ABSTRACT
Introduction
Small airway disease (SAD) represents a common and critical feature of Chronic Obstructive Pulmonary Disease (COPD). Introduced in the ’60s, SAD has gradually gained increasing interest as assessment methodologies have improved. Chronic exposure to smoking and noxious particles or gases induces inflammation and remodeling, leading to airway obstruction and SAD, eventually resulting in complete airway loss.
Areas covered
A literature search up to June 2024 was performed in PubMed to identify articles on SAD and airway diseases mainly COPD, but also to the extent that it seemed relevant in the uncontrolled/severe asthma field, where SAD is better studied. We provide clinicians and translational scientists with a comprehensive analysis of the existing literature on SAD in COPD, concentrating on the underlying pathophysiological mechanisms, diagnostic techniques, and current pharmacological approaches targeting airflow obstruction in small airways.
Expert opinion
Small airways are the primary site for the onset and progression of airflow obstruction in patients with COPD, with significant clinical consequences associated with poor lung function, hyperinflation, and impaired quality of life. The early identification of individuals with subclinical SAD may allow us to prevent its further progress from airway loss and potential development of emphysema and choose the appropriate therapeutic approach.
1. Introduction
Chronic Obstructive Pulmonary Disease (COPD) constitutes a significant public health concern, with a global prevalence of approximately 400 million individuals and an estimated 3 million fatalities on an annual basis [Citation1,Citation2]. The current understanding is that COPD is a heterogeneous disorder characterized by chronic respiratory symptoms and persistent, often progressive airflow limitation due to abnormalities of the airways (bronchitis and bronchiolitis) and/or alveoli (emphysema), caused mainly by exposure to noxious gases or particles [Citation3].
The term’ small airway disease’ in COPD was first introduced in a study published in 1968 by Hogg et al. [Citation4]. This study reported a significant increase in small airway resistance among patients with emphysema, attributed to both narrowing and loss of the small airways due to pathological modifications such as inflammation, mucus plugging, and airway remodeling. In recent years, the presence of small airway disease (SAD) in COPD has been widely acknowledged to be associated with poor reversible airflow limitation and heightened symptom burden [Citation5], while several studies have identified SAD as a precursor of emphysema [Citation6,Citation7]. Notably, the prevalence of SAD increases with disease severity in COPD [Citation8,Citation9], suggesting that the condition is progressive, which warrants the beneficial effects of early detection and intervention.
Small airways are commonly referred to as the ‘silent zone’ of the lungs [Citation10]. Early abnormalities may be evident for years without being recognized, as the disorder becomes detectable by conventional spirometry only when the small airways are consistently damaged and obliterated [Citation11]. In accordance with this concept, the term ‘pre-COPD’ has been proposed as a potential precursor stage of COPD, which is characterized by physiological abnormalities and/or structural lung lesions (e.g. significant emphysema) observed on computed tomography (CT) in the absence of airflow obstruction [Citation12]. Recent work on small airways disease with the use of micro-CT indicates that the lungs of patients with emphysematous pre-COPD already show fewer small airways and airway remodeling, even in the absence of physiologic airway obstruction [Citation13], identifying small airways dysfunction as an early and important sign of the disease. In addition, patients with pre-COPD and Global Initiative for Chronic Obstructive Lung Disease (GOLD) 1–2 COPD stages exhibited more pronounced lung function decline compared with preserved ratio impaired spirometry (PRISm) and GOLD 3–4 patients [Citation14], emphasizing the clinical importance of SAD recognition in the implementation of earlier interventions.
Over the past two decades, there has been a growing interest in employing more sensitive methods of lung function assessment and high-resolution imaging techniques for evaluating and measuring SAD in COPD. These techniques have demonstrated significant advancements in accuracy and reliability compared with traditional assessment approaches [Citation15]. Technological advancements have facilitated the creation of pharmaceutical formulations and delivery systems that enable drug particles to reach both central and peripheral airways, aimed at improving therapeutic results [Citation16].
