ABSTRACT
Introduction
Nontuberculous mycobacterial (NTM) lung disease (LD) is the most common clinical manifestation of NTM infection and is a growing health concern. Up to 85% of NTM-LD cases are caused by Mycobacterium avium complex (MAC). Increased awareness of NTM-LD caused by MAC is needed as patients with this disease experience substantial burden and unmet treatment needs.
Areas covered
This review provides clinicians and regulatory and healthcare decision makers an overview of the clinical, economic, and humanistic burden of NTM-LD and the unmet treatment needs faced by patients and clinicians. The review focuses on NTM-LD caused by MAC. A summary of the 2020 NTM guidelines specifically for MAC-LD and an overview of novel treatment options, including amikacin liposome inhalation suspension (ALIS) as the first approved therapy for refractory MAC-LD, and investigational drugs in testing phase are provided.
Expert opinion
Key advancements in NTM-LD management include recent updates to clinical practice guidelines, approval of ALIS for the treatment of refractory MAC-LD, and ongoing clinical trials of investigational treatments. Yet opportunities still exist to improve patient outcomes, including development of better screening tools, such as reliable and responsive biomarkers to help identify high-risk patients, and addressing unmet treatment needs.
1. Introduction
Nontuberculous mycobacteria (NTM) encompass more than 190 species commonly found in water and soil [Citation1]. Human exposure to NTM is considered common, as NTM are ubiquitous in the environment [Citation2]. NTM infection in susceptible individuals is believed to occur via inhalation of aerosolized NTM [Citation2]. NTM can cause infections in various organs and tissues; however, NTM lung disease (LD) is the most common clinical manifestation of NTM infection [Citation3].
NTM-LD is a growing health concern [Citation4]. The objective of this review is to provide clinicians and regulatory and healthcare decision makers with an overview of the clinical, economic, and humanistic burden of NTM-LD, the unmet treatment needs faced by patients and clinicians, and the benefits of new treatments. Considering the diversity of NTM species, differences in their relevance as causative agents of NTM-LD, and the variety of different treatment approaches being investigated, this review focuses on NTM-LD caused by Mycobacterium avium complex (MAC), which encompasses the most common pathogens causing NTM-LD worldwide [Citation4].
2. Pathogenesis
NTM-LD is difficult to treat, in part due to the resistance of NTM to a wide range of antibiotics [Citation5,Citation6]. Post inhalation, NTM may form biofilms along the epithelial lining and are engulfed by local immune cells, specifically macrophages [Citation7,Citation8]. Pulmonary macrophages are the main reservoir of NTM in the host [Citation7,Citation8]. In pulmonary macrophages, NTM subvert normal cellular defense mechanisms, persist without being cleared by the immune system, and replicate () [Citation7–9].
Figure 1. NTM Pathogenesis and Dysfunction of the Phagosome
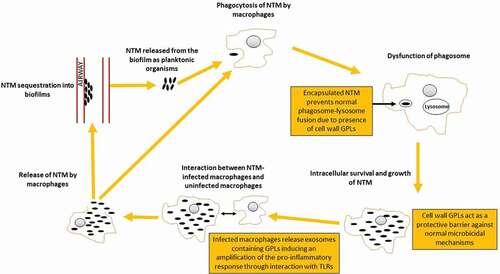
Reduced antibiotic susceptibility is conferred by the relative impermeability of the NTM cell wall, the intracellular localization of the NTM organism, and – for extracellular NTM in the airways – growth in biofilms [Citation10,Citation11]. Intracellular growth and biofilms play important roles in the clinical course of pulmonary mucosal infection by NTM and could explain both the chronic nature of and the difficulty of treating NTM-LD, including infections caused by MAC [Citation6,Citation10,Citation11].
Glycopeptolipids (GPLs) present in the MAC cell wall are key molecules for the survival of MAC in macrophages and for the regulation of the host innate immunity response; thus, GPLs are considered a major MAC pathogenicity factor [Citation9]. An additional role of GPLs is its relationship with biofilm formation as the synthesis of GPLs is important for adhesion and initial establishment of biofilms [Citation9]. The composition and concentration of GPLs are variable by species, can impact colony morphology, and contribute to species-specific pathogenesis and ability to cause disease (i.e. virulence) [Citation12].
Virulence of NTM species differs greatly, and not all of NTM species are able to cause disease in humans [Citation1,Citation2,Citation13]. To date, MAC contains twelve species, the most common being M. avium, M. intracellulare and M. chimaera [Citation14]. A review paper reported that 63% of patients with M. avium isolates had clinically confirmed disease, while M. intracellulare was deemed clinically relevant in 88% of the patients but with greater variation observed among studies [Citation15]. It is not known whether isolates of one species in different countries differ in virulence.
3. Epidemiology
Reliable estimates of the incidence and prevalence of NTM-LD are complicated by the fact that it has not been a reportable disease in Asia, Europe, and most of the United States (US) [Citation16]. Additionally, NTM case definitions can vary across studies, with some reporting the incidence or prevalence based on 1 NTM isolate (i.e. NTM infection) and others based on diagnostic codes or guideline criteria for clinically confirmed disease, which may include microbiologic findings (e.g. ≥ 2 positive culture results for NTM from separate sputum samples), presence of pulmonary or systemic symptoms, and radiologic abnormalities in the lungs [Citation1,Citation16,Citation17]. Population-based epidemiological studies presented below used primary health insurance data and referred to relevant NTM-LD codes. These databases usually have large regional and population coverage; however, they do not include information on specific NTM species. There are fewer studies reporting on the epidemiology of MAC specifically, although several studies have suggested that MAC is by far the most common NTM species identified and the leading cause of NTM infections [Citation4].
In spite of these limitations, some trends are clear from multiple epidemiological investigations. The prevalence of NTM-LD appears to vary by geographic region, being highest in eastern Asia and lower in the US and Europe. Prevalence, expressed as a rate in terms of number of cases per 100,000 inhabitants, was estimated at 24.0 to 29.0 cases of NTM-LD per 100,000 individuals in Japan between 2011 to 2013, and at 33.3 to 39.6 cases of NTM infection per 100,000 individuals in South Korea in 2016 [Citation18–21].
Prevalence of NTM-LD in the US and Europe is similar and appears to be lower than in Asia. In the continental US, prevalence estimates based on health claims data range from 1.4 to 13.9 cases per 100,000 individuals [Citation17]. Prevalence appears to increase substantially in older age groups; among individuals aged 65 years or older and receiving Medicare, these rates were 70.2 cases of NTM-LD per 100,000 individuals [Citation22]. Rates of NTM-LD in Hawaii, a US state in the Pacific Ocean, are however similar to those observed in East Asia and are estimated at 19 cases per 100,000 individuals, with some data suggesting that NTM prevalence could be as high as 44 cases per 100,000 individuals [Citation23].
The annual prevalence of NTM-LD in the European Union (EU)-4 (Spain, Italy, France, and Germany) and the United Kingdom (UK) was similar to US data at 6.2 cases per 100,000 individuals in 2016, ranging from a low of 6.0 cases per 100,000 individuals in Spain to a high of 6.6 cases per 100,000 individuals in the UK [Citation16]. These estimates were derived using a Delphi method with an expert panel in combination with a regional prevalence-estimation model that incorporated data obtained from a blinded physician screening survey and a real-world NTM-LD treating-physician/patient-chart observational study. A recent study in the Netherlands estimated the annual prevalence of NTM-LD using hospital and drug prescription databases (from 2012–2019) to be between 2.3 and 5.9 patients per 100,000 individuals [Citation24]. In Denmark, a study based on a national laboratory using clinical specimens reported annual incidence (i.e. new cases each year) to be 1.2 cases per 100,000 for NTM disease but no prevalence data were reported [Citation25]. A study published in 2020 using retrospective data from electronic medical records in the UK reported that there may be considerable numbers of patients with undiagnosed NTM-LD [Citation26]. The total prevalence of diagnosed and undiagnosed cases of NTM-LD in the UK was estimated to range between 9 and 16 cases per 100 ,000 individuals [Citation26].
While no population-based studies were published from Africa, the Middle East, or Southeast Asian countries, studies from Africa reported that up to 15% of patients with suspected pulmonary tuberculosis (TB) and 20% of patients with suspected multidrug-resistant TB may actually have NTM-LD [Citation4]. Studies from the Southeast Asian region and other endemic TB areas found that many patients with NTM-LD had a prior history of TB [Citation27–29].
Irrespective of geography, in-country regional differences in epidemiology of NTM-LD have also been reported [Citation16]. In Japan, for example, annual national NTM-LD prevalence was estimated at 24.9 cases per 100,000 individuals for the entire country; however, regional rates ranged from 13.8 cases per 100,000 individuals in the Tohoku region to 32.5 cases per 100,000 individuals in the Chubu region [Citation16]. Prevalence rates of NTM-LD reported in the UK and EU-4 countries also appear to vary regionally within countries, with one study reporting for the UK, 0.9–7.0; Spain, 3.3–8.4; Italy, 3.8–10.4; France, 1.3–13.6; and Germany, 3.9–8.2 cases per 100,000 individuals [Citation16]. The reasons for these differences are unclear, but it is possible that differences in climate, cultural practices, and healthcare systems may account for epidemiological differences among geographies [Citation16].
