ABSTRACT
Introduction
Aerosol therapy is commonly prescribed in the mechanically ventilated patient. Jet nebulizers (JN) and vibrating mesh nebulizers (VMN) are the most common nebulizer types, however, despite VMN’s well established superior performance, JN use remains the most commonly used of the two. In this review, we describe the key differentiators between nebulizer types and how considered selection of nebulizer type may enable successful therapy and the optimization of drug/device combination products.
Areas covered
Following a review of the published literature up to February 2023, the current state of the art in relation to JN and VMN is discussed under the headings of in vitro performance of nebulizers during mechanical ventilation, respective compatibility with formulations for inhalation, clinical trials making use of VMN during mechanical ventilation, distribution of nebulized aerosol throughout the lung, measuring the respective performance of nebulizers in the patient and non-drug delivery considerations in nebulizer choice.
Expert opinion
Whether for standard care, or the development of drug/device combination products, the choice of nebulizer type should not be made without consideration of the unique needs of the combination of each of drug, disease and patient types, as well as target site for deposition, and healthcare professional and patient safety.
1. Introduction
Aerosol therapy during invasive mechanical ventilation is a frontline therapy in the treatment of a variety of respiratory disorders, including, but not limited to, bronchospasm, mucociliary clearance dysfunction, microbial infections, and inflammation. Globally, the most used aerosol delivery devices during mechanical ventilation are pressurized metered dose inhalers (pMDI), compressor driven jet nebulizers (JN), and vibrating mesh nebulizers (VMN) [Citation1–3].
The mechanism of action and relative aerosol delivery performance of each is well described in the literature, and with a renewed interest in aerosol-mediated delivery of prophylactics and therapeutics in the ventilated patient following from the COVID-19 pandemic, both developers and clinicians are revisiting many of the old practices around aerosol therapy. Both the JN- and VMN-type nebulizers are open cup, typically Class 2 medical devices, suitable for use with aqueous formulations. pMDIs are considered drug/device combination products and come with a very limited selection of ventilator-appropriate medications available, for example, there are no antibiotic pMDI products.
The clinical outcome data in studies evaluating pMDI versus nebulizer in ventilated adult patients is largely published pre-2003, when VMN first became available in the intensive care unit (ICU) setting. Thus, a significant number of studies referring to ‘nebulizer’ performance relate specifically to compressed air-driven JN. These studies largely concluded that pMDI and JN (‘nebulizer’) were equivalent using measures such as bronchodilation [Citation4], but bench studies would suggest that this may be a result of the patients simply having received similar doses, with those doses likely preferentially deposited in the upper conducting airways [Citation5].
Unfortunately, since then there have only been a very limited number of studies investigating comparative clinical outcomes between the different types of aerosol delivery device in mechanically ventilated patients. In those studies, VMN has been shown to outperform both pMDI and JN when measured in terms of clinical responses and length of stay on ventilator [Citation6,Citation7]. Additionally, VMN given its global availability, ease of use, application across all respiratory interventions [Citation8], demonstrated clinical outcomes and exceptionally strong underlying science, has been adopted for many investigator-initiated trials as well as clinical phase evaluations of safety and efficacy, especially during the COVID-19 pandemic.
This expert review aims to detail the relative differences between both JN and VMN types specifically and does not include the pMDI inhalers. Here, we address this topic under a variety of relevant headings that are intended to provide a comprehensive overview of the current state of the art for clinicians and developers alike with a view to optimizing therapeutic approaches in ventilated patients.
2. Available types of nebulizer systems
There are only three VMN systems currently available in the market for acute and critical care applications, that is to say, the Aerogen Solo, the Aerogen Pro and the Philips NIVO (for noninvasive ventilation (NIV) only). While these three make use of the same Aerogen – manufactured nickel palladium alloy vibrating mesh, and are supplied by the millions each year, critically, they are not the same VMN technology in the PDDS nebulizer system used in the unsuccessful INHALE amikacin trials run by Bayer [Citation9]. Following the failure of the INHALE Phase 3 trial to meet its clinical endpoints, that product was abandoned by Bayer, and is no longer manufactured or available in any form [Citation10]. The reasons for the failure of the INHALE, VAPORISE, and IASIS trials have been evaluated and confirmed to not be associated with the nebulizer, and the potential of VMN-delivered antibiotics remains recognized as promising [Citation10,Citation11]. For clarity, those devices listed above are considered actively VMN, which differ in their mode of operation from passive vibrating mesh type devices. Briefly, active VMN is coupled with an annular piezo element that when electrically excited, vibrates, and causes the attached mesh to itself vibrate [Citation12]. Passive VMN devices are comprised of a static mesh, behind which sits a sonotrode. On excitation of the sonotrode, liquid is forced through this static/passive mesh which then becomes aerosolized.
The variety of commercially available JNs is far greater. Given their simple design and construction, they are easily and cheaply manufactured, and consequently, are widely available. However, one key concern is the significant performance variation (droplet size, output rate, delivered dose, residual volume) between JN devices, which has been comprehensively described [Citation13–15].
There have been several improvements in both JN and VMN system designs with both capable of being controlled by the ventilator, or nebulizer controller system itself to operate in a breath synchronized fashion where aerosol is only generated during inhalation, and not exhalation for example [Citation16]. Such designs may provide both direct and indirect patient benefits such as greater aerosol delivery than non-synchronized modes, and the consequent reduction in exhaled aerosol. Reduced aerosol being delivered down the expiratory limb leads to less contamination of expiratory filters, and thus fewer filter changes. It should be noted however, that synchronized delivery does not always guarantee increased aerosol delivery, and in some cases, aerosol is generated during the exhalation phase, so as to build up a bolus in time for the next breath. In one recent study investigating the aerosol delivery performance of continuous JN, synchronized JN and continuous VMN across five circuit positions in three different ventilator types, VMN was seen to consistently deliver more aerosol the end of the endotracheal tube than both versions of JN [Citation17]. An older study, synchronized JN was reported to deliver similar levels of drug in in vitro models of adult and pediatric patients during synchronized inspiratory and expiratory modes, as well as standard continuous output VMN [Citation18]. Breath synchronized VMN has also been described and has been shown to be capable of significant improvements in delivered dose, with a lower respiratory tract delivery of 50–70% of the nominal dose [Citation19]. That system was developed specifically as part of a combination product intended for the delivery of amikacin and was validated by means of clinical trials. Current VMN are not typically breath synchronized, possibly because dose delivery of higher ‘non-substantially equivalent’ doses, would require clinical trials for general purpose Class 2 devices should the dose actually be significantly higher. However, one report of a prototype breath synchronized VMN used during noninvasive positive pressure ventilation in adults, demonstrated higher inhaled doses of amikacin over standard non-synchronized delivery [Citation20].
Integration of JN and VMN controls into the ventilator interface is commonplace. Examples include integrated JN in some Drager and Hamilton ventilator models, as well as integration of VMN into some Maquet, Philips, Hamilton Medical, GE, Medtronic, and Drager ventilator models.
Further, given the looming deadline for EU Medical Device Regulations (MDR) compliance, at least some manufacturers may rationalize their product offerings and so some commodity JN may become unavailable. This is possible given the increasing costs of bringing to and maintaining a medical device in the market, coupled with the expectation of payers and regulators that manufacturers provide more robust evidence of a device’s cost-effectiveness and favorable healthcare economics. Indeed, it has been suggested that such evidence could become a precondition for the market approval and reimbursement of a specific named device, as opposed to classes of devices, thereby increasing the barriers to market entry/staying in the market for low cost, mass production JN [Citation21].