This narrative review aims to provide a comprehensive analysis of the existing literature on the influence of SAD on COPD, focusing on the underlying pathophysiological mechanisms, diagnostic techniques, and current pharmacological approaches targeting small airway obstruction. In addition, this review addresses potential future developments in the care and treatment of this disabling condition. Studies included in this article were identified by searching the PubMed (MEDLINE) database, using the search terms COPD AND (‘small airway disease’ OR ‘small airways disease’ OR ‘small airway dysfunction’ OR ‘small airways dysfunction’) and by cross-referencing citations in identified studies that were available in print or online up to June 2024 and selected on the basis of their relevance.
2.1. Definition-anatomy
Peripheral airways, also often referred to as small airways, are defined as conducting airways with a diameter of less than 2 mm, typically located from approximately the eighth generation of bronchi [Citation17]. Small airways encompass the terminal bronchioles (the smallest airways devoid of alveoli, which are merely conducting and do not engage in gas exchange), respiratory bronchioles (which occasionally contain alveoli), and the gas-exchange apparatus, comprising the alveolar ducts and alveolar sacs [Citation18] ().
Figure 1. The generations of the human bronchial tree. The branching structure of the airways commences at the level of the trachea, extending into the lungs as bronchi, bronchioles, and alveoli. The small airways, characterized by a diameter of less than 2 mm, typically emerge from approximately the seventh or eighth generation of bronchi. These small airways include the terminal bronchioles, which do not participate in gas exchange, as well as the respiratory bronchioles, which occasionally contain alveoli, and the gas-exchange area, encompassing the alveolar ducts and alveolar sacs. Created with BioRender.com.
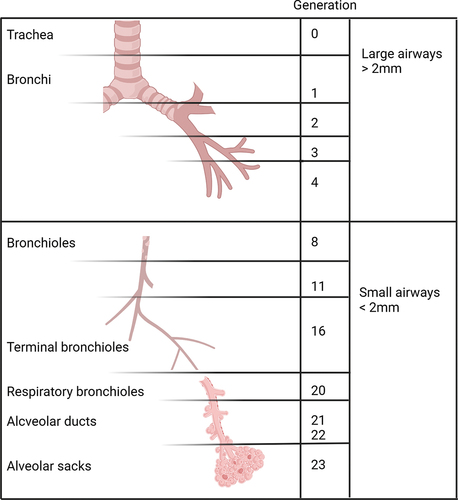
As the bronchioles divide, airways undergo a reduction in the length and diameter, as a result of which their cross-sectional area increases exponentially from ~2.5 cm2 at the level of the trachea to ~180 cm2 at the level of the terminal bronchioles and to nearly 300 cm2 at the level of the acinar level, which is as much as 90 times greater than that of the main bronchi [Citation19]. Therefore, despite their small lumen diameter, small airways eventually account for 98.8% (approximately 4500 ml) of the total lung volume [Citation20]. Accordingly, in normal lungs, their contribution to the overall airway resistance of the tracheobronchial tree is less than 10% [Citation16]. As a result of this anatomical structure, the airflow in terminal bronchioles is turbulent and characterized by high velocity, while it transitions to laminar and density-independent flow in the peripheral small airways [Citation21].
An additional notable physiological differentiation from large airways is that small airways exhibit little or no cartilaginous support [Citation22]. Given their narrow diameter and lack of cartilage, small airways are particularly susceptible to harm by pollutants, cigarette smoke, and other noxious agents, making them at risk of being the first and primary site of pathological response to inflammation, occlusion, or remodeling [Citation23]. In contrast, respiratory bronchioles are lined by a liquid with surfactant properties, which imparts a low surface tension, thereby protecting them from early closing at low volumes, particularly during expiration [Citation24].
2.2. Pathophysiology and inflammation
Accessing the small airways is challenging. However, several studies using various techniques have allowed us to understand the underlying mechanisms of inflammation. The critical elements of SAD underlying inflammation in COPD are airway remodeling, mucus plugging, immune cell infiltration, small airways loss, and emphysematous destruction [Citation25].