Regardless of differences in epidemiology data, the incidence and prevalence of NTM-LD are generally increasing worldwide. In South Korea, one study estimated that NTM infection prevalence estimates increased 5-fold and incidence estimates increased more than 3-fold from 2007 to 2016 [Citation19], and a second study estimated that incidence of NTM-LD increased from 7.0 cases per 100,000 person-years in 2001 to 55.6 cases per 100,000 person-years in 2015 [Citation30]. In Japan, one study estimated that the incidence of NTM-LD increased by 2.6-fold from 2007 to 2014 [Citation31]. In the US, NTM-LD prevalence estimates using a large national managed care claims database increased from 6.78 in 2008 to 11.70 cases per 100,000 individuals in 2015, with an average annual increase in prevalence of 7.5% [Citation32]. In the same time period among Medicare beneficiaries, prevalence estimates increased from 45.6 in 2008 to 70.2 cases per 100,000 individuals in 2015, with an average annual increase of 6.5% [Citation22]. Factors influencing this observed increase in NTM incidence and prevalence may include improved detection methods, a higher level of physician awareness, increased NTM exposure, and greater host susceptibility [Citation33].
Several studies have suggested that MAC is by far the most common NTM species identified, accounting for up to 85% of all NTM-LD cases in the US, up to 82% of cases in Europe, and up to 76.2% of cases in East Asia [Citation4]. Of all MAC isolates, M. avium has been the most frequently isolated MAC species in North America (69%), South America (70%), and Europe (53%) [Citation15]. In Africa and Oceania, M. intracellulare was the most commonly isolated MAC species (77% and 80%, respectively). In Asia, a large proportion of isolates are reported as MAC not further specified (71%), followed by M. avium (16%), and M. intracellulare (13%) [Citation15]. Nevertheless, differences in isolation frequency are also noted routinely, underlining the importance of local situational differences for informing daily clinical practice [Citation15].
4. Patients at risk
NTM-LD is generally a disease of vulnerable populations, such as patients with respiratory comorbidities (e.g. chronic obstructive pulmonary disease [COPD] or bronchiectasis), immunodeficiencies (genetically or acquired due to e.g. oral as well as inhaled corticosteroids, anti-tumor necrosis factor-α therapy, or immunosuppression associated with cancer chemotherapy), or physical characteristics (e.g. connective tissue gene variants, thoracic skeletal abnormalities, and slender body habitus) [Citation3,Citation12,Citation33–37]. Risk of NTM-LD is also increased in the elderly [Citation38]. A history of pulmonary TB has also been reported as an important risk factor for NTM-LD in countries such as France, Denmark and the UK [Citation39–41], as well as in regions with high prevalence of pulmonary TB [Citation27–29]. There is no evidence of human-to-human transmission of MAC.
5. Clinical and humanistic burden
The clinical presentation of NTM-LD is variable, may be nonspecific, and may overlap with other respiratory diseases such as bronchiectasis, COPD, or pulmonary TB [Citation42–44]. NTM-LD commonly presents as the nodular-bronchiectatic form, particularly in patients without underlying lung disease [Citation34]. The fibrocavitary form, which is commonly mistaken for pulmonary TB or malignancy, is often observed in patients with preexisting underlying structural lung diseases and is a more progressive form of the disease [Citation33,Citation34].
The diagnosis of NTM-LD is often missed or delayed; some patients exhibit symptoms for years before a correct diagnosis is made [Citation26,Citation42,Citation44]. For many patients, diagnosis comes after the disease has progressed in severity [Citation45].
Cough, often productive, is the most common symptom experienced by patients with NTM-LD [Citation43,Citation44,Citation46]. Other typical symptoms include fatigue, fever, hemoptysis, shortness of breath, malaise, and night sweats. Patients with NTM-LD reported that bothersome symptoms such as fatigue, coughing up sputum/mucus, and dyspnea negatively impact their quality of life [Citation46]. Typically, these bothersome symptoms have detrimental effects across several quality of life domains, including social functioning (e.g. the patient experiences increased social isolation from others due to embarrassment over symptoms), physical functioning (e.g. the patient has difficulty completing daily tasks), mental health (e.g. the patient feels anxiety due to symptoms), and energy level (e.g. the patient feels fatigue and exhaustion due to symptoms) [Citation46]. Few studies have measured quality of life in patients with NTM-LD using standardized questionnaires [Citation47,Citation48]. Patients with NTM-LD (N = 51; MAC-LD, n = 43) in Canada reported significantly lower health-related quality of life compared with the Canadian general population across all domains measured by the 36-item Short Form Health Survey (SF-36; ) [Citation48]. The scores observed for each SF-36 domain were worse than what was previously reported for patients with common chronic conditions, such as ischemic heart disease, diabetes, arthritis, or COPD [Citation48]. These findings reinforce the clinical impression that NTM-LD has a major impact on health-related quality of life that is at least as severe as several common chronic diseases. Similarly, patients aged 75 years or older with MAC-LD in Japan (N = 84) had significantly lower scores across several domains of the SF-36, including role physical, general health, vitality, social functioning, role-emotional and role-social components, compared with the general Japanese population in the same age category [Citation47].
Figure 2. SF-36 Scores in Patients with NTM-LD Compared with Population Norms in Canada*

Patients with NTM-LD exhibit a progressive decline in lung function as measured by forced expiratory volume in 1 second (FEV1) and forced vital capacity (FVC) [Citation49,Citation50]. Patients with persistently positive NTM cultures have a 3-times higher risk of progressive lung damage compared with patients who have negative cultures [Citation51]. The presence of multiple isolates seems to exacerbate disease in patients with underlying chronic lung diseases associated with progressive decreases in lung function, such as COPD; one study found that lung function decline was over 40% greater among patients with COPD and multiple NTM isolates compared with patients with COPD and no NTM isolates [Citation49]. It has also been reported that patients with NTM-LD had an increased risk of atrial fibrillation (HR, 2.3; P< 0.001) compared with patients without NTM-LD [Citation52].
6. Mortality
A systematic review by Diel et al. reported that most studies in patients with MAC-LD documented a 5-year all-cause mortality exceeding 25%, indicating poor prognosis [Citation53]. Studies predominantly including patients with cavitary disease or greater comorbidity reported a higher risk of death. Patients in Asian studies tended to have lower 5-year mortality (19%, 95% CI 14–23%) compared with those from Europe (35%, 95% CI 27–43%) and North America (33%, 95% CI 32–35%).
Several studies using health claims data have demonstrated the incremental impact of NTM infection on patient all-cause mortality. Overall mortality risk is up to 4-times higher in patients with NTM-LD compared with matched controls, after adjusting for demographic factors and baseline comorbidities () [Citation52,Citation54–56]. Results from a retrospective study in the US found a 4.0-year decrease in expected survival in patients with NTM-LD and no comorbidities compared with the general population; in patients with comorbidities and NTM-LD, there was an 8.6-year decrease in expected survival [Citation57].
Table 1. Mortality Estimates Among Patients with NTM-LD Compared with Matched Controls
A greater awareness of the effect of NTM-LD on mortality is needed, as evidence suggests that physicians may underestimate this risk [Citation58]. A survey of European physicians (N = 280) found that 25% of respondents were unsure about the impact of NTM-LD on mortality, and 13% responded that NTM-LD had no significant impact on mortality [Citation58].
7. Economic burden
Consistent with the medical outcomes, NTM-LD is associated with a substantial increase in healthcare resource use. Patients with NTM-LD are at a 2- to 5-times higher risk of hospitalization compared with matched controls [Citation54,Citation59]. Underlying comorbidities can further increase resource use, with patients with NTM-LD and comorbidities requiring more than twice the number of NTM-LD–related hospitalizations compared with patients without comorbidities [Citation57]. Furthermore, patients with NTM-LD and comorbid respiratory diseases have significantly increased healthcare resource use compared with patients who have respiratory diseases without NTM-LD [Citation60,Citation61]. Results from an analysis of US Medicare claims demonstrated that patients with COPD and NTM-LD and patients with bronchiectasis and NTM-LD had significantly higher disease burden due to hospitalizations and emergency department visits compared with patients with COPD alone and bronchiectasis alone [Citation60,Citation61]. Lastly, results from a study of patients with refractory MAC-LD in Canada, France, Germany, and the UK demonstrated that 70% of patients who experienced pulmonary exacerbations required an emergency department visit and 66% were ultimately hospitalized [Citation62].
Studies in the US and Germany showed that overall healthcare expenditures in the first year after NTM-LD diagnosis were approximately 2.5-times and nearly 4-times greater, respectively, for patients with NTM-LD than for controls [Citation54,Citation59].
8. Management
Successful treatment of NTM-LD requires administration of a multidrug regimen typically including 3 to 5 antibiotics [Citation1]. Patients often receive 18 to 24 months of treatment as guideline recommendations are to continue treatment for 12 months following culture conversion [Citation1]. Patients who continue to have positive sputum cultures after 6 months of guideline-based therapy (GBT) are considered to have refractory disease, as defined by the 2020 NTM clinical practice guidelines developed jointly by the American Thoracic Society (ATS), the European Respiratory Society (ERS), the European Society of Clinical Microbiology and Infectious Diseases (ESCMID), and the Infectious Diseases Society of America (IDSA) (i.e. the 2020 NTM guidelines) [Citation1]. Patients with refractory disease can be treated for several years [Citation13]. Treatment of NTM-LD is complicated by adverse drug effects and suboptimal treatment success rates often associated with currently recommended multidrug regimens [Citation63]. In addition to pharmacological treatment, a holistic approach to NTM-LD care that includes pulmonary rehabilitation and exercise programs to improve exercise capacity and reduce breathlessness, dietary and nutritional management for frail patients, and psychological, social and educational support are important to maintain patient engagement in the long-term treatment program and improve outcomes [Citation64].