3. Global prevalence of nebulizer usage during mechanical ventilation
Over the years both nebulizer types have been adopted across the clinical spectrum, for example for use with high flow nasal oxygen and NIV [Citation22–24]. VMN was initially adopted for use in the neonate intensive care unit (NICU) and pediatric intensive care unit (PICU) setting given its silent operation, compatibility with ventilator and high flow equipment, and the higher lung doses delivered in those patients [Citation25–27]. Additionally, and unlike JN, VMN does not add flow or pressure to the carefully managed ventilator waveforms and so is well suited to those ventilated NICU and PICU patients, as well as those patients at risk of ventilator induced lung injury through volutrauma or barotrauma [Citation28–30].
Surveys of international aerosol therapy usage in the ICU indicate that anywhere between 48% and 55% of devices used are JN, and between 11% and 15% of nebulizers used are VMN [Citation1–3].
4. Reported in vitro performance of nebulizers during mechanical ventilation
The relative amounts of drug delivered by both JN and VMN has been well described in the literature, but only using a narrow selection of patient parameters, representative of only a few disease states. In all cases, VMN has been shown to deliver more drug to the ventilated lung than the JN, regardless of position in the circuit, interface type (tracheostomy tube versus endotracheal tube) mode of ventilation, mode of action of the nebulizer (e.g. breath actuated) and drug type [Citation5,Citation17,Citation31–33].
Using the published literature to provide representative data points, illustrates the potential fate of the aerosolized drug as it transits through the mechanical ventilation circuit toward its ultimate site of deposition in the airways. The significant sources of loss for the JN includes the residual medication remaining in the nebulizer medication cup after the dose is complete. There are reports of significant retained mass remaining after dose delivery, up to approximately 65% by mass of the original drug dose remaining in the JN in some cases [Citation5,Citation14].
Figure 1. Illustration of the fate of the drug fractions for both VMN and JN during mechanical ventilation. Nominal dose is that dose originally placed in nebulizer. Emitted dose is that dose that escapes the device and is available for inhalation (nominal dose minus residual volume). Total delivered dose is that dose delivered to the distal end of the endotracheal tube. Tracheal dose is that dose deposited within the trachea. Lung dose is that dose that has deposited in the airways, excluding the trachea. Note: Values for emitted dose and total delivered dose are adopted from [Citation5]. The fraction deposited in the trachea and lung was calculated by applying the relative tracheal versus lung ratios reported in [Citation73] to the total delivered dose.
![Figure 1. Illustration of the fate of the drug fractions for both VMN and JN during mechanical ventilation. Nominal dose is that dose originally placed in nebulizer. Emitted dose is that dose that escapes the device and is available for inhalation (nominal dose minus residual volume). Total delivered dose is that dose delivered to the distal end of the endotracheal tube. Tracheal dose is that dose deposited within the trachea. Lung dose is that dose that has deposited in the airways, excluding the trachea. Note: Values for emitted dose and total delivered dose are adopted from [Citation5]. The fraction deposited in the trachea and lung was calculated by applying the relative tracheal versus lung ratios reported in [Citation73] to the total delivered dose.](/cms/asset/02ffcae6-48d8-47f5-a973-789255754654/ierx_a_2183194_f0001_oc.jpg)
JN are reported to produce small droplet sizes however these are carried at high velocity by the air flow that generates the droplets. That increase in velocity likely explains the increased deposition of JN fraction within the ventilator circuitry but also likely predicts deposition of that ballistic fraction very soon after exiting the endotracheal tube, that is to say, in the upper conducting airways [Citation34]. VMN aerosol, with comparable or indeed moderately higher recorded droplet size is nevertheless more efficiently entrained in the ventilator-generated inspiratory flow and therefore is much more likely to penetrate deeper into the lung [Citation5]. More work is needed to increase the current understanding of these relative distributions as an understanding of both the total amount and the distribution of the deposited fraction in the lung together is key in being able to correctly predict and interrogate clinical effects [Citation35].
5. Nebulizer compatibility with formulations for inhalation
Both general purpose VMN and JN are indicated as suitable for use with the majority of those formulations approved for inhalation, but not those already indicated for delivery with a specific device, that is to say, those that are part of a drug device combination product.
Despite the open cup nature of JN and the wide variety and application of JN types available, there are few reports describing controlled studies of JN performance and/or compatibility with specific formulations or formulation types from which generalizable conclusions can be drawn. Indeed, this is likely a result of their level of familiarity that stems from decades of availability and the fact that most practicing clinicians today will have been trained on nebulizer therapy using JN only. However, as a result of this lack of appreciation of fundamentals, the nuances of aerosol therapy and a proper understanding of the potential enabling of therapies nebulizers may provide is lacking. This is not without its risks or missed opportunities for better patient care and drug development programs.
Some JN are known to have issue with micron range suspension formulations, such as corticosteroids, which are nebulized by anywhere between 60% and 80% of clinicians in the ICU [Citation1,Citation2]. One study indicates that following from JN nebulization of 1 and 4.5 μm latex beads, no beads were detected in the inhalable droplet range produced by the JN, with the beads remaining in the nebulizer, and the detected aerosol limited to the aqueous buffer only [Citation36]. Of note, corticosteroid particle sizes, e.g. Pulmicort, are approximately 4.5 μm in diameter, and while it is not well described, it is likely that the mechanism of particle retention involves particles larger than the JN generated droplets, which tend to be approximately 3 μm in diameter, are not transported in those smaller droplets. There have been reports of efficient JN delivery of nano range suspensions, which would support this theory [Citation37]. Nanoparticle aggregation has been reported for JN, and in this instance has been explained as being a result of the buffer evaporation known to occur when the high velocity gas passes through the narrow venturi [Citation38]. VMN is also reported to retain some of the suspended microparticles, with the retention influenced by the particle diameter relative to aperture diameter and not necessarily the droplet diameter. Interestingly, that retained mass is not comprehensively described for actively vibrating VMN devices used in the ICU, whereas it has been for passive vibrating mesh type devices such as the Omron devices. As observed by Najlah et al., VMN [Anonymized] was seen to break up some particles, and therefore there may be the potential for reduced retention given that smaller particles are generated, which in turn are nebulized and not retained [Citation36]. Nevertheless, it can be expected that there will likely be some retained suspension particles in VMN, however, there are no published studies that have fully characterized this for VMN intended for use during mechanical ventilation and whether that amount of retained particles adversely effects the delivered dose, and ultimately clinical outcomes. Two preliminary VMN, [Anonymized] studies suggest that there is still the potential for meaningful doses to be delivered in neonate, pediatric and adult patients [Citation39,Citation40]. Some studies investigating the effect of VMN on formulation characteristics such as osmolality, concentration and temperature suggest that any retained mass in the medication cup reservoir is minimal, with no recorded change in budesonide concentration over time [Citation41]. Sensibly, larger particles can be expected to be retained to a greater degree, and as discussed above, researchers and developers have sought to generate nanosized particles for nanosuspensions [Citation42,Citation43]. In studies investigating the combination of VMN and nanosuspensions, many make no record of significant retention in the medication cup nor aggregation [Citation44–49]. This is an important research area worthy of greater exploration, as it is a gap in the current state of the art, especially for current suspension products, but also considering the potential for future suspension-based ICU-focused therapeutics and prophylactics. Importantly, appropriate formulation design can mitigate many of the undesired characteristics such as aggregation [Citation50].