Remodeling is an inappropriate and extensive wound-healing response to inflammation caused by cigarette smoke, air pollution, bacteria, viruses, etc. Induced inflammation and subsequent immune responses lead to the activation of myofibroblasts and their interaction with the extracellular matrix.
Myofibroblasts originate from the sub-epithelial fibroblasts in interplay with the surrounding epithelial cells, forming epithelial-mesenchymal transition (EMT) and circulating fibrocytes [Citation26], which have been shown to increase in both distal and proximal tissue specimens of patients with COPD undergoing thoracic surgery [Citation27].
Extracellular matrix proteins, such as laminin and collagen, are increased in the small airways of COPD patients [Citation28]. Due to the excessive chronic inflammation and the limited regeneration capacity of the lung tissue, this process, even if initially aimed at wound repair, often leads to permanent changes in tissue architecture and remodeling [Citation25]. Historically, various immune cells have been associated with SAD in COPD, and several studies demonstrate extensive immune cell infiltration and underlying inflammation. These immune cells include macrophages, neutrophils, eosinophils, and B and T lymphocytes (both CD4 helper and CD8 cytotoxic), while there are reports on lymphoid follicles, as well [Citation29]. Notably, the number of T lymphocytes and cytotoxic CD8+ lymphocytes is associated with COPD severity [Citation30]. In lung biopsies of patients with COPD, an increased number of CD3 T lymphocytes, CD8 lymphocytes, and neutrophils relate to the presence of emphysema [Citation31]. Increased numbers of mast cells have also been found in the peripheral airways of patients with COPD, and these inflammatory cells correlate with lung function and centrilobular emphysema [Citation32]. Emerging data indicate that terminal bronchiolar alveolar attachments are the initial site of tissue destruction and centrilobular emphysema formation in early COPD; M1-like macrophages and neutrophils located within alveolar attachments are associated with the pathobiology of elastin fiber loss, whereas adaptive immune cells (naive, CD4, and CD8 T cells, and B cells) are related to evidence of terminal bronchiole wall remodeling [Citation33].
Increased mucus production and reduced clearance, leading to extensive mucus plugs, represent cardinal pathologic features of the small airways in patients with COPD. In line with this, cigarette smoking is associated with goblet cell hyperplasia and metaplasia in the small airways [Citation34]. Cigarette smoking reduces mucus clearance in small airways by shortening the length of ciliae [Citation35]. The result is an increase in mucin plugs in the small airways of patients with COPD, which is associated with early death in patients with severe emphysema who have undergone lung volume reduction surgery [Citation36].
Signs of inflammation in small airway disease, namely mucus gland hyperplasia and tissue remodeling, can potentially be linked to the susceptibility of COPD patients to frequent infections. Mucus contains antimicrobial peptides, such as IgA and defensins. However, it has been found that mucus in the small airways of patients with COPD contains low levels of secretory IgA, resulting in increased levels of 16S ribosomal RNA [Citation37]. Notably, the small airways in patients with stable COPD display an increased viral load [Citation38]. Even more extracellular matrix proteins participating in remodeling, such as laminin [Citation39] and collagen [Citation40], can bind to specific bacterial pathogens found in patients with COPD, such as non-typeable Haemophilus influenzae and Moraxella catarrhalis.
An interesting and less-studied population of patients with SAD is those exposed to biomass (i.e. organic matter, wood, animal waste, and forestry residues used as fuel). Several pathological changes have been observed in these patients, such as bronchial wall thickening, squamous cell metaplasia, goblet cell hyperplasia, peribronchial fibrosis, and bronchiectasis. Many of these patients are females, with typical deposition of anthracotic pigments [Citation41]. In an early study, biopsies from women with biomass exposure, with or without COPD, showed that women with COPD and biomass smoke exposure had more remodeling and fibrotic changes than women with COPD and tobacco smoke exposure [Citation42]. However, it remains to be clarified whether the differences observed are the consequence of severity of exposure, smoke composition, or both.