Randomized controlled trials (RCTs) to support treatment decisions in NTM-LD are few, and clinical studies attempting to define appropriate algorithms have historically used inconsistent outcome measures [Citation65,Citation66]. However, recent updates to clinical practice guidelines [Citation1], the publication of treatment outcome definitions for NTM-LD based on international expert consensus () [Citation66], the availability of RCT evidence for amikacin liposome inhalation suspension (ALIS) in refractory MAC-LD [Citation67], and ongoing clinical trials of new investigational treatments in MAC-LD [Citation68,Citation69] have moved to close this gap in our understanding of the treatment of patients with NTM-LD.
Table 2. Key Outcomes for Management of NTM-LD, per the NTM-NET Consensus
Key goals of NTM-LD treatment are to cure the disease and improve patient well-being [Citation70]. Achieving ‘microbiological cure’ () [Citation66] is the most important marker of long-term treatment success, as failure to cure the infection can cause further lung damage and worsen patient prognosis [Citation50,Citation70,Citation71]. In some patients where a cure or sustained culture conversion may not be deemed possible, stability or symptomatic improvement may be the primary goal of treatment [Citation70].
Antibiotic treatment for NTM-LD should continue for 12 months after culture conversion, at which point the infection is considered successfully treated if cultures remain negative until the end of treatment [Citation1]. Benefits of successfully treating the infection include improved survival, reduced decline in lung function, and improved quality of life [Citation50,Citation51,Citation71–74]. Several studies have shown that in patients with MAC-LD, overall survival was higher in patients who were successfully treated (i.e. achieved sputum conversion to negative) compared with patients who failed therapy [Citation71–73]. Mortality rates among patients with clarithromycin-resistant MAC-LD who continued to have positive cultures were 34% at 1 year and 45% at 2 years, compared with 1- and 2-year mortality rates of 0% for patients who achieved negative culture conversion [Citation71]. Furthermore, patients who were successfully treated compared with those with persistent positive cultures had 3-times lower odds of progressive lung damage [Citation51] and an over 45% slower decline in lung function, as measured by FEV1 and FVC [Citation50]. In a separate study, patients with successfully treated disease showed improved quality of life as measured by the St. George’s Respiratory Questionnaire [Citation74]. Quality-of-life improvements were noted to last up to 3 years post-treatment in patients with successful treatment; by contrast, quality of life only transiently improved before returning to baseline levels in patients whose disease was not successfully treated [Citation74].
When individuals with NTM-LD fail to achieve culture conversion with the initial line of therapy, or if they revert from a negative to a positive culture during this time, successful treatment with additional rounds of therapy is less likely [Citation75]. Patients who continue to have positive sputum cultures after 6 months of GBT are considered to have refractory disease, per the 2020 NTM guidelines [Citation1]. Other clinical practice guidelines and expert consensus statements define refractory disease and treatment failure using a 12-month timeframe [Citation33,Citation66]; however, a 6-month treatment duration aligns with evidence indicating that a lack of microbiological response after 6 months of initial treatment accurately predicts treatment failure or non-response after at least 12 months of initial treatment [Citation1,Citation76,Citation77]. The 2020 NTM guidelines therefore recommend changing GBT in patients with refractory NTM-LD [Citation1].
9. Treatment recommendations: 2020 ATS/ERS/ESCMID/IDSA guidelines
The 2020 NTM guidelines focus on the NTM species that most commonly cause LD, including MAC [Citation1]. The 2020 NTM guidelines suggest initiating treatment in patients meeting the diagnostic criteria for NTM-LD rather than watchful waiting, especially in the context of positive acid-fast bacilli sputum smears and/or cavitary lung disease [Citation1]. The decision to initiate treatment should nonetheless be individualized based on various factors such as the virulence of the infecting species, the presence of clinical factors associated with a relatively poor prognosis, patient priorities, and the severity of symptoms [Citation1]. The 2020 NTM guidelines do note that watchful waiting may be appropriate for some patients whose infective species is less responsive to treatment and for patients with mild symptoms and/or higher potential for medication intolerance.
In general, drug susceptibility testing is performed for drugs used in treatment regimens and for which there are clear correlations between in vitro activity and the in vivo outcomes of treatment [Citation1]. For patients with MAC-LD, correlation between in vitro drug susceptibility and clinical outcomes has been clearly demonstrated only for macrolides and amikacin [Citation1,Citation5]. Acquired macrolide resistance in MAC is due to point mutations in the 23S rRNA (rrl) gene and the breakpoint is an MIC ≥ 32 μg/mL [Citation1]. Acquired resistance for amikacin is due to resistance conferring mutations in the 16S rRNA (rrs) gene and are largely isolated from patients who had extensive exposure to amikacin and/or related aminoglycosides [Citation1]. For parenteral amikacin, the breakpoint for resistance is an MIC ≥ 64 μg/mL and for ALIS it is ≥128 ug/mL [Citation1]. Drug susceptibility testing of MAC isolates should be performed at a laboratory with significant experience in these techniques, as the reproducibility of these results is lower at inexperienced laboratories [Citation5,Citation65].
The initial treatment recommendation for patients with macrolide-susceptible MAC-LD consists of a 3-drug, macrolide-based regimen – azithromycin (or clarithromycin, although azithromycin is preferred), rifampicin (or rifabutin), and ethambutol – administered for at least 12 months after sputum cultures convert to negative (). A macrolide-based regimen is strongly recommended for MAC-LD in the 2020 NTM guidelines. Furthermore, a 3-drug regimen (containing a macrolide and ethambutol) is preferred over a 2-drug regimen (a macrolide and ethambutol alone) to prevent the development of macrolide resistance. The 2020 NTM guidelines note that macrolide susceptibility is a consistent predictor of treatment success for MAC-LD, with increased rates of culture conversion and lower mortality noted in patients with disease caused by macrolide-susceptible versus resistant MAC isolates.
Table 3. 2020 ATS/ERS/ESCMID/IDSA Recommended Treatment Regimensa for MAC-LD According to Disease Status and/or Severity
For patients with cavitary or advanced/severe bronchiectasis or macrolide-resistant MAC-LD, a multidrug regimen plus either parenteral amikacin or streptomycin is suggested [Citation1]. For patients with refractory MAC-LD (i.e. patients who remain culture positive after 6 months of GBT), the 2020 NTM guidelines strongly recommend the addition of ALIS to the 3-drug, macrolide-based regimen; ‘strong recommendation’ was graded as ‘the recommendation can be adopted as policy in most situations’.
Surgical resection may be considered in selected individuals, particularly in patients with localized pulmonary disease who are refractory to medical therapy or who have macrolide-resistant disease [Citation1]. The decision to proceed with surgical resection must be weighed against the risks and benefits of surgery and surgery should be performed by a surgeon experienced in performing surgery on patients with mycobacterial disease [Citation1].
10. Unmet treatment needs
In patients with MAC-LD, success rates of the initial macrolide-containing regimen remain suboptimal due to frequent adverse drug reactions, drug-drug interactions, and high rates of disease recurrence [Citation63,Citation78]. Approximately 20% to 40% of patients with MAC-LD fail to respond to initial treatment, and an additional 10% to approximately 50% of patients with MAC-LD who are initially treated successfully have a disease recurrence [Citation79–83]. Treatment of MAC-LD in patients who fail to respond to initial therapy or who experience a recurrence is often prolonged and associated with variable efficacy [Citation84–87]. Furthermore, the chance of treatment success with additional rounds of therapy is decreased, even if in vitro macrolide susceptibility is maintained [Citation75]. Treatment options for these patients are limited, with ALIS – in addition to a macrolide-based and guideline-compliant regimen – the only treatment backed by clinical trial evidence and strongly recommended in the NTM guidelines for patients with MAC-LD who failed an initial line of therapy [Citation1]. Other options include intensification of therapy with the addition of a parenteral agent (e.g. amikacin) and, in appropriate cases, surgery [Citation1,Citation78].
Poor adherence and premature discontinuation of antibiotic treatment has also been described [Citation45,Citation88,Citation89]. Reasons contributing to poor adherence may include antibiotic regimen complexity, duration of therapy, and tolerability [Citation88,Citation89]. Additionally, patients with NTM-LD commonly have comorbidities that can contribute to polypharmacy and drug interactions, and physicians may lack experience managing patients with NTM-LD [Citation89]. Poor adherence to the NTM guidelines may have negative consequences on the long-term management of patients with MAC-LD, including the development of macrolide resistance [Citation45,Citation88], whereas adherence to guidelines has been correlated with better treatment outcomes [Citation90]. Avoiding macrolide and amikacin resistance is a high priority in MAC-LD management [Citation78]. Studies consistently show that macrolide-resistant MAC-LD is associated with treatment failure and increased mortality compared with macrolide-susceptible MAC-LD [Citation71,Citation91,Citation92]. Use of inappropriate (i.e. non–guideline recommended) regimens is an additional key risk factor in the development of macrolide-resistant NTM-LD; non–guideline adherent regimens may include macrolide monotherapy, macrolide plus fluoroquinolone (without other companion medications), and macrolide plus rifampicin (without ethambutol) [Citation71,Citation92]. Nonetheless, even among patients who use GBT, prolonged exposure to antibiotic therapy can still lead to the development of macrolide resistance [Citation91,Citation93,Citation94]. One study reported 22% of patients with refractory MAC-LD developed macrolide resistance after a median treatment duration of 33 months [Citation93].
11. Novel treatments for MAC-LD
Considering intrinsic and acquired drug resistance of NTM to a wide range of drugs, new antimicrobials or targeted delivery of currently available antibiotics is needed [Citation95]. Given the ability of MAC to grow biofilms and replicate in intracellular compartments, it is crucial that antibiotics demonstrate effective targeting of bacteria regardless of their location. Poor in vitro-in vivo correlation further contributes to challenges in drug discovery for MAC and other NTM species; therefore, new preclinical assays and models are needed that improve drug candidate evaluation.
