RV dysfunction in patients with normal mPAP at rest could potentially be an early prognostic marker in IPF patients and could therefore serve as an additional marker for early lung transplant referral. However, these patients should undergo cardiac catheterization when they meet the criteria for referral for lung transplantation in the late phase of the disease. Second, as RV dysfunction and PH in these patients are not exclusively caused by hypoxia, to what extent they coexist with other factors and whether they could be affected by treatments such as antifibrotic agents and pulmonary vasodilators are unclear.
5. Conclusion
RV dysfunction was detected in 37% of non-advanced IPF patients without severe hypoxemia at rest. Moreover, RV dysfunction was associated with increased mPAP measured by TTE at rest and during exercise. Early recognition was only possible using RVLS, which makes this technique an important tool for evaluating IPF patients. Special attention should be given to patients with RV dysfunction, as they may have worse prognosis and could benefit from new specific drugs or even oxygen therapy for transitory hypoxia in the future.
Article highlights
IPF patients at early stages of disease did not have abnormalities in RV function by conventional echocardiography parameters
Speckle tracking echocardiography detected early RV dysfunction in 37% of those patients
RV dysfunction is associated to pulmonary hypertension in the early stages of IPF
Mean pulmonary pressure during exercise becomes even higher in patients with RV dysfunction compared to those without RV dysfunction
Special attention should be given to patients with RV dysfunction, as they may have worse prognosis.
Declaration of interest
The authors have no relevant affiliations or financial involvement with any organization or entity with a financial interest in or financial conflict with the subject matter or materials discussed in the manuscript. This includes employment, consultancies, honoraria, stock ownership or options, expert testimony, grants or patents received or pending, or royalties.
Reviewer disclosures
Peer reviewers on this manuscript have no relevant financial or other relationships to disclose.
Author contributions
SBC analyzed and interpreted patient data and was a major contributor in writing the manuscript. MPR reviewed all data and made substantial contributions to the conception and design of the work. FXM acquired and analyzed data from CPT. Both NMCF and VVPS selected all patients. VMA reviewed the work. CAMS acquired and analyzed the data and revised the work. All authors read and approved the final manuscript.
Ethics statement
The study was approved by the Ethics Committee of the University of Brasília (approval number-CAAE: 71,022,817.2.0000.5558)
Acknowledgments
The authors specially thank Lauro Casqueiro Vianna, PhD, from NeuroVASQ-Integrative Physiology Laboratory (University of Brasília), for helping in data execution. We would like to thank Editage for English language editing.
Data availability
The data that support the findings of this study are available on request from the corresponding author, SBC. The data are not publicly available due to their containing information that could compromise the privacy of participants.
Additional information
Funding
References
- Ley B, Collard HR, King TE Jr. Clinical course and prediction of survival in idiopathic pulmonary fibrosis. Am J Respir Crit Care Med. 2011;183:431–440.
- Nadrous HF, Pellikka PA, Krowka MJ, et al. Pulmonary hypertension in patients with idiopathic pulmonary fibrosis. Chest. 2005;128:2393–2399.
- Lettieri CJ, Nathan SD, Barnett SD, et al. Prevalence and outcomes of pulmonary arterial hypertension in advanced idiopathic pulmonary fibrosis. Chest. 2006;129:746–1752.
- Klinger JR. Group III pulmonary hypertension: pulmonary hypertension associated with lung disease: epidemiology, pathophysiology, and treatments. Cardiol Clin. 2016;34:413–433.
- Patel NM, Lederer DJ, Borczuk AC, et al. Pulmonary hypertension in idiopathic pulmonary fibrosis. Chest. 2007;132:998–1006.
- McGoon M, Gutterman D, Steen V, et al. Screening, early detection, and diagnosis of pulmonary arterial hypertension: ACCP evidence-based clinical practice guidelines. Chest. 2004;126:14s–34s.
- Kolb TM, Hassoun PM. Right ventricular dysfunction in chronic lung disease. Cardiol Clin. 2012;30:243–256.
- Cobra SB, Cardoso RM, Rodrigues MP. Usefulness of the second heart sound for predicting pulmonary hypertension in patients with interstitial lung disease. Sao Paulo Med J. 2016;134:34–39.
- Lang RM, Badano LP, Mor-Avi V, et al. Recommendations for cardiac chamber quantification by echocardiography in adults: an update from the American society of echocardiography and the European association of cardiovascular imaging. Eur Heart J Cardiovasc Imaging. 2015;16:233–270.
- Giusca S, Dambrauskaite V, Scheurwegs C, et al. Deformation imaging describes right ventricular function better than longitudinal displacement of the tricuspid ring. Heart. 2010;96:281–288.
- Rajagopal S, Forsha DE, Risum N, et al. Comprehensive assessment of right ventricular function in patients with pulmonary hypertension with global longitudinal peak systolic strain derived from multiple right ventricular views. J Am Soc Echocardiogr. 2014;27:657–665.e3.