Similar concentration observations were made where increases in osmolality were noted during JN operation indicating solvent evaporation, and again, these changes were not seen with VMN [Citation51]. Maintenance of the pharmaceutical formulation’s intended osmolality is critical in maintaining the stability and potential for proper action post deposition, however critically, an increase in osmolality is associated with increased cough in patients [Citation52]. For reasons of potential thoracic pneumatosis for example, cough should be avoided during mechanical ventilation [Citation53]. Another consideration of dynamic changes in drug concentration over time, is the lack of accurate control of dosing. Concentrations at the start of the dose, will be as prescribed, however, as the dose continues, and concentration increases, there may be instability in the formulation, potential toxicity concerns, or at a very basic level, no understanding of what the cumulative amount of drug the patient may have received was over time.
The practice of nebulized drug admixtures, where co-administration of drugs separately prescribed, is not recommended, but is a widely reported practice. Admixtures have been shown to adversely affect JN performance. The degree of deviation from the JN manufacturer-stated aerosol performance is unpredictable, given the wide potential for admixed drugs, and their excipient interactions [Citation54–56].
Retained drug mass may be further influenced by the physicochemical properties of the drug, and its tendency to froth, for example. In the case of the colistimethate sodium (CMS), which has a known tendency to frothing, there are reports of 100% ethanol being added to the JN in order to reduce the frothing and aid nebulization [Citation57]. While approved at low concentrations for use in some pMDIs, high concentrations of ethanol are not on the generally recognized as safe (GRAS) list of formulation excipients and carries obvious toxicity concerns. In contrast, when CMS is being nebulized by VMN, it may simply require further dilution with the standard reconstitution buffer in order to increase the surface tension of the solution, so as to improve the output rate and also stability of CMS. The overall delivered dose of CMS, and aerosol characteristics for VMN-nebulized CMS have been shown to remain the same when this approach is taken [Citation58].
Overall, VMN has broad compatibility with a variety of formulations, but it is important to note that VMN’s aerosol performance may be influenced by the physicochemical properties of the formulation itself, that is to say, surface tension and viscosity [Citation59]. Higher viscosity leads to a reduction in the output rate, whereas very low surface tension, may result in drug slipping through the mesh apertures, a phenomenon known as the ‘Loxy effect’. The size of the suspended particles may also have an effect on VMN aerosol performance; however, it has been clearly demonstrated that VMN is less prone to issues with suspended particles than JN [Citation36]. This is likely due to the active vibration of the mesh itself, and the consequent breaking up of larger particles, coupled with self-clearing of mesh apertures as they vibrate at approximately 128,000 times per second.
Further, VMN is compatible with several labile formulations, that may be prone to degradation during the process of nebulization. This broad compatibility across drug and formulation type positions VMN well for delivery of future therapeutics, including but not limited to biologics, cell therapies, extracellular vesicles, bacteriophage, vaccines, antibodies and nanoparticle formulations to name a few [Citation60–71]. See for examples of relevance in the acute and critical care environment.
Table 1. Examples of vibrating mesh nebulizer (VMN)-delivered therapeutics with application in the acute and critical care setting.
6. Distribution of nebulized aerosol throughout the lung
Critically, and despite, a solid understanding of how much in total is delivered to the lung, little work has been carried out in understanding how much goes where, that is to say, the relative deposition patterns achieved with the different modern nebulizers. Previous work has looked at the ultrasonic type nebulizers, which are no longer readily available [Citation71]. Dugernier et al. published an imaging study in adult patients undergoing both volume controlled, and pressure support ventilation modes, indicating that VMN delivers a homogenous distribution of aerosol throughout the lung [Citation72]. These authors are not aware of any similar studies conducted in ventilated adult patients using JN, nor any such studies directly comparing VMN to JN.
Preliminary scintigraphy results were however recently presented indicating that, in a mechanically ventilated ex vivo pig lung model (volume-controlled ventilation, Vt 800 mL, I:E 1:2, RR 15, 105–125 kg animals) trachea versus lung deposition ratios were 1:2.27, 1:0.61, and 1:0.60 for VMN, JN and pMDI, respectively. This indicates that more than twice the aerosol fraction is delivered into the lung, as opposed to the trachea for the VMN, whereas the majority of the dose is deposited in the trachea with less of the dose delivered to the lung when using a JN and pMDI [Citation73].
Another contributor to aerosol distribution and ultimately the location of deposition is the disease state and relative ventilation between lung lobes. This is well described for spontaneous breathing patients, however, in the ventilated patient population, there is a paucity of information on the effect of mechanical ventilation settings, modes, flow rates, airway patency, and disease state [Citation74–76].
More work is required in this area in order to better understand the fundamental differences between the deposition patterns with each device type and the ventilation parameters themselves as this will better allow for design of optimal treatment strategies that are both drug and disease appropriate.
7. Measuring the respective performance of nebulizers in the patient
The relative advantages of one nebulizer type over the other are tightly linked with the drug of interest, its mechanism of action and patient type. There are several means of comparing relative device performance and these include radio-imaging studies, respiratory mechanics measurements, and drug sampling post-delivery.
Radio-imaging studies have formed an integral part of both drug and device development pathways for inhalation products. As stated above, an understanding of the total deposition, as well as the distribution is critical in optimizing design, and treatment strategy, and radio-imaging studies have contributed significantly to this. Radio-imaging allows for sensitive quantification of the total deposited dose, its distribution within the lung, and clearance dynamics [Citation77–79].
Respiratory mechanics measurements, that is to say, monitoring of clinical readouts such as improvements in airway resistance, arterial oxygen levels et cetera, will be directed by the specific disease and provide clinically meaningful measures of patient response to therapy.
Drug sampling post-delivery is often an indirect measure of drug delivery, both the total and its distribution within the body. The specific location of sampling will again be directed by specific disease and drug type. Sampling may be carried out locally in the lung in order to assess pharmacokinetic/pharmacodynamic (PK/PD), for example using broncho alveolar lavage (BAL), epithelial lining fluid (ELF), or lung micro dialysis, systemically, looking at blood, or via the urine. Each have their merits with respect to ease of application, appropriateness, sensitivity and reliability, and are often more descriptive when used in combination [Citation80].
We read with interest the article by Kyriakoudi et al. who studied the PK characteristics of nebulized CMS using both JN and VMN in critically ill adult patients with ventilator associated respiratory infections [Citation81]. Here both ELF and blood levels of CMS were used to compare the relative efficacy of both device types, and in ELF samples similar levels of CMS were recorded. The highest blood levels of CMS were noted in the for VMN, with the authors concluding that JN was likely a viable alternative to VMN in these patients. However, these observations are likely explained by the propensity of JN to deliver mainly to the upper airways, with more seen in the lower airways with VMN. That is to say the fraction of VMN dose delivered to the upper airways being similar to JN, but the comparative VMN fraction is higher in the peripheral airways, which have greater access to the systemic circulation, and thus may explain the higher CMS blood levels. Further, given the well described limitation in total dose delivery in the ventilated patient, the adoption of JN for antibiotic delivery should be carefully considered, especially given the risk of suboptimal dosing, and the consequent development of MDR bacteria. Despite colistin’s long history of use, there are increasing fears of the spread of the plasmid-mediated mcr-1 colistin resistance gene, and so vigilance must be maintained not only in its appropriate prescription but also the way it is delivered to the lung for maximal effect with minimal dose [Citation82].