ALIS is the first treatment specifically developed for the treatment of patients with MAC-LD with limited treatment options [Citation78,Citation96]. ALIS has been available in the US since late 2018 and is the first Food and Drug Administration–approved treatment for refractory MAC-LD [Citation97]. In October 2020, ALIS received marketing authorization in the EU for the treatment of NTM lung infections caused by MAC in adults with limited treatment options who do not have cystic fibrosis [Citation98]. In March 2021, ALIS was approved for use in Japan for the treatment of NTM-LD caused by MAC in patients who have not been successfully treated with a multidrug regimen.
ALIS is a liposomal formulation of amikacin [Citation97]. Although amikacin is an effective concentration-dependent antibiotic active against MAC in vitro, parenteral or inhaled use of amikacin injection solution havs demonstrated significant limitations [Citation99–103]. Amikacin has a limited ability to cross membranes of mammalian cells [Citation103]. The intracellular localization of MAC in macrophages therefore limits the ability of free amikacin to achieve sufficient antimycobacterial levels inside these infected cells [Citation103]. Additionally, the parenteral use of amikacin solution in patients with MAC-LD is associated with a high frequency of renal, auditory, and vestibular toxicity [Citation100,Citation102].
Due to the significant systemic toxicity associated with parenteral administration of amikacin, physicians have been using nonliposomal amikacin by inhalation in an attempt to improve tolerability and increase effectiveness [Citation63]. Evidence for nonliposomal inhaled amikacin solution is based on only 5 retrospective case series (N = 138 patients, 55 with MAC). The results of these studies are difficult to interpret and compare due to multiple inconsistencies in design and outcome [Citation1]. None of these studies had comparator arms and all reported highly variable outcomes. Lastly, inhalation of aerosolized nonliposomal aminoglycoside solutions is associated with rapid clearance from lung tissue that necessitates frequent, prolonged dosing to achieve sufficient amounts of drug in the lung [Citation99,Citation104].
ALIS is distinguished from amikacin solution for injection by the use of liposomal technology and administration through nebulization, which should reduce amikacin systemic exposure () [Citation105]. Administration of ALIS has been shown to provide both free and liposomal amikacin, expected to target both extracellular bacteria and intracellular NTM [Citation106,Citation107].
Figure 3. Depiction of Amikacin Liposome Inhalation Suspension (ALIS) Liposome Structure Source: Amikacin liposomal inhalation suspension [package insert] 2020 [Citation97]
![Figure 3. Depiction of Amikacin Liposome Inhalation Suspension (ALIS) Liposome Structure Source: Amikacin liposomal inhalation suspension [package insert] 2020 [Citation97]](/cms/asset/d9aa0891-af16-4f68-a58a-70f3bcaf2857/ierx_a_1987891_f0003_oc.jpg)
In vitro and in vivo data demonstrate that ALIS has superior delivery of amikacin into macrophages, airways, and lung tissue compared with non-liposomal amikacin [Citation105]. In an in vitro study, ALIS penetrated MAC biofilms and displayed concentration-dependent killing of MAC [Citation105]. ALIS administration also results in low systemic exposure to amikacin, with less than 10% of an ALIS dose reaching the systemic circulation [Citation108].
The inclusion of ALIS in the 2020 NTM guidelines is based on the results of the pivotal phase 3, randomized, open-label, multicenter CONVERT study [Citation1,Citation67]. The CONVERT study enrolled patients with MAC-LD who were refractory to GBT and had been unsuccessfully treated for several years (mean = 3.94 years) [Citation109]. Patients who received ALIS + GBT compared with GBT alone demonstrated greater sputum culture conversion by month 6 of treatment (29.0% [n/N: 65/224] vs 8.9% [10/112]; P < 0.001), greater sustained culture conversion (i.e. successful treatment of MAC infection) at 12 months of treatment post conversion (18.3% [41/224] vs 2.7% [3/112]; P < 0.0001), and greater durable culture conversion at 3 months off treatment (16.1% [36/224] vs 0% [0/112]; P < 0.0001; ) [Citation67,Citation109]. Among the 75 patients (ALIS + GBT, n = 65 and GBT alone, n = 10) who achieved culture conversion by month 6 of treatment, 55.4% of patients who received ALIS + GBT had durable culture conversion at the 3-month treatment follow-up visit compared with 0% of patients who received GBT alone (P = 0.0017) [Citation109]. The open-label extension study identified no additional safety concerns [Citation110]. Given the lack of response to GBT among patients prior to study enrollment and severity of the disease, results suggest that by adding ALIS to GBT, this difficult-to-treat patient population has a chance for successful treatment of the NTM infection. A post-hoc analysis of the CONVERT study on the number needed to treat (NNT) has been reported. Specifically, for 1 additional patient with refractory MAC-LD to achieve culture conversion by month 6 with ALIS + GBT, the NNT was 5 patients, and to achieve both sustained and durable culture conversion the NNT was 6 patients [Citation111].
Figure 4. Culture Conversion at 6 months, and Sustainability and Durability of Culture Conversion Among Patients Who Completed a Full 12 Months of Post-conversion Treatment in the CONVERT Study

The most common adverse events among ALIS-treated patients in the CONVERT study included dysphonia, cough, hemoptysis, dyspnea, fatigue, diarrhea, nausea, and oropharyngeal pain [Citation67]. A clinical practice research study relying on patient interviews indicated that by using physician-guided measures (e.g. bronchodilator use, oral rinses, and/or temporary dosing adjustments), these adverse events were managed successfully [Citation96]. Adverse events typically associated with systemic aminoglycoside therapy, such as hearing loss and renal function abnormalities, were generally similar between treatment arms in the CONVERT study, with the exception of tinnitus [Citation67]. In a pooled safety analysis of phase 2 and 3 clinical trials evaluating ALIS (ALIS + GBT [N = 404] vs GBT alone [N = 157]), the number needed to harm (NNH) was 28 for ototoxicity, 166 for nephrotoxicity, 40 for neuromuscular effects, and 60 for allergic alveolitis [Citation111]. The NNH analysis, in combination with efficacy (NNT), suggests ALIS offers a clinically meaningful treatment option for patients with difficult-to-treat MAC-LD.
11.1. Investigational approaches in MAC-LD
Several investigational therapies described below are also being evaluated in the treatment of MAC-LD; however, there is currently a paucity of data supporting the use of these therapies. The level of evidence supporting these therapies ranges widely, including ongoing phase 2 trials, retrospective cohort studies, case reports, and/or in vivo and in vitro evidence. No published phase 3 clinical trial results were available to support the efficacy of the investigational therapies, and studies often lack control arms and/or have small patient numbers (e.g. 10 patients or fewer).
Investigational therapies currently being evaluated in clinical trials for the treatment of MAC-LD include inhaled nitric oxide (NO) and clofazimine. Nitric oxide is a powerful vasodilator and has a role in host defense mechanisms against infection at various sites, including the lungs [Citation112]. In vitro research and case reports suggest that inhaled NO could have an effect against NTM, by potentially reducing bacterial load and improving lung function capacity [Citation113–115]. Inhaled NO is being evaluated in a phase 2 trial for treatment of lung infections, including MAC, other NTM infections, and viral infections [Citation69].
A phase 2 study investigating the use of clofazimine monotherapy in patients with MAC-LD is ongoing in the US as of December 2020 [Citation68], and an RCT comparing clofazimine-ethambutol-azithromycin versus rifampicin-ethambutol-azithromycin was recently completed in the Netherlands. In addition to ongoing clinical trials, clofazimine and other investigational agents (e.g. bedaquiline, moxifloxacin, and linezolid) have been evaluated in retrospective cohort studies and case series. Clofazimine, which in vitro is active against MAC and is synergistic with macrolides and amikacin [Citation116,Citation117], was effective for the treatment of MAC-LD in several cohort studies; however, side effects (e.g. dermatologic, gastrointestinal) have limited its use to select groups of patients [Citation63,Citation84,Citation86,Citation118]. Clofazimine is also important as a replacement drug in case of rifampicin or ethambutol intolerance [Citation119]. Results from a study conducted in NTM-infected mice demonstrated that inhaled clofazimine improved bacterial elimination from the lungs of mice compared with negative controls and oral clofazimine (P < 0.05); however, these results are not confirmed in human studies [Citation120].
Preliminary results from a small (N = 10; MAC-LD, n = 6) case series suggest that bedaquiline may show an initial effect on symptoms and bacterial load in patients with refractory MAC-LD [Citation87]. However, a follow-up study and an individual case report show that treatment failure is common due to acquired bedaquiline resistance [Citation121,Citation122]. In vitro evidence suggests that bedaquiline has moderate concentration-dependent bacteriostatic activity against MAC, with MICs between <0.007 and 1 mg/L [Citation123]. The combination of bedaquiline and clofazimine in vitro was associated with more potent activity against M. avium, and it slowed the emergence of bedaquiline resistance [Citation123]. Larger studies are therefore needed to further evaluate the potential use of bedaquiline in patients with refractory MAC-LD [Citation63].
Results from an antimicrobial susceptibility testing trial suggest moxifloxacin is inactive against MAC isolates, with most isolates testing intermediate or completely resistant [Citation124]. Additionally, results from a retrospective study (N = 41) suggest an increase in clarithromycin- and moxifloxacin-resistant MAC strains in patients who failed moxifloxacin therapy [Citation125]. Results from this study should be evaluated cautiously due to the potential for selection bias, as the addition of moxifloxacin was based on the decision of the attending physician without an established institutional protocol, concurrent use of streptomycin or resection surgery, and limited sample size [Citation125].