- Weidemann F, Jamal F, Sutherland GR, et al. Myocardial function defined by strain rate and strain during alterations in inotropic states and heart rate. Am J Physiol Heart Circ Physiol. 2002;283:H792–H799.
- D’Andrea A, Stanziola AA, Saggar R, et al. Right ventricular functional reserve in early-stage idiopathic pulmonary fibrosis: an exercise two-dimensional speckle tracking Doppler echocardiography study. Chest. 2019;155:297–306.
- Han MK, Bach DS, Hagan PG, et al. Sildenafil preserves exercise capacity in patients with idiopathic pulmonary fibrosis and right-sided ventricular dysfunction. Chest. 2013;143:1699–1708.
- Tolle JJ, Waxman AB, Van Horn TL, et al. Exercise-induced pulmonary arterial hypertension. Circulation. 2008;118:2183–2189.
- Rodrigues MP, Vissoci CM, Rosa SP, et al. 24-Hour hypoxia and pulmonary hypertension in patients with idiopathic pulmonary fibrosis. Open Respir Med J. 2017;11:10–16.
- Collum SD, Amione-Guerra J, Cruz-Solbes AS, et al. Pulmonary hypertension associated with idiopathic pulmonary fibrosis: current and future perspectives. Can Respir J. 2017;2017:1430350.
- Raghu G, Remy-Jardin M, Myers JL, et al. Diagnosis of idiopathic pulmonary fibrosis. an official ATS/ERS/JRS/ALAT clinical practice guideline. Am J Respir Crit Care Med. 2018;198:e44–e68.
- Ley B, Ryerson CJ, Vittinghoff E, et al. A multidimensional index and staging system for idiopathic pulmonary fibrosis. Ann Intern Med. 2012;156:684–691.
- Ferris BG. Epidemiology standardization project (American Thoracic Society). Am Rev Respir Dis. 1978;118:1–120.
- Chemla D, Castelain V, Provencher S, et al. Evaluation of various empirical formulas for estimating mean pulmonary artery pressure by using systolic pulmonary artery pressure in adults. Chest. 2009;135:760–768.
- Abbas AE, Fortuin FD, Schiller NB, et al. A simple method for noninvasive estimation of pulmonary vascular resistance. J Am Coll Cardiol. 2003;41:1021–1027.
- Galie N, Humbert M, Vachiery JL, et al. ESC/ERS Guidelines for the diagnosis and treatment of pulmonary hypertension: the joint task force for the diagnosis and treatment of pulmonary hypertension of the European society of cardiology (ESC) and the European respiratory society (ERS): endorsed by: association for European paediatric and congenital cardiology (AEPC), international society for heart and lung transplantation (ISHLT). Eur Heart J. 2015;2016(37):67–119.
- Rudski LG, Lai WW, Afilalo J, et al. Guidelines for the echocardiographic assessment of the right heart in adults: a report from the American society of echocardiography endorsed by the European association of echocardiography, a registered branch of the European society of cardiology, and the Canadian society of echocardiography. J Am Soc Echocardiogr. 2010;23:685–713; quiz 786–788.
- Leitman M, Lysyansky P, Sidenko S, et al. Two-dimensional strain-a novel software for real-time quantitative echocardiographic assessment of myocardial function. J Am Soc Echocardiogr. 2004;17:1021–1029.
- American Thoracic Society; American College of Chest Physicians. ATS/ACCP statement on cardiopulmonary exercise testing. Am J Respir Crit Care Med. 2003;167:211–277.
- Borg GA. Perceived exertion. Exerc Sport Sci Rev. 1974;2:131–153.
- Degani-Costa LH, Levarge B, Digumarthy SR, et al. Pulmonary vascular response patterns during exercise in interstitial lung disease. Eur Respir J. 2015;46(3):738–749. .
- Panagiotou M, Church AC, Johnson MK, et al. Pulmonary vascular and cardiac impairment in interstitial lung disease. Eur Respir Rev. 2017;26:160053.
- Fonseca C, Abraham D, Renzoni EA. Endothelin in pulmonary fibrosis. Am J Respir Cell Mol Biol. 2011;44:1–10.
- Lu KJ, Chen JX, Profitis K, et al. Right ventricular global longitudinal strain is an independent predictor of right ventricular function: a multimodality study of cardiac magnetic resonance imaging, real time three-dimensional echocardiography and speckle tracking echocardiography. Echocardiography. 2015;32:966–974.
- Kovacs G, Berghold A, Scheidl S, et al. Pulmonary arterial pressure during rest and exercise in healthy subjects: a systematic review. Eur Respir J. 2009;34:888–894.
- Kimura M, Taniguchi H, Kondoh Y, et al. Pulmonary hypertension as a prognostic indicator at the initial evaluation in idiopathic pulmonary fibrosis. Respiration. 2013;85:456–463.
- Hansen JE, Wasserman K. Pathophysiology of activity limitation in patients with interstitial lung disease. Chest. 1996;109:1566–1576.