Compared to the higher blood levels of CMS post-intravenous administration, VMN-administered CMS, with its higher than JN-administered CMS blood levels does not seem to be related with any adverse side effects in patients. In a recent randomized control trial in ventilator-associated pneumonia (VAP) in pediatrics, Sachdev et al. evaluated the efficacy and safety of nebulized CMS in the treatment of Gram-negative VAP. VMN-nebulized colistin was safe with no major adverse effects [Citation83]. A study by Pourheidar et al. evaluated the efficacy and safety of IV ampicillin-sulbactam plus nebulized colistin in the treatment of VAP caused by MDR Acinetobacter in adult ICU patients, as an alternative to IV plus nebulized colistin. Both groups received nebulized colistin every 8 h with VMN. The results demonstrated that the high dose IV ampicillin-sulbactam plus nebulized colistin regimen has comparable efficacy with IV plus nebulized colistin in the treatment of VAP caused by MDRA, with sensitivity to colistin only, with probably lower incidence of kidney injury [Citation84]. Further, in another adult patient study, Lu et al. demonstrated that VMN-nebulization of high-dose colistin was effective in treating VAP caused by multidrug-resistant Pseudomonas aeruginosa (59% of patients cured) or Acinetobacter baumannii (91% of patients cured) [Citation85]. Finally, another recent adult study investigating the pulmonary and systemic PKs of CMS in VAP patients noted that following VMN-mediated delivery, plasma concentrations were lower than those associated with nephrotoxicity [Citation86].
Indeed, in many applications VMN is used in order to target the systemic circulation. VMN was originally developed with a focus on inhaled insulin and was shown to be effective in that regard [Citation87]. Another example of systemically targeted drug is nebulized epoprostenol. Nebulized epoprostenol is an alternative for inhaled nitric oxide in the management of pulmonary arterial hypertension and possibly acute hypoxemia. VMN, in combination with a continuous nebulization tube set has been reported used in its delivery [Citation88,Citation89].
The various methods used to measure deposition and the concentration of nebulized antibiotics at the site of infection have several technical limitations. ELF sampling is a useful tool and has been used to look at PK/PD parameters for a variety of antibiotics delivered to the airways. However, it is limited in its ability to represent drug distribution throughout the whole lung and in critically ill patients with lower respiratory tract infections greater variability in ELF concentrations is acknowledged [Citation80,Citation90,Citation91]. Further, the measurement of ELF concentrations of antibiotics could be skewed by contamination as a result of lysis of ELF cells and technical constraints of BAL, as well as reliable assessment of the unbound drug concentration in the lung [Citation92]. As such, we would posit that ELF as a means of comparing the performance of different nebulizer types in critically ill patients is regrettably an inappropriate approach, and further development of techniques such as micro-dialysis, are required to better characterize local antibiotic concentrations [Citation93].
Fundamentally, effective treatment of ventilator associated respiratory infections (VARI) requires a combination of an effective antimicrobial, an effective delivery system capable of delivering sufficient quantities to the lung, and further, capable of delivering throughout the lung in order to maximize targeting to the infection sites [Citation94]. This can only be done with a nebulizer that is capable of widespread distribution of aerosol throughout the lung.
Drug sampling post-delivery is a well-established method of comparing nebulizers by means of relative lung bioavailability. Measures such as the urinary PKs method provides greater sensitivity and less variation than measures such as BAL and ELF. A range of inhaled drugs have been demonstrated as suitable pharmacologic tracers for comparing airway deposition between nebulizers, these include Tobramycin, Gentamycin, Ipratropium, Salbutamol, and others [Citation95–97]. The urinary PKs method has been used in the investigation of relative lung bioavailability following delivery of various formulations during mechanical ventilation of patients. Uses have included an assessment of the effect of humidification on the delivered dose in patients, as well as comparing nebulizers to pMDI.
In a randomized crossover design involving thirty-six asthmatic adult patients in receipt of mechanical ventilation, urinary salbutamol was used as a measure of the amount of aerosol delivered to the lung under dry and humidified ventilator circuit conditions when using VMN, JN and pMDI [Citation7]. In this study, no significant difference was noted between the two humidification conditions, for all devices, however, the VMN was noted to have significantly higher systemic bioavailability of salbutamol than JN. In a separate study by the same group, clinical outcomes were investigated in ventilated asthmatic adult patients [Citation6]. Here, VMN resulted in a trend to shorter ICU days (~1.4-day reduction).
Following from that observation of a shorter ICU stay and echoed in other clinical studies such as with reduced length of stay in spontaneous breathing adult patients in the emergency department, and in pediatric subjects with asthma, the relative drug delivery performance of each nebulizer type should be viewed with the bigger picture in mind, and not just delivered dose [Citation98,Citation99]. The implication of larger delivered doses goes beyond fewer doses being required to compensate for poor nebulizer performance, but they impact healthcare economics where lower admissions and shorter lengths of stay reduces healthcare costs, fewer doses lower drug costs, fewer visits to the bedside for refilling of a nebulizer reduces caregiver costs and workload. Nevertheless, it must be stated also, that despite the potential for lower efficiency of delivery et cetera, JN can and do deliver sufficient drug in many cases. Often that speaks more to the potency of the drug than the performance of the device, but importantly JN named on the drug label for use with specific drugs have been proven through extensive clinical trials to be effective delivery systems for use in that combination.
8. Non-drug delivery considerations
Further considerations for aerosol delivery during mechanical ventilation go beyond even the therapeutic objective. Following from the SARS epidemic and COVID-19 pandemic, maintenance of a closed circuit became a critical consideration in mitigating bystander exposure to exhaled patient-derived bioaerosol, or indeed just exhaled fugitive medical aerosols. JN need to be disassembled for drug refill and the resulting opening of the patient circuit, and consequent escape of patient-derived bioaerosol but also the increased risk of de-recruitment and atelectasis of the lung [Citation29,Citation100]. VMN have been shown to maintain the closed patient circuit throughout operation without the need to break that circuit for a refill or cleaning, see [Citation29,Citation30,Citation101]. This inherent advantage of VMN is reflected in several guidance and recommendation documents issued around the world where VMN is specified as capable of maintaining the necessary closed circuit [Citation102–108].
Figure 2. (a) Illustration of the maintenance of the closed ventilator circuit during the drug refill process for the vibrating mesh nebulizer (VMN). (b) Illustration of the opening of the ventilator circuit for the process of drug refill using the JN. Blue lines indicate normal air flow within the closed circuit. Red arrows indicate leaked air from opened circuit. Adopted from [Citation29].
![Figure 2. (a) Illustration of the maintenance of the closed ventilator circuit during the drug refill process for the vibrating mesh nebulizer (VMN). (b) Illustration of the opening of the ventilator circuit for the process of drug refill using the JN. Blue lines indicate normal air flow within the closed circuit. Red arrows indicate leaked air from opened circuit. Adopted from [Citation29].](/cms/asset/7a276dc5-7ef0-4a42-b545-ad6bafa976ec/ierx_a_2183194_f0002_oc.jpg)
An additional concern for JN is the potential for microbe-laden patient secretions migrating into the medication cup. This has been recorded in the clinic and is well described [Citation109–113]. Patient secretion-derived contamination is made possible by the fact that JN are typically manufacturer-indicated for inclusion in the patient circuit at or near the patient wye. With the medication cup resting below the circuit, secretions can fall into the JN and become aerosolized, being delivered to the lung as an aerosol. Additionally, given the large openings, and necessity to completely open and handle the JN medication reservoir or cup, there is an increased likelihood of unintended nosocomial infection through handling. These design features of JN and the consequent risk of microbial contamination may play a role in VARI.
VMN by design must be positioned above the circuit and may be placed as far back as the ventilator side of the humidifier. As such, it is protected from patient secretions by several feet of inspiratory limb tubing, as well as the humidifier pot. Furthermore, the VMN medication cup is isolated from the patient circuit by the vibrating mesh element itself. See .