Results from a retrospective cohort study evaluating a linezolid-containing regimen (N = 102; 78% with lung disease, 33% with MAC isolates) showed that adverse events necessitating drug discontinuation occurred in more than 40% of patients; the retrospective and nonrandomized design of this study limits the ability to make conclusions regarding the tolerability of linezolid in the context of multidrug NTM therapy [Citation126]. In vitro results also suggest that linezolid has poor activity against MAC [Citation124].
Evidence for the following investigational treatments for MAC-LD is largely limited to case reports and in vitro evidence. In vitro data suggest that minocycline has activity against some MAC strains and was very active in the hollow fiber model of MAC infection [Citation127]; whether these in vitro results will translate to clinical benefit is not known. In vitro evidence suggests tedizolid has concentration-dependent activity against M. avium, and results from a case report demonstrated that tedizolid was used as an alternative treatment in a patient who was diagnosed with NTM-LD caused by MAC and M. kansasii and experienced intolerance to linezolid [Citation128,Citation129]. Other treatment modalities that are being investigated in NTM-LD, with very limited data specifically in MAC-LD, include non-antibiotic interventions such as immunomodulation, hypertonic saline inhalation and other airway-clearance methods, omadacycline, inhaled tigecycline, and mycobacteriophages [Citation130–135].
12. Conclusion
NTM-LD is a growing health concern, with MAC accounting for the majority of infections. Even as NTM-LD is associated with a substantial clinical, humanistic, and economic burden, the treatment of MAC-LD presents therapeutic challenges including the need for prolonged multidrug regimens and high rates of treatment failure. Treatments are often associated with poor tolerability and the need for frequent regimen changes. Patients with MAC-LD who fail first-line therapy have had few treatment options in the past. Recent updates to clinical practice guidelines, publication of RCT evidence supporting the efficacy and safety of ALIS in refractory MAC-LD, and approval of ALIS as the first therapy specifically developed for MAC-LD with limited treatment options, as well as ongoing clinical trials of investigational treatments in MAC-LD, demonstrate significant advancements in the treatment of this disease.
13. Expert opinion
Since 2015, several advancements have been made in the management of NTM-LD. Nonetheless, many areas still require attention to improve patient outcomes and address unmet needs faced by patients and clinicians:
Earlier diagnosis: Increased testing is needed in patients at high risk for NTM-LD through greater awareness of NTM among primary care physicians and use of tools to help with screening. A multidisciplinary approach to patient care should be adopted. When primary care physicians recognize patients with suspected NTM-LD, patients should be referred to and have access to pulmonologists and infectious disease physicians.
Role of biomarkers: There is a lack of reliable and responsive biomarkers to assess disease activity, progression, and response to therapy. Although additional research is needed, semiquantitative sputum culture results [Citation76] and serological markers [Citation136] have been evaluated and have shown potential utility.
Standards of care: Close cooperation between expert centers, healthcare providers, and empowered patients is crucial to improve outcomes. The NTM community should thus come together to define minimal and best possible standards of care. For example, consensus is needed on the tests and order of completion of the tests to best guide diagnosis and treatments, monitoring of adverse events for different treatments, approaches to measuring and improving patient compliance, optimization of antibiotic doses, and measurement of antibiotic concentrations in blood for therapeutic drug monitoring.
Patient voice: Hurdles in healthcare provision as perceived by patients should be explored to address the key gaps and needs of patients [Citation137].
Patient-reported outcome measures (PROMs): Development of valid, reliable, and responsive disease-specific PROMs is needed to help determine patient burden, disease severity, progression, and response to treatment. PROMs may also predict prognosis and have the potential to help assess treatment effectiveness.
Patient registries: The development of global NTM disease registries and crosslinking of existing regional registries will enable a better understanding of the epidemiology, natural history of disease, and real-world management and outcomes of NTM-LD, including MAC-LD.
Research priorities to improve therapy: The clinical research community should focus its attention on enrolling patients with NTM-LD in prospective, comparative studies with objective measurements. Consistent use of standard NTM-NET outcome definitions across prospective studies should be encouraged, given that the use of standard outcomes may streamline reporting and allow researchers to conduct meta-analyses comparing treatments. Additionally, preclinical evaluation of therapies should be improved by systematic validation and application of (animal) models, use of the same reference NTM strains, and publication of model validations.
Rational regimen design: Drug and regimen developers and scientists need to use principles of rational regimen design to exploit activity and synergy, prevent drug-drug interactions (pharmacokinetic interactions), and prevent overlapping toxicities to build better treatment regimens for NTM-LD. The current MAC regimen (with 2 drugs with poor in vitro activity [i.e. rifampicin and ethambutol] and a drug that has negative pharmacokinetic interactions with macrolides [i.e. rifampicin]) is not ideal, and the role of rifampicin is unclear.
Therapeutic advances: The first drug specifically developed for patients with MAC-LD with limited treatment options has been approved in the US, EU, and Japan, and few agents such as clofazimine and inhaled nitric oxide are awaiting evaluation within RCTs. The increased interest and efforts observed to develop new, more efficacious, and tolerable treatments for MAC-LD offer prospects of improved outcomes in this difficult-to-manage disease.
Article highlights
• NTM-LD is a growing health concern that generally occurs in vulnerable populations, such as patients with respiratory comorbidities or immunodeficiencies.
• Patients who develop NTM-LD may experience decreased lung function and quality of life and increased mortality and healthcare expenses compared with patients without the disease.
• MAC-LD is difficult to treat. Patients can receive 18 to 24 months of a multidrug antibiotic regimen consisting of 3 to 5 drugs, adverse events are common, and success rates are suboptimal.
• Recent advancements in the treatment of MAC-LD include approval of amikacin liposome inhalation suspension (ALIS) by the United States Food and Drug Administration, the European Commission in the EU, and the Pharmaceuticals and Medical Devices Agency in Japan. ALIS is the first drug specifically developed for patients with MAC-LD and limited treatment options.
• Additional therapies for MAC-LD are being evaluated in clinical trials. Studies evaluating innovative treatment strategies are needed to address this unmet treatment need.
Declaration of interest
J. van Ingen has been a member of advisory boards for Insmed, Paratek, Janssen Pharmaceuticals, and Spero Therapeutics. He heads one of the trial laboratories active in clinical trials sponsored by Insmed Incorporated. M. Obradovic is an employee of Insmed Incorporated. B. Lesher is an employee of OPEN Health, which received funding from Insmed Incorporated for the current work. E.M. Hart is an employee of OPEN Health, which received funding from Insmed Incorporated for the current work. M. Hassan is an employee of Insmed Incorporated. A. Chatterjee is an employee of Insmed Incorporated. C.L. Daley has been a member of advisory boards for Insmed, Paratek, Spero, Matinas, Cipla, and AN2. He has received research grants from BugWorks, Insmed, Spero, and Paratek.
The authors have no other relevant affiliations or financial involvement with any organization or entity with a financial interest in or financial conflict with the subject matter or materials discussed in the manuscript apart from those disclosed.
Reviewer disclosures
Peer reviewers on this manuscript have no relevant financial or other relationships to disclose.
Acknowledgments
Editorial support was provided by Christina DuVernay and Catherine Mirvis from OPEN Health (Bethesda, MD) and funded by the study sponsor.
Additional information
Funding
References
- Daley CL, Iaccarino JM Jr, and Lange C, et al. Treatment of nontuberculous mycobacterial pulmonary disease: an official ATS/ERS/ESCMID/IDSA clinical practice guideline: executive summary. Clin Infect Dis. 2020; 71(4):e1–e36.
- Jeon D. Infection source and epidemiology of nontuberculous mycobacterial lung disease. Tuberc Respir Dis (Seoul). 2019;82(2):94–101.
- Ratnatunga CN, Lutzky VP, Kupz A, et al. The rise of non-tuberculosis mycobacterial lung disease. Front Immunol. 2020;11:303.
- Prevots DR, Marras TK. Epidemiology of human pulmonary infection with nontuberculous mycobacteria: a review. Clin Chest Med. 2015 Mar;36(1):13–34.
- van Ingen J, Kuijper EJ. Drug susceptibility testing of nontuberculous mycobacteria. Future Microbiol. 2014;9(9):1095–1110.
- Wu ML, Aziz DB, Dartois V, et al. NTM drug discovery: status, gaps and the way forward. Drug Discov Today. 2018;23(8):1502–1519.
- Bermudez LE, Goodman J. Mycobacterium tuberculosis invades and replicates within type II alveolar cells. Infect Immun. 1996;64(4):1400–1406.
- Ganbat D, Seehase S, Richter E, et al. Mycobacteria infect different cell types in the human lung and cause species dependent cellular changes in infected cells. BMC Pulm Med. 2016;16(1):19.
- Busatto C, Vianna JS, Lvj DS, et al. Mycobacterium avium: an overview. Tuberculosis (Edinb). 2019;114:127–134.
- Falkinham JO. Challenges of NTM drug development. Front Microbiol. 2018;9:1613.
- Kim S-Y, Han SA, Kim DH, et al. Nontuberculous mycobacterial lung disease: ecology, microbiology, pathogenesis, and antibiotic resistance mechanisms. Precis Future Med. 2017 9;1(3):99–114.
- Honda JR, Knight V, Chan ED, et al. Pathogenesis and risk factors for nontuberculous mycobacterial lung disease. Clin Chest Med. 2015;36(1):1–11.