Figure 3. Potential positioning of the closed-circuit vibrating mesh nebulizer (VMN) and open circuit compressed-air driven jet nebulizer (JN) is illustrated in the patient circuit of a mechanical ventilator. Note: VMN may be placed remote to the patient, where the JN is most commonly placed, and often mandated at the patient eye.
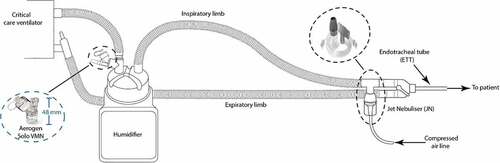
9. Conclusion
With the development of new and repurposing of old therapeutics, nebulized aerosols will most certainly play an enabling role in making new prophylactics and therapeutics available to the critically ill patient. In order to realize this potential, a greater understanding of aerosol therapy, and more specifically, nebulizer types are required. VMN are increasingly being adopted for application in the acute and critical care setting, and their inherent advantages which include greater dose delivery, wider aerosol deposition patterns within the lung, compatibility with labile formulations, and healthcare provider and patient safety will likely result in more focus on these devices for both standard care and novel drug/device combination products.
10. Expert opinion
Poor clinical outcomes are still unacceptably high despite, in many cases, adequate empiric proof of the potential of inhaled therapies in the mechanically ventilated patient. This presents an opportunity to invest further time and considered research in prompt, and most importantly, adequate therapeutic delivery using the right tools. Developers, patients, and physicians should work closely to better understand drug delivery and ultimately, to decrease the mortality burden in preventable and treatable conditions such as ventilator associated lower respiratory tract infections. Critically, shortcuts without rigorous or early evaluation of the potential, relative advantages or disadvantages of a drug delivery approach should not become common practice in the emergent field of nebulizer science.
Echoed by Rouby et al., planning and interrogation of any clinical outputs following aerosol-mediated delivery of anti-infectives, as an example, will need to take a holistic approach [Citation11]. A thorough knowledge and reporting of details such as nebulizer type, location of nebulizer within the ventilator circuit, ventilation parameters, an understanding of likely location of deposition, delivered lung dose over time as well as appropriate sampling will be required.
Greater attention should be paid to appropriate selection of nebulizer type when considering disease type, drug type, patient type and preferred location and amount of the dose to be delivered. Nebulizers are not simply noninvasive syringes for drug delivery.
Article highlights
Global aerosol therapy surveys: Jet and vibrating mesh nebulizers the most commonly used nebulizer types during mechanical ventilation.
Compatibility with formulations: Jet nebulizers are known to leave up to 65% of the nominal dose in the nebulizer after dosing is complete. Strategies to improve the ability to nebulize drugs such as frothing-prone colistin are simpler and potentially safer with vibrating mesh nebulizers.
Total Drug Delivery: Vibrating mesh nebulizers deliver more drug to the mechanically ventilated patient lung.
Drug Distribution: Is known to vary between nebulizer types with vibrating mesh nebulizers distributing the aerosol more widely throughout the lung, however, more research is required in this area.
Pharmacokinetics: A combination of PK methods are required to accurately compare the relative drug delivery performance of the two different nebulizer types. The higher than jet nebulizer blood levels of colistin noted with vibrating mesh nebulizers is still lower than those seen with intravenous administration, and thus far have not been associated with adverse side effects such as nephrotoxicity.
Healthcare professional and patient safety: Vibrating mesh nebulizers are the only nebulizer type that maintains the closed ventilator circuit, preventing lung de-recruitment, and mitigates against the risk of release of infectious and medical aerosols. Further, they do not add flow or pressure to the circuit.
Declaration of interest
R MacLoughlin is an employee of Aerogen. The authors have no other relevant affiliations or financial involvement with any organization or entity with a financial interest in or financial conflict with the subject matter or materials discussed in the manuscript apart from those disclosed.
Reviewer disclosures
Peer reviewers on this manuscript have no relevant financial or other relationships to disclose.
Article highlights
Global aerosol therapy surveys: Jet and Vibrating Mesh Nebulizers the most commonly used nebulizer types during mechanical ventilation.
Compatibility with formulations: Jet nebulizers are known to leave up to 65% of the nominal dose in the nebulizer after dosing is complete. Strategies to improve the ability to nebulize drugs such as frothing-prone colistin are simpler and potentially safer with vibrating mesh nebulizers.
Total Drug Delivery: Vibrating mesh nebulizers deliver more drug to the mechanically ventilated patient lung.
Drug Distribution: Is known to vary between nebulizer types with vibrating mesh nebulizers distributing the aerosol more widely throughout the lung, however, more research is required in this area.
Pharmacokinetics: A combination of PK methods are required to accurately compare the relative drug delivery performance of the two different nebulizer types. The higher than jet nebulizer serum blood levels of colistin noted with vibrating mesh nebulizers is still lower than those seen with intraveneous administration, and thus far have not been associated with adverse side effects such as nephrotoxicity.
Healthcare professional and patient Safety: Vibrating Mesh Nebulizers are the only nebulizer type that maintains the closed ventilator circuit, preventing lung de-recruitment, and mitigates against the risk of release of infectious and medical aerosols. Further, they do not add flow or pressure to the circuit.
Acknowledgment
The authors would like to thank Dr Elena Fernández Fernández for suggesting the need for this manusript.
Additional information
Funding
References
- Sun Q, Chang W, Liu X, et al. Aerosol therapy during mechanical ventilation in intensive care units: a questionnaire-based survey of 2203 ICU medical staff in China. J Intens Med. 2022;2:189–194.
- Ehrmann S, Roche-Campo F, Sferrazza Papa GF, et al. Aerosol therapy during mechanical ventilation: an international survey. Intensive Care Med. 2013;39:1048–1056.
- Ehrmann S, Roche-Campo F, Bodet-Contentin L, et al. Aerosol therapy in intensive and intermediate care units: prospective observation of 2808 critically ill patients. Intensive Care Med. 2016;42:192–201.
- Duarte AG, Momii K, Bidani A. Bronchodilator therapy with metered-dose inhaler and spacer versus nebulizer in mechanically ventilated patients: comparison of magnitude and duration of response. Respir Care. 2000;45:817–823.
- Naughton PJ, Joyce M, Mac Giolla Eain M, et al. Evaluation of aerosol drug delivery options during adult mechanical ventilation in the COVID-19 era. Pharmaceutics. 2021;13:1574.
- Moustafa IOF, ElHansy MHE, Al Hallag M, et al. Clinical outcome associated with the use of different inhalation method with and without humidification in asthmatic mechanically ventilated patients. Pulm Pharmacol Ther. 2017;45:40–46.
- Moustafa IOF, MRA-A A, Al Hallag M, et al. Lung deposition and systemic bioavailability of different aerosol devices with and without humidification in mechanically ventilated patients. Heart Lung. 2017;46:464–467.
- Fernández Fernández E, Joyce M, O’Sullivan A, et al. Evaluation of aerosol therapy during the escalation of care in a model of adult cystic fibrosis. Antibiotics. 2021;10:472.
- Luyt C-E, Clavel M, Guntupalli K, et al. Pharmacokinetics and lung delivery of PDDS-aerosolized amikacin (NKTR-061) in intubated and mechanically ventilated patients with nosocomial pneumonia. Crit Care. 2009;13:R200.
- Fink JB. Reliability of mesh aerosol devices used during INHALE trial. J Aerosol Med Pulm Drug Deliv. 2022;35:107–108.