- Philley JV, DeGroote MA, Honda JR, et al. Treatment of non-tuberculous mycobacterial lung disease. Curr Treat Options Infect Dis. 2016;8(4):275–296.
- van Ingen J, Turenne CY, Tortoli E, et al. A definition of the mycobacterium avium complex for taxonomical and clinical purposes, a review. Int J Syst Evol Microbiol. 2018 Nov;68(11):3666–3677.
- Zweijpfenning SMH, van Ingen J, Hoefsloot W, et al. Geographic distribution of nontuberculous mycobacteria isolated from clinical specimens: a systematic review. Semin Respir Crit Care Med. 2018;39(3):336–342.
- Schildkraut JA, Gallagher J, Morimoto K, et al. Epidemiology of nontuberculous mycobacterial pulmonary disease in Europe and Japan by Delphi estimation. Respir Med. 2020:106164. DOI:https://doi.org/10.1016/j.rmed.2020.106164
- Adjemian J, Daniel-Wayman S, and Ricotta E, et al. Epidemiology of nontuberculous mycobacteriosis. Semin Respir Crit Care Med. 2018;39(3):325–335.
- Izumi K, Morimoto K, Hasegawa N, et al. Epidemiology of adults and children treated for nontuberculous mycobacterial pulmonary disease in Japan. Ann Am Thorac Soc. 2019;16(3):341–347.
- Lee H, Myung W, Koh WJ, et al. Epidemiology of nontuberculous mycobacterial infection, South Korea, 2007-2016. Emerg Infect Dis. 2019;25(3):569–572.
- Morimoto K, Hasegawa N, Izumi K, et al. A laboratory-based analysis of nontuberculous mycobacterial lung disease in Japan from 2012 to 2013. Ann Am Thorac Soc. 2017;14(1):49–56.
- Park SC, Kang MJ, Han CH, et al. Prevalence, incidence, and mortality of nontuberculous mycobacterial infection in Korea: a nationwide population-based study. BMC Pulm Med. 2019;19(1):140.
- Winthrop KL, Adjemian J, Mirsaeidi M, et al. Incidence and prevalence of nontuberculous mycobacterial lung disease in US medicare beneficiaries, 2008–2015. IDWEEK 2018; Oct 3–7; San Francisco, CA; 2018.
- Adjemian J, Frankland TB, Daida YG, et al. Epidemiology of nontuberculous mycobacterial lung disease and tuberculosis, Hawaii, USA. Emerg Infect Dis. 2017;23(3):439–447.
- Schildkraut JA, Zweijpfenning SMH, Nap M, et al. The epidemiology of nontuberculous mycobacterial pulmonary disease in the Netherlands. ERJ Open Res. 2021 Jul;7(3). DOI:https://doi.org/10.1183/23120541.00207-2021
- Hermansen TS, Ravn P, Svensson E, et al. Nontuberculous mycobacteria in Denmark, incidence and clinical importance during the last quarter-century. Sci Rep. 2017 Jul 27;7(1):6696.
- Doyle OM, van der Laan R, Obradovic M, et al. Identification of potentially undiagnosed patients with nontuberculous mycobacterial lung disease using machine learning applied to primary care data in the UK. Eur Respir J. 2020;56(4):2000045.
- Fusco Da Costa AR, Falkinham JO 3rd, Lopes ML, et al. Occurrence of nontuberculous mycobacterial pulmonary infection in an endemic area of tuberculosis. PLoS Negl Trop Dis. 2013;7(7):e2340.
- Lim AYH, Chotirmall SH, Fok ETK, et al. Profiling non-tuberculous mycobacteria in an Asian setting: characteristics and clinical outcomes of hospitalized patients in Singapore. BMC Pulm Med. 2018 May 22;18(1):85.
- Ahmed I, Tiberi S, Farooqi J, et al. Non-tuberculous mycobacterial infections-A neglected and emerging problem. Int J Infect Dis. 2020 Mar;92s:S46–s50.
- Ko RE, Moon SM, Ahn S, et al. Changing epidemiology of nontuberculous mycobacterial lung diseases in a tertiary referral hospital in Korea between 2001 and 2015. J Korean Med Sci. 2018;33(8):e65–e65.
- Namkoong H, Kurashima A, Morimoto K, et al. Epidemiology of pulmonary nontuberculous mycobacterial disease, Japan. Emerg Infect Dis. 2016;22(6):1116–1117.
- Winthrop KL, Marras TK, Adjemian J, et al. Incidence and prevalence of nontuberculous mycobacterial lung disease in a large U.S. managed care health plan, 2008-2015. Ann Am Thorac Soc. 2020;17(2):178–185.
- Haworth CS, Banks J, Capstick T, et al. British thoracic society guidelines for the management of non-tuberculous mycobacterial pulmonary disease (NTM-PD). Thorax. 2017;72(Suppl 2):ii1–ii64.
- Larsson LO, Polverino E, Hoefsloot W, et al. Pulmonary disease by non-tuberculous mycobacteria - clinical management, unmet needs and future perspectives. Expert Rev Respir Med. 2017 Dec;11(12):977–989.
- Bruno M, Zweijpfenning SMH, and Verhoeven J, et al. Subtle immunodeficiencies in nodular-bronchiectatic mycobacterium avium complex lung disease. ERJ Open Res. 2020;6(4):00548–2020.
- Honda JR, Alper S, Bai X, et al. Acquired and genetic host susceptibility factors and microbial pathogenic factors that predispose to nontuberculous mycobacterial infections. Curr Opin Immunol. 2018 Oct;54:66–73.
- Wu UI, Holland SM. Host susceptibility to non-tuberculous mycobacterial infections. Lancet Infect Dis. 2015 Aug;15(8):968–980.
- Mirsaeidi M, Farshidpour M, Ebrahimi G, et al. Management of nontuberculous mycobacterial infection in the elderly. Eur J Intern Med. 2014;25(4):356–363.
- Andrejak C, Chiron R, Veziris N, et al. PIN57 burden of non-tuberculous mycobacterial lung disease (NTMLD) in France: a claim database analysis [conference abstract]. Value Health. 2020;23:S554.
- Andréjak C, Nielsen R, Thomsen V, et al. Chronic respiratory disease, inhaled corticosteroids and risk of non-tuberculous mycobacteriosis. Thorax. 2013 Mar;68(3):256–262.
- Axson EL, Bual N, Bloom CI, et al. Risk factors and secondary care utilisation in a primary care population with non-tuberculous mycobacterial disease in the UK. Eur J Clin Microbiol Infect Dis. 2019 Jan;38(1):117–124.
- Khan Z, Miller A, Bachan M, et al. Mycobacterium avium complex (MAC) lung disease in two inner city community hospitals: recognition, prevalence, co-infection with mycobacterium tuberculosis (MTB) and pulmonary function (PF) improvements after treatment. Open Respir Med J. 2010;4:76–81.
- Kim RD, Greenberg DE, Ehrmantraut ME, et al. Pulmonary nontuberculous mycobacterial disease: prospective study of a distinct preexisting syndrome. Am J Respir Crit Care Med. 2008;178(10):1066–1074.
- Kotilainen H, Valtonen V, Tukiainen P, et al. Clinical findings in relation to mortality in non-tuberculous mycobacterial infections: patients with mycobacterium avium complex have better survival than patients with other mycobacteria. Eur J Clin Microbiol Infect Dis. 2015 Sep;34(9):1909–1918.
- van Ingen J, Wagner D, Gallagher J, et al. Poor adherence to management guidelines in nontuberculous mycobacterial pulmonary diseases. Eur Respir J. 2017;49(2):1601855.
- Leitman A. NTM lung disease: patient experiences & preferences. Presented at FDA public workshop: Development of antibacterial drugs for treatment of nontuberculous mycobacterial disease. Silver Spring, MD; 2019 [ cited 2020 Aug 3]. Available from: https://www.fda.gov/media/124058/download
- Asakura T, Ishii M, Ishii K, et al. Health-related QOL of elderly patients with pulmonary M. avium complex disease in a university hospital. Int J Tuberc Lung Dis. 2018;22(6):695–703.
- Mehta M, Marras TK. Impaired health-related quality of life in pulmonary nontuberculous mycobacterial disease. Respir Med. 2011;105(11):1718–1725.
- Huang CT, Tsai YJ, Wu HD, et al. Impact of non-tuberculous mycobacteria on pulmonary function decline in chronic obstructive pulmonary disease. Int J Tuberc Lung Dis. 2012;16(4):539–545.
- Park HY, Jeong BH, Chon HR, et al. Lung function decline according to clinical course in nontuberculous mycobacterial lung disease. Chest. 2016;150(6):1222–1232.
- Pan SW, Shu CC, Feng JY, et al. Microbiological persistence in patients with mycobacterium avium complex lung disease: the predictors and the impact on radiographic progression. Clin Infect Dis. 2017;65(6):927–934.
- Park CS, Choi E-K, Kim B, et al. Association between atrial fibrillation, myocardial infarction, heart failure and mortality in patients with nontuberculous mycobacterial infection: a nationwide population-based study. Sci Rep. 2019;9(1):15503.
- Diel R, Lipman M, Hoefsloot W, et al. High mortality in patients with mycobacterium avium complex lung disease: a systematic review. BMC Infect Dis. 2018 May 3;18(1):206.
- Diel R, Jacob J, Lampenius N, et al. Burden of non-tuberculous mycobacterial pulmonary disease in Germany. Eur Respir J. 2017;49(4):1602109.
- Marras TK, Campitelli MA, Lu H, et al. Pulmonary nontuberculous mycobacteria-associated deaths, Ontario, Canada, 2001-2013. Emerg Infect Dis. 2017;23(3):468–476.
- Marras TK, Vinnard C, Zhang Q, et al. Relative risk of all-cause mortality in patients with nontuberculous mycobacterial lung disease in a US managed care population. Respir Med. 2018;145:80–88.
- Mourad A, Baker AW, and Stout JE, et al. Reduction in expected survival associated with nontuberculous mycobacterial pulmonary disease. Clin Infect Dis. 2021; 72(10)2020:e552–e557.
- Wagner D, van Ingen J, van der Laan R, et al. Non-tuberculous mycobacterial lung disease in patients with bronchiectasis: perceived risk, severity and guideline adherence in a European physician survey. BMJ Open Respir Res. 2020;7(1). DOI:https://doi.org/10.1136/bmjresp-2019-000498
- Marras TK, Mirsaeidi M, Chou E, et al. Health care utilization and expenditures following diagnosis of nontuberculous mycobacterial lung disease in the United States. J Manag Care Spec Pharm. 2018;24(10):964–974.
- Wang P, Hassan M, and Chatterjee A, et al. The incremental burden of nontuberculous mycobacterial lung disease (NTMLD) among patients with chronic obstructive pulmonary disease (COPD): hospitalizations and ER visits among US medicare beneficiaries. Poster presented at: IDWEEK 2020 [virtual meeting]; 2020 Oct 21.
- Wang P, Marras TK, and Alemao E, et al. The incremental burden of nontuberculous mycobacterial lung disease (NTMLD) among patients with bronchiectasis (BE): hospitalizations and ER visits among US medicare beneficiaries. Poster presented at: WBC 2020 [virtual meeting]; 2020 Dec 18.
- Goring SM, Wilson JB, Risebrough NR, et al. The cost of mycobacterium avium complex lung disease in Canada, France, Germany, and the United Kingdom: a nationally representative observational study. BMC Health Serv Res. 2018;18(1):700.
- Kwon YS, Koh WJ, Daley CL, et al. Treatment of mycobacterium avium complex pulmonary disease. Tuberc Respir Dis (Seoul). 2019;82(1):15–26.
- Ali J. A multidisciplinary approach to the management of nontuberculous mycobacterial lung disease: a clinical perspective. Expert Rev Respir Med. 2021 May;15(5):663–673.
- Daley CL. Mycobacterium avium complex disease. Microbiol Spectr. 2017;5(2). DOI:https://doi.org/10.1128/microbiolspec.TNMI7-0045-2017
- van Ingen J, Aksamit T, and Andrejak C, et al. Treatment outcome definitions in nontuberculous mycobacterial pulmonary disease: an NTM-NET consensus statement. Eur Respir J. 2018;51(3):1800170.
- Griffith D, Eagle G, and Thomson RM, et al. Amikacin liposome inhalation suspension for treatment-refractory lung disease caused by mycobacterium avium complex (CONVERT). A prospective, open-label, randomized study. Am J Respir Crit Care Med. 2018;198(12):1559–1569.
- ClinicalTrials.gov. NCT02968212. Clofazimine in the treatment of pulmonary mycobacterium avium complex (MAC). 2020 [ cited 2020 Jul 31]. Available from: https://clinicaltrials.gov/ct2/show/NCT02968212
- ClinicalTrials.gov. NCT03331445. Inhaled gaseous nitric oxide (gNO) antimicrobial treatment of difficult bacterial and viral lung (COVID-19) infections (NONTM). 2020 [ cited 2020 Aug 13]. Available from: https://clinicaltrials.gov/ct2/show/NCT03331445
- Loebinger MR, Birring SS. Patient reported outcomes for non-tuberculous mycobacterial disease. Eur Respir J. 2020;55(1):1902204.
- Griffith DE, Brown-Elliott BA, Langsjoen B, et al. Clinical and molecular analysis of macrolide resistance in mycobacterium avium complex lung disease. Am J Respir Crit Care Med. 2006;174(8):928–934.
- Ito Y, Hirai T, Maekawa K, et al. Predictors of 5-year mortality in pulmonary mycobacterium avium-intracellulare complex disease. Int J Tuberc Lung Dis. 2012;16(3):408–414.
- Jenkins PA, Campbell IA, Banks J, et al. Clarithromycin vs ciprofloxacin as adjuncts to rifampicin and ethambutol in treating opportunist mycobacterial lung diseases and an assessment of mycobacterium vaccae immunotherapy. Thorax. 2008;63(7):627–634.
- Kwak N, Kim SA, Choi SM, et al. Longitudinal changes in health-related quality of life according to clinical course among patients with non-tuberculous mycobacterial pulmonary disease: a prospective cohort study. BMC Pulm Med. 2020;20(1):126.
- Griffith DE, Aksamit TR. Therapy of refractory nontuberculous mycobacterial lung disease. Curr Opin Infect Dis. 2012;25(2):218–227.
- Griffith DE, Adjemian J, Brown-Elliott BA, et al. Semiquantitative culture analysis during therapy for mycobacterium avium complex lung disease. Am J Respir Crit Care Med. 2015;192(6):754–760.
- Moon SM, Jhun BW, Daley CL, et al. Unresolved issues in treatment outcome definitions for nontuberculous mycobacterial pulmonary disease. Eur Respir J. 2019;53(5). DOI:https://doi.org/10.1183/13993003.01636-2018
- Griffith DE, Aksamit TR. Managing M. avium complex lung disease with a little help from my friend. Chest. 2020;159(4):1372–1381.
- Boyle DP, Zembower TR, Qi C, et al. Relapse versus reinfection of mycobacterium avium complex pulmonary disease. Patient characteristics and macrolide susceptibility. Ann Am Thorac Soc. 2016;13(11):1956–1961.
- Diel R, Nienhaus A, Ringshausen FC, et al. Microbiologic outcome of interventions against mycobacterium avium complex pulmonary disease: a systematic review. Chest. 2018;153(4):888–921.
- Lee BY, Kim S, Hong Y, et al. Risk factors for recurrence after successful treatment of mycobacterium avium complex lung disease. Antimicrob Agents Chemother. 2015;59(6):2972–2977.
- Wallace RJ Jr., Brown-Elliott BA, McNulty S, et al. Macrolide/Azalide therapy for nodular/bronchiectatic mycobacterium avium complex lung disease. Chest. 2014 Aug;146(2):276–282.
- Koh WJ, Moon SM, Kim SY, et al. Outcomes of mycobacterium avium complex lung disease based on clinical phenotype. Eur Respir J. 2017 Sep;50(3). DOI:https://doi.org/10.1183/13993003.02503-2016
- Jarand J, Davis JP, Cowie RL, et al. Long-term follow-up of mycobacterium avium complex lung disease in patients treated with regimens including clofazimine and/or rifampin. Chest. 2016;149(5):1285–1293.
- Jo KW, Kim S, Lee JY, et al. Treatment outcomes of refractory MAC pulmonary disease treated with drugs with unclear efficacy. J Infect Chemother. 2014;20(10):602–606.
- Martiniano SL, Wagner BD, Levin A, et al. Safety and effectiveness of clofazimine for primary and refractory nontuberculous mycobacterial infection. Chest. 2017;152(4):800–809.
- Philley JV, Wallace RJ Jr., Benwill JL, et al. Preliminary results of bedaquiline as salvage therapy for patients with nontuberculous mycobacterial lung disease. Chest. 2015;148(2):499–506.
- Adjemian J, Prevots DR, Gallagher J, et al. Lack of adherence to evidence-based treatment guidelines for nontuberculous mycobacterial lung disease. Ann Am Thorac Soc. 2014;11(1):9–16.
- Diel R, Obradovic M, Tyler S, et al. Real-world treatment patterns in patients with nontuberculous mycobacterial lung disease in general and pneumologist practices in Germany. J Clin Tuberc Other Mycobact. 2020; 20:100178.
- Abate G, Stapleton JT, and Rouphael N, et al. Variability in the management of adults with pulmonary nontuberculous mycobacterial disease. Clin Infect Dis. 2021 72(7): 1127–1137 .
- Moon SM, Park HY, Kim SY, et al. Clinical characteristics, treatment outcomes, and resistance mutations associated with macrolide-resistant mycobacterium avium complex lung disease. Antimicrob Agents Chemother. 2016;60(11):6758–6765.
- Morimoto K, Namkoong H, Hasegawa N, et al. Macrolide-resistant mycobacterium avium complex lung disease: analysis of 102 consecutive cases. Ann Am Thorac Soc. 2016;13(11):1904–1911.
- Jhun BW, Kim SY, Moon SM, et al. Development of macrolide resistance and reinfection in refractory mycobacterium avium complex lung disease. Am J Respir Crit Care Med. 2018;198(10):1322–1330.
- Kadota T, Matsui H, Hirose T, et al. Analysis of drug treatment outcome in clarithromycin-resistant mycobacterium avium complex lung disease. BMC Infect Dis. 2016;16:31.
- Chalmers JD, van Ingen J, van der Laan R, et al. Liposomal drug delivery to manage nontuberculous mycobacterial pulmonary disease and other chronic lung infections. Eur Respir Rev. 2021 Sep 30;30(161). DOI:https://doi.org/10.1183/16000617.0010-2021
- Swenson C, Lapinel NC, Ali J, et al. Clinical management of respiratory adverse events associated with amikacin liposome inhalation suspension: results from a patient survey. Open Forum Infect Dis. 2020;7(4):ofaa079.
- Amikacin liposomal inhalation suspension [package insert]. Bridgewater, NJ: Insmed Incorporated; 2020.