- Rouby -J-J, Monsel A, Leone M, et al. The IASIS, INHALE and VAPORISE trials. Reasons for a triple failure: study design, aminoglycosides dosing and technique of nebulisation. Anaesth Crit Care Pain Med. 2020;39:179–183.
- Olszewski OZ, MacLoughlin R, Blake A, et al. A silicon-based MEMS vibrating mesh nebulizer for inhaled drug delivery. Procedia Eng. 2016;168:1521–1524.
- Adorni G, Seifert G, Buttini F, et al. Aerosolization performance of jet nebulizers and biopharmaceutical aspects. Pharmaceutics. 2019;11:406.
- Zhou Y, Ahuja A, Irvin CM, et al. Evaluation of nebulizer performance under various humidity conditions. J Aerosol Med. 2005;18:283–293.
- Hatley RH, Byrne SM. Variability in delivered dose and respirable delivered dose from nebulizers: are current regulatory testing guidelines sufficient to produce meaningful information? Med Devices. 2017;10:17–28.
- Longest W, Spence B, Hindle M. Devices for improved delivery of nebulized pharmaceutical aerosols to the lungs. J Aerosol Med Pulm Drug Deliv. 2019;32:317–339.
- Hou H, Xu D, Dai B, et al. Position of different nebulizer types for aerosol delivery in an adult model of mechanical ventilation. Front Med. 2022;9:2819.
- Wan G-H, Lin RRT, Fink JB, et al. In vitro evaluation of aerosol delivery by different nebulization modes in pediatric and adult mechanical ventilators. DOI:10.4187/respcare.02999.
- Dhand R, Sohal H. Pulmonary drug delivery system for inhalation therapy in mechanically ventilated patients. Expert Rev Med Devices. 2014;5(1):9–18.
- Michotte J-B, Staderini E, Aubriot A-S, et al. Pulmonary drug delivery following continuous vibrating mesh nebulization and inspiratory synchronized vibrating mesh nebulization during noninvasive ventilation in healthy volunteers. J Aerosol Med Pulm Drug Deliv. 2018;31:33–41.
- Ben-Menahem SM, Nistor-Gallo R, Macia G, et al. How the new European regulation on medical devices will affect innovation. Nat Biomed Eng. 2020;4:585–590.
- Li J, Fink JB, MacLoughlin R, et al. A narrative review on trans-nasal pulmonary aerosol delivery. Crit Care. 2020;24:506.
- Galindo-Filho VC, Alcoforado L, Rattes C, et al. A mesh nebulizer is more effective than jet nebulizer to nebulize bronchodilators during non-invasive ventilation of subjects with COPD: a randomized controlled trial with radiolabeled aerosols. Respir Med. 2019;153:60–67.
- Dugernier J, Hesse M, Jumetz T, et al. Aerosol delivery with two nebulizers through high-flow nasal cannula: a randomized cross-over single-photon emission computed tomography-computed tomography study. J Aerosol Med Pulm Drug Deliv. 2017;30:349–358.
- Dubus JC, Vecellio L, de Monte M, et al. Aerosol deposition in neonatal ventilation. Pediatr Res. 2005;58:10–14.
- Réminiac F, Vecellio L, MacLoughlin R, et al. Nasal high flow nebulization in infants and toddlers: an in vitro and in vivo scintigraphic study. Pediatr Pulmonol. 2017;52:337–344.
- MacLoughlin R, Telfer C, Clark A, et al. Aerosol: a novel vehicle for pharmacotherapy in neonates. Curr Pharm Des. 2017;23:5928–5934.
- Li X, Tan W, Zhao H, et al. Effects of jet nebulization on ventilator performance with different invasive ventilation modes: a bench study. Front Med (Lausanne). 2022;9:3024.
- Joyce M, McGrath JA, Mac Giolla Eain M, et al. Nebuliser type influences both patient-derived bioaerosol emissions and ventilation parameters during mechanical ventilation. Pharmaceutics. 2021;13:199.
- Wang R, Leime CO, Gao W, et al. Aerosol delivery in models of pediatric high flow nasal oxygen and mechanical ventilation. Pediatr Pulmonol. 2022. DOI:10.1002/ppul.26270.
- Berlinski A, Willis JR. Effect of tidal volume and nebulizer type and position on albuterol delivery in a pediatric model of mechanical ventilation. Respir Care. 2015;60:1424–1430.
- Berlinski A, Willis JR. Albuterol delivery by 4 different nebulizers placed in 4 different positions in a pediatric ventilator in vitro model. Respir Care. 2013;58:1124–1133.
- Ari A, Fink JB. Inhalation therapy in patients with tracheostomy: a guide to clinicians. Expert Rev Respir Med. 2017;11 3 :201–208.
- Harvey CJ, O’Doherty MJ, Page CJ, et al. Comparison of jet and ultrasonic nebulizer pulmonary aerosol deposition during mechanical ventilation. Eur Respir J. 1997;10:905–909.
- Dugernier J, Ehrmann S, Sottiaux T, et al. Aerosol delivery during invasive mechanical ventilation: a systematic review. Crit Care. 2017;21:264.
- Najlah M, Parveen I, Alhnan MA, et al. The effects of suspension particle size on the performance of air-jet, ultrasonic and vibrating-mesh nebulisers. Int J Pharm. 2014;461:234–241.
- Rossi I, Sonvico F, McConville JT, et al. Nebulized coenzyme Q 10 nanosuspensions: a versatile approach for pulmonary antioxidant therapy. Eur J Pharm Sci. 2018;113:159–170.
- Hernández-Trejo N, Kayser O, Steckel H, et al. Characterization of nebulized buparvaquone nanosuspensions—effect of nebulization technology. J Drug Target. 2005;13:499–507.
- Brady P, McKillop C, Joyce M, et al. P11.4 Choice of nebuliser type affects lung dosing with budesonide in both adult and paediatric patients during mechanical ventilation. Ir J Med Sci. 2018;187:237–303.
- Job S, Kopuri A, Ives K, et al. PO-0743 Use of a new-generation electronic micropump nebuliser to deliver budesonide in chronic lung disease: a feasible alternative to systemic dexamethasone? Arch Dis Child. 2014;99:A498.2–A498.
- Hu J, Chen X, Li S, et al. Comparison of the performance of inhalation nebulizer solution and suspension delivered with active and passive vibrating-mesh device. J Drug Deliv Sci Technol. 2020;55:101353.
- Amani A, Amini MA, Ali HSM, et al. Alternatives to conventional suspensions for pulmonary drug delivery by nebulisers: a review. J Pharm Sci. 2011;100:4563–4570.
- Forest V, Pourchez J. Nano-delivery to the lung – by inhalation or other routes and why nano when micro is largely sufficient? Adv Drug Deliv Rev. 2022;183:114173.
- Doroudian M, O’Neill A, O’Reilly C, et al. Aerosolized drug-loaded nanoparticles targeting migration inhibitory factors inhibit Pseudomonas aeruginosa induced inflammation and biofilm formation. Nanomedicine. 2020;15(30):2933–2953. DOI:10.2217/nnm-2020-0344.
- Bahlool AZ, Fattah S, O’Sullivan A, et al. Development of inhalable ATRA-loaded PLGA nanoparticles as host-directed immunotherapy against tuberculosis. Pharmaceutics. 2022;14:1745.
- Verma NK, Crosbie-Staunton K, Satti A, et al. Magnetic core-shell nanoparticles for drug delivery by nebulization. J Nanobiotechnology. 2013;23(11):1. DOI:10.1186/1477-3155-11-1.