- European Medicines Agency. Arikayce liposomal. 2020 [ cited 2020 Jul 31]. Available from: https://www.ema.europa.eu/en/medicines/human/summaries-opinion/arikayce-liposomal
- Geller DE, Pitlick WH, Nardella PA, et al. Pharmacokinetics and bioavailability of aerosolized tobramycin in cystic fibrosis. Chest. 2002;122(1):219–226.
- Jhun BW, Yang B, Moon SM, et al. Amikacin inhalation as salvage therapy for refractory nontuberculous mycobacterial lung disease. Antimicrob Agents Chemother. 2018;62(7). DOI:https://doi.org/10.1128/AAC.00011-18
- Molina-Torres CA, Tamez-Peña L, Castro-Garza J, et al. Evaluation of the intracellular activity of drugs against mycobacterium abscessus using a THP-1 macrophage model. J Microbiol Methods. 2018;148:29–32.
- Peloquin CA, Berning SE, Nitta AT, et al. Aminoglycoside toxicity: daily versus thrice-weekly dosing for treatment of mycobacterial diseases. Clin Infect Dis. 2004;38(11):1538–1544.
- van Ingen J, Egelund EF, Levin A, et al. The pharmacokinetics and pharmacodynamics of pulmonary mycobacterium avium complex disease treatment. Am J Respir Crit Care Med. 2012;186(6):559–565.
- Waters V, Ratjen F. Inhaled liposomal amikacin. Expert Rev Respir Med. 2014;8(4):401–409.
- Zhang J, Leifer F, Rose S, et al. Amikacin liposome inhalation suspension (ALIS) penetrates non-tuberculous mycobacterial biofilms and enhances amikacin uptake into macrophages. Front Microbiol. 2018;9:915.
- Li Z, Zhang Y, Wurtz W, et al. Characterization of nebulized liposomal amikacin (Arikace) as a function of droplet size. J Aerosol Med Pulm Drug Deliv. 2008;21(3):245–254.
- Rose SJ, Neville ME, Gupta R, et al. Delivery of aerosolized liposomal amikacin as a novel approach for the treatment of nontuberculous mycobacteria in an experimental model of pulmonary infection. PLoS One. 2014;9(9):e108703.
- Rubino C, Onufrak N, van Ingen J, et al. Population pharmacokinetic evaluation of amikacin liposome inhalation suspension in patients with treatment-refractory nontuberculous mycobacterial lung disease. Eur J Drug Metab. 2021;46(2):277–287.
- Griffith DE, Thomson R, and Flume PA, et al. Amikacin liposome inhalation suspension for refractory MAC lung disease: sustainability and durability of culture conversion and safety of long-term exposure. Chest 2021 160 3 . 2021:831–842.
- Winthrop KL, Flume PA, Thomson R, et al. Amikacin liposome inhalation suspension for MAC lung disease: a 12-month open-label extension study. Ann Am Thorac Soc. 2021;18(7):1147–1157.
- Marras TK, Hassan M, and Ciesielska M, et al. ALIS (amikacin liposome inhalation suspension) for the treatment of patients with refractory mycobacterium avium complex lung disease (MACLD): the number needed to treat (NNT) and number needed to harm (NNH). Oral presentation at the American Thoracic Society (ATS) Conference 2021 [virtual meeting].
- Bogdan C. Nitric oxide and the immune response. Nat Immunol. 2001 Oct;2(10):907–916.
- Ghaffari A, Da Silva JL, Bogdanovski K, et al. High-dose nitric oxide as a potential new therapeutic agent against mycobacterium abscessus. Eur Respir J. 2018;52(suppl 62):PA5463.
- Bogdanovski K, Chau T, Robinson CJ, et al. Antibacterial activity of high-dose nitric oxide against pulmonary mycobacterium abscessus disease. Access Microbiol. 2020;2(9):acmi000154.
- Bentur L, Masarweh K, Livnat-Levanon G, et al. Nitric oxide inhalations in cf patients infected with mycobacterium abscessus complex: a prospective, open-labeled, multi-center pilot study [conference abstract]. Am J Respir Crit Care Med; 2018. p. 197. (MeetingAbstracts).
- Ferro BE, Meletiadis J, and Wattenberg M, et al. Clofazimine prevents the regrowth of mycobacterium abscessus and mycobacterium avium type strains exposed to amikacin and clarithromycin. Antimicrob Agents Chemother. 2015;60(2) ;1097–105.
- van Ingen J, Totten SE, Helstrom NK, et al. In vitro synergy between clofazimine and amikacin in treatment of nontuberculous mycobacterial disease. Antimicrob Agents Chemother. 2012;56(12):6324–6327.
- Yang B, Jhun BW, and Moon SM, et al. A clofazimine-containing regimen for the treatment of mycobacterium abscessus lung disease. Antimicrob Agents Chemother. 2017;61(6) ;e02052–16.
- van Ingen J, Aliberti S, and Andrejak C, et al. Management of drug toxicity in Mycobacterium avium complex pulmonary disease: an expert panel survey. Clin Infect Dis. 2021;73(1):2020:e256–e259.
- Banaschewski B, Verma D, Pennings LJ, et al. Clofazimine inhalation suspension for the aerosol treatment of pulmonary nontuberculous mycobacterial infections. J Cyst Fibros. 2019;18(5):714–720.
- Alexander DC, Vasireddy R, Vasireddy S, et al. Emergence of mmpT5 variants during bedaquiline treatment of mycobacterium intracellulare lung disease. J Clin Microbiol. 2017;55(2):574–584.
- Zweijpfenning SMH, Schildkraut JA, Coolen JPM, et al. Failure with acquired resistance of an optimised bedaquiline-based treatment regimen for pulmonary mycobacterium avium complex disease. Eur Respir J. 2019;54(1):1900118.
- Ruth MM, Sangen JJN, Remmers K, et al. A bedaquiline/clofazimine combination regimen might add activity to the treatment of clinically relevant non-tuberculous mycobacteria. J Antimicrob Chemother. 2019;74(4):935–943.
- Rockland M, Ruth MM, Aalders N, et al. Implementation of semiautomated antimicrobial susceptibility interpretation hardware for nontuberculous mycobacteria may overestimate susceptibility. J Clin Microbiol. 2019;57(4):e01756–18.
- Koh WJ, Hong G, Kim SY, et al. Treatment of refractory mycobacterium avium complex lung disease with a moxifloxacin-containing regimen. Antimicrob Agents Chemother. 2013;57(5):2281–2285.
- Winthrop KL, Ku JH, Marras TK, et al. The tolerability of linezolid in the treatment of nontuberculous mycobacterial disease. Eur Respir J. 2015;45(4):1177–1179.
- Ruth MM, Magombedze G, Gumbo T, et al. Minocycline treatment for pulmonary mycobacterium avium complex disease based on pharmacokinetics/pharmacodynamics and bayesian framework mathematical models. J Antimicrob Chemother. 2019;74(7):1952–1961.
- Ruth MM, Koeken VACM, Pennings LJ, et al. Is there a role for tedizolid in the treatment of non-tuberculous mycobacterial disease? J Antimicrob Chemother. 2019;75(3):609–617.
- Yuste JR, Bertó J, Del Pozo JL, et al. Prolonged use of tedizolid in a pulmonary non-tuberculous mycobacterial infection after linezolid-induced toxicity. J Antimicrob Chemother. 2016;72(2):625–628.
- Cowman S, van Ingen J, Griffith D, et al. Non-tuberculous mycobacterial pulmonary disease. Eur Respir J. 2019;54(1):1900250.
- Dedrick RM, Guerrero-Bustamante CA, Garlena RA, et al. Engineered bacteriophages for treatment of a patient with a disseminated drug-resistant mycobacterium abscessus. Nat Med. 2019;25(5):730–733.
- Kaushik A, Ammerman NC, Martins O, et al. In vitro activity of new tetracycline analogs omadacycline and eravacycline against drug-resistant clinical isolates of mycobacterium abscessus. Antimicrob Agents Chemother. 2019;63(6):e00470–19.
- Pearce C, Ruth MM, Pennings LJ, et al. Inhaled tigecycline is effective against mycobacterium abscessus in vitro and in vivo. J Antimicrob Chemother. 2020;75(7):1889–1894.
- Huiberts A, Zweijpfenning SMH, Pennings LJ, et al. Outcomes of hypertonic saline inhalation as a treatment modality in nontuberculous mycobacterial pulmonary disease. Eur Respir J. 2019 Jul;54(1). DOI:https://doi.org/10.1183/13993003.02143-2018
- Lan CC, Lai SR, Chien JY, et al. Nonpharmacological treatment for patients with nontuberculous mycobacterial lung disease. J Formos Med Assoc. 2020 Jun;119(Suppl 1):S42–s50.
- Kitada S, Maekura R, Yoshimura K, et al. Levels of antibody against glycopeptidolipid core as a marker for monitoring treatment response in mycobacterium avium complex pulmonary disease: a prospective cohort study. J Clin Microbiol. 2017 Mar;55(3):884–892.
- Henkle E, Aksamit T, Barker A, et al. Patient-centered research priorities for pulmonary nontuberculous mycobacteria (NTM) infection. An NTM research consortium workshop report. Ann Am Thorac Soc. 2016 Sep;13(9):S379–84.
- Shu CC, Wu MF, and Pan SW, et al. Host immune response against environmental nontuberculous mycobacteria and the risk populations of nontuberculous mycobacterial lung disease. J Formos Med Assoc. 2020;119(Suppl 1):s13–s22.
- Adjemian J, Olivier KN, Seitz AE, et al. Prevalence of nontuberculous mycobacterial lung disease in U.S. medicare beneficiaries. Am J Respir Crit Care Med. 2012;185(8):881–886.