- Gaspar MM, Gobbo O, Ehrhardt C. Generation of liposome aerosols with the Aeroneb Pro and the AeroProbe nebulizers. J Liposome Res. 2010;20(1):55–61.
- Zhu YG, Meng SM, Monsel A, et al. Nebulized exosomes derived from allogenic adipose tissue mesenchymal stromal cells in patients with severe COVID-19: a pilot study. Stem Cell Res Ther. 2022;13. DOI:10.1186/s13287-022-02900-5
- Fernández Fernández E, Santos-Carballal B, de Santi C, et al. Biopolymer-based nanoparticles for cystic fibrosis lung gene therapy studies. Materials. 2018;11:122.
- Sécher T, Bodier-Montagutelli E, Parent C, et al. Aggregates associated with instability of antibodies during aerosolization induce adverse immunological effects. Pharmaceutics. 2022;14:671.
- Sidler-Moix AL, Di Paolo ER, Dolci U, et al. Physicochemical aspects and efficiency of albuterol nebulization: comparison of three aerosol types in an in vitro pediatric model. Respir Care. 2015;60:38–46.
- Sahakijpijarn S, Smyth HDC, Miller DP, et al. Post-inhalation cough with therapeutic aerosols: formulation considerations. Adv Drug Deliv Rev. 2020;165–166:127–141.
- Tachiiri Y, Inoue S, Kawaguchi M. A case of thoracic pneumatosis due to severe coughs and tracheal tube displacement induced by tracheal tube size mismatch. JA Clin Rep. 2019;5:1–4.
- Kamin W, Erdnüss F, Krämer I. Inhalation solutions — which ones may be mixed? Physico-chemical compatibility of drug solutions in nebulizers — update 2013. J Cyst Fibros. 2014;13:243–250.
- Krämer I, Schwabe A, Lichtinghagen R, et al. Physicochemical compatibility of mixtures of dornase alfa and tobramycin containing nebulizer solutions. Pediatr Pulmonol. 2009;44:134–141.
- Berlinski A, Waldrep JC. Nebulized drug admixtures: effect on aerosol characteristics and albuterol output. J Aerosol Med. 2006;19(4):484–490. http://www.liebertpub.com/jam
- Katz SL, Ho SL, Coates AL. Nebulizer choice for inhaled colistin treatment in cystic fibrosis. Chest. 2001;119:250–255.
- Bihan K, Zahr N, Becquemin M-H, et al. Influence of diluent volume of colistimethate sodium on aerosol characteristics and pharmacokinetics in ventilator-associated pneumonia caused by MDR bacteria. J Antimicrob Chemother. 2018;73:1639–1646.
- Ghazanfari T, Elhissi AMA, Ding Z, et al. The influence of fluid physicochemical properties on vibrating-mesh nebulization. Int J Pharm. 2007;339:103–111.
- Brave H, MacLoughlin R. State of the art review of cell therapy in the treatment of lung disease, and the potential for aerosol delivery. Int J Mol Sci. 2020;21:6435.
- Azhdari MH, Goodarzi N, Doroudian M, et al. Molecular insight into the therapeutic effects of stem cell-derived exosomes in respiratory diseases and the potential for pulmonary delivery. Int J Mol Sci. 2022;23:6273.
- Pitiot A, Heuzé-Vourc’h N, Sécher T. Alternative routes of administration for therapeutic antibodies—state of the art. Antibodies. 2022;11:56.
- Fröhlich E, Salar-Behzadi S. Oral inhalation for delivery of proteins and peptides to the lungs. Eur J Pharm Biopharm. 2021;163:198–211.
- Chow MYT, Qiu Y, Lam JKW. Inhaled RNA therapy: from promise to reality. Trends Pharmacol Sci. 2020;41:715–729.
- Chang RYK, Wallin M, Lin Y, et al. Phage therapy for respiratory infections. Adv Drug Deliv Rev. 2018;133:76–86.
- Doroudian M,O, Neill A, Mac Loughlin R, et al. Nanotechnology in pulmonary medicine. Curr Opin Pharmacol. 2021;56:85–92.
- Brennan LC, O’Sullivan A, MacLoughlin R. Cellular therapy for the treatment of paediatric respiratory disease. Int J Mol Sci. 2021;22:8906.
- Daly S, O’Sullivan A, MacLoughlin R. Cellular immunotherapy and the lung. Vaccines (Basel). 2021;9:1018.
- McCarthy SD, González HE, Higgins BD. Future trends in nebulized therapies for pulmonary disease. J Pers Med. 2020;10:37.
- Masterson CH, McCarthy SD, O’Toole D, et al. The role of cells and their products in respiratory drug delivery: the past, present, and future. Expert Opin Drug Deliv. 2020;17:1689–1702.
- Aerosolized Pulmonary Delivery of mRNA Constructs Attenuates Severity of Escherichia coli Pneumonia in the Rat Sean D. McCarthy, Christopher B. Rohde, Matt Angel, Claire H. Masterson, Ronan MacLoughlin, Juan Fandiño, Héctor E. González, Declan Byrnes, John G. Laffey, and Daniel O'Toole Nucleic Acid Therapeutics 0 0:0 DOI :10.1089/nat.2022.0049.
- Vatzia E, Feest K, McNee A, et al. Immunization with matrix-, nucleoprotein and neuraminidase protects against H3N2 influenza challenge in pH1N1 pre-exposed pigs. npj Vaccines. 2013;8:19. DOI :10.1038/s41541-023-00620-2.
- Klockare M, Dufva A, Danielsson A-M, et al. Comparison between direct humidification and nebulization of the respiratory tract at mechanical ventilation: distribution of saline solution studied by gamma camera. J Clin Nurs. 2006;15:301–307.
- Dugernier J, Reychler G, Wittebole X, et al. Aerosol delivery with two ventilation modes during mechanical ventilation: a randomized study. Ann Intensive Care. 2016;6:73.
- MacLoughlin R, Champigny C, Montharu J, et al. Aerosol deposition in a mechanically ventilated ex vivo porcine lung using a vibrating mesh nebuliser and a pressurized metered dose inhaler. Drug Delivery to the Lungs Conference; 2022. https://ddl-conference.com/ddl2022/conference-papers/aerosol-deposition-in-a-mechanically-ventilated-ex-vivo-porcine-lung-using-a-vibrating-mesh-nebuliser-and-a-pressurized-metered-dose-inhaler/. accessed 2022 Dec 10.
- Greenblatt EE, Winkler T, Harris RS, et al. What causes uneven aerosol deposition in the bronchoconstricted lung? a quantitative imaging study. J Aerosol Med Pulm Drug Deliv. 2016;29:57–75.
- Darquenne C. Aerosol deposition in health and disease. J Aerosol Med Pulm Drug Deliv. 2012;25:140–147.
- Darquenne C, Fleming JS, Katz I, et al. Bridging the gap between science and clinical efficacy: physiology, imaging, and modeling of aerosols in the lung. J Aerosol Med Pulm Drug Deliv. 2016;29(2):107–126.
- Mairinger S, Hernández-Lozano I, Zeitlinger M, et al. Nuclear medicine imaging methods as novel tools in the assessment of pulmonary drug disposition. Expert Opin Drug Deliv. 2022;19:1561–1575.
- Man F, Tang J, Swedrowska M, et al. Imaging drug delivery to the lungs: methods and applications in oncology. Adv Drug Deliv Rev. 2023;192:114641.
- Bennett WD, Wu J, Fuller F, et al. Duration of action of hypertonic saline on mucociliary clearance in the normal lung. J Appl Physiol. 2015;118:1483–1490.
- Sadiq MW, Holz O, Ellinghusen BD, et al. Lung pharmacokinetics of inhaled and systemic drugs: a clinical evaluation. Br J Pharmacol. 2021;178:4440–4451.
- Kyriakoudi A, Pontikis K, Valsami G, et al. Pharmacokinetic characteristics of nebulized colistimethate sodium using two different types of nebulizers in critically ill patients with ventilator-associated respiratory infections. Antibiotics. 2022;11:1528.
- Karaiskos I, Souli M, Galani I, et al. Colistin: still a lifesaver for the 21st century? Expert Opin Drug Metab Toxicol. 2017;13:59–71.
- Sachdev A, Sagar N, Gupta D, et al. Efficacy of adjuvant colistimethate sodium nebulization in ventilator associated pneumonia in pediatric intensive care unit – a randomized controlled study. J Pediatric Crit Care. 2019;6:15.
- Pourheidar E, Haghighi M, Kouchek M, et al. Comparison of intravenous ampicillin-sulbactam plus nebulized colistin with intravenous colistin plus nebulized colistin in treatment of ventilator associated pneumonia caused by multi drug resistant Acinetobacter baumannii: randomized open label trial. Iranian J Pharm Res. 2019;18:269–281.
- Lu Q, Luo R, Bodin L, et al. Efficacy of high-dose nebulized colistin in ventilator-associated pneumonia caused by multidrug-resistant Pseudomonas aeruginosa and Acinetobacter baumannii. Anesthesiology. 2012;117:1335–1347.
- Gkoufa A, Sou T, Karaiskos I, et al. Pulmonary and systemic pharmacokinetics of colistin methanesulfonate (CMS) and formed colistin following nebulisation of CMS among patients with ventilator-associated pneumonia. Int J Antimicrob Agents. 2022;59:106588.
- Rashid J, Absar S, Nahar K, et al. Newer devices and improved formulations of inhaled insulin. Expert Opin Drug Deliv. 2015;12(6):917–928.
- Elnadoury O, Beattie J, Lubinsky AS. Uninterrupted continuous and intermittent nebulizer therapy in a COVID-19 patient using sequential vibratory mesh nebulizers: a case report. J Aerosol Med Pulm Drug Deliv. 2020;33:357–360.
- Li J, Augustynovich AE, Gurnani PK, et al. In-vitro and in-vivo comparisons of high versus low concentrations of inhaled epoprostenol to adult intubated patients. Respir Res. 2021;22:1–10.
- Drwiega EN, Rodvold KA. Penetration of antibacterial agents into pulmonary epithelial lining fluid: an update. Clin Pharmacokinet. 2022;61:17–46.
- Rodvold KA, George JM, Yoo L. Penetration of anti-infective agents into pulmonary epithelial lining Fluid. Clin Pharmacokinet. 2011;50:637–664.
- Kiem S, Schentag JJ. Interpretation of epithelial lining fluid concentrations of antibiotics against methicillin resistant Staphylococcus aureus. Infect Chemother. 2014;46:219.
- Mukker JK, Singh RSP, Derendorf H. Pharmacokinetic and pharmacodynamic implications in inhalable antimicrobial therapy. Adv Drug Deliv Rev. 2015;85:57–64.
- Desgrouas M, Ehrmann S. Inhaled antibiotics during mechanical ventilation—why it will work. Ann Transl Med. 2021;9:598.
- Asmus MJ, Stewart BA, Milavetz G, et al. Tobramycin as a pharmacologic tracer to compare airway deposition from nebulizers. Pharmacotherapy. 2002;22:557–563.
- Al-Amoud AI, Clark BJ, Assi KA, et al. Determination of the bioavailability of gentamicin to the lungs following inhalation from two jet nebulizers. Br J Clin Pharmacol. 2005;59:542–545.
- Moraine -J-J, Truflandier K, Vandenbergen N, et al. Placement of the nebulizer before the humidifier during mechanical ventilation: effect on aerosol delivery. Heart Lung. 2009;38:435–439.
- Dunne RB, Shortt S. Comparison of bronchodilator administration with vibrating mesh nebulizer and standard jet nebulizer in the emergency department. Am J Emerg Med. 2018;36:641–646.
- Moody GB, Luckett PM, Shockley CM, et al. Clinical efficacy of vibrating mesh and jet nebulizers with different interfaces in pediatric subjects with asthma. Respir Care. 2020;65:1451–1463.
- Mac Giolla Eain M, Joyce M, MacLoughlin R. An in vitro visual study of fugitive aerosols released during aerosol therapy to an invasively ventilated simulated patient. Drug Deliv. 2021;28:1496–1500.
- O’Toole C, McGrath JA, Joyce M, et al. Fugitive aerosol therapy emissions during mechanical ventilation: in vitro assessment of the effect of tidal volume and use of protective filters. Aerosol Air Qual Res. 2020;20:2604–2613.
- Fink JB, Ehrmann S, Li J, et al. Reducing aerosol-related risk of transmission in the era of COVID-19: an interim guidance endorsed by the International Society of Aerosols in Medicine. J Aerosol Med Pulm Drug Deliv. 2020;33:300–304.
- Cinesi Gómez C, Rodríguez Ó P, Luján Torné M, et al. Recomendaciones de consenso respecto al soporte respiratorio no invasivo en el paciente adulto con insuficiencia respiratoria aguda secundaria a infección por SARS-CoV-2. Arch Bronconeumol. 2020;56:11–18.
- Respiratory Care Committee of Chinese Thoracic Society. Expert consensus on preventing nosocomial transmission during respiratory care for critically ill patients infected by 2019 novel coronavirus pneumonia. Zhonghua Jie He He Hu Xi Za Zhi. 2020;43:288–296.
- British Thoracic Society. COVID-19: information for the respiratory community | British Thoracic Society | better lung health for all, https://www.brit-thoracic.org.uk/covid-19/covid-19-information-for-the-respiratory-community/ accessed 2022 Aug 2.
- Kumar P, Kulkarni AP, Govil D, et al. Airway management and related procedures in critically ill COVID-19 patients: position Statement of the Indian Society of Critical Care Medicine. Indian J Crit Care Med. 2020;24(8): 630–642 . DOI:10.5005/jp-journals-10071-23471.
- Federazione delle Associazioni dei Dirigenti Ospedalieri Internisti. COVID-19 | GUIDA CLINICO-PRATICA FADOI FADOI è orgogliosa di presentare la Guida Clinico-Pratica sull’infezione da SARS-CoV-2 in italiano, completa di bibliografia, a cura del Gruppo Giovani FADOI [cited 2023 Feb 01]. Available from:www.fadoi.org
- GOLD Science Committee. Global Strategy For the Diagnosis, Management and Prevention of Chronic Obstructive Pulmonary Disease - Report 2022. 2022. https://goldcopd.org/wp-content/uploads/2021/11/GOLD-REPORT-2022-v1.1-22Nov2021_WMV.pdf, accessed 2022 Nov 29.
- Craven DE, Lichtenberg DA, Goularte TA, et al. Contaminated medication nebulizers in mechanical ventilator circuits. Am J Med. 1984;77:834–838.
- Swanson CS, Dhand R, Cao L, et al. Microbiome profiles of nebulizers in hospital use. J Aerosol Med Pulm Drug Deliv. 2022;35:212–222.
- Monforte V, Román A, Gavaldà J, et al. Contamination of the nebulization systems used in the prophylaxis with amphotericin B nebulized in lung transplantation. Transplant Proc. 2005;37:4056–4058.