2.3. Assessment
Timely detection of small airway dysfunction in COPD continues to pose a critical challenge, as conventional techniques for evaluating small airways may be inadequate because of their small size and relative limited access [Citation43]. The currently available methods utilized to assess small airway dysfunction are presented below and summarized in . However, several of these methods are complex or invasive, necessitating the establishment of a consensus on the gold-standard approach.
Table 1. Currently available techniques for the assessment of small airways disease.
2.3.1. Spirometry
Spirometry is the gold standard for diagnosis and assessment of COPD [Citation12]. However, forced expiratory volume in 1 s (FEV1) primarily reflects abnormalities in the large airways and is often insufficient for evaluating expiratory flow in SAD [Citation22]. Moreover, FEV1 does not align with the histopathological features observed in early or mild COPD, nor does it relate to the severity of functional impairment and symptoms in individual patients [Citation44]. Interestingly, although FVC is not a direct marker of small airway dysfunction, the bronchodilator responsiveness of forced expiratory capacity (FVC) has been suggested as an indirect marker of more distal airway abnormalities [Citation45]. Similarly, the ratio of FEV1 to slow vital capacity (FEV1/SVC) is often used to assess small airway obstruction, as it has been shown to be more sensitive in detecting small airway dysfunction compared to the commonly used FEV1/FVC ratio in detecting small airway disease [Citation46]. Forced expiratory flow between 25% and 75% of the FVC (FEF25–75%) and maximal mid-expiratory flow (MMEF) have been employed in several studies as more reliable indirect markers of small airway obstruction [Citation47,Citation48]. MMEF has been used for screening patients with suspected early COPD who have clinical symptoms without detected persistent airflow limitation in pulmonary function examination [Citation49], suggesting the usefulness of the MMEF as an early indicator of small airway dysfunction in COPD. It is commonly accepted that small airway dysfunction occurs when two of the three indicators listed above fall below the expected value of 65% [Citation50]. However, further evidence is required to validate their reproducibility and specificity, as they are largely volume- and effort-dependent [Citation51]. Thus, the current guidelines do not support their use for routine assessment of SAD in clinical practice [Citation50]. The ratio of forced expiratory volumes in 3 and 6 s (FEV3/FEV6) has recently gained prominence as a more sensitive spirometric marker of SAD. Subjects presenting with a decreased FEV3/FEV6 ratio display increased gas air trapping and lung hyperinflation on computed tomography (CT) scans, greater symptom severity, and more pronounced functional decline than those with a ratio exceeding the lower limit of normality [Citation52,Citation53].
2.3.2. Body plethysmography
Body plethysmography is a noninvasive technique that enables the assessment of lung volumes, which are not directly measurable by conventional spirometric tests. Body plethysmography can be used to quantify functional parameters, including total lung capacity (TLC), functional residual capacity (FRC), inspiratory capacity (IC), and residual air volume (RV). These measurements offer precise and reliable information regarding lung hyperinflation and gas air-trapping, thereby reflecting probably SAD [Citation22]. It is generally accepted that an RV value greater than 120% or an RV/TLC ratio exceeding 40% of the predicted values are considered reliable markers of air trapping, irrespective of FEV1, particularly in the early stages of COPD [Citation54]. Furthermore, the degree of small airway inflammation and the radiologic extent of emphysema relate positively to FRC (%), RV (%), and RV/TLC parameters in patients with COPD [Citation30]. Body plethysmography may also be used to evaluate dynamic hyperinflation during exercise due to SAD in patients with mild COPD, and it has been shown that IC demonstrates a more robust relation to distance in the 6-min walk test (functional exercise capacity) than FEV1 [Citation55]. Thus, individuals with truly mild COPD (GOLD I) who experience respiratory symptoms during exercise may display low IC values and IC/TLC ratios [Citation56,Citation57].
2.3.3. Impulse oscillometry
Impulse oscillometry (IOS) is a noninvasive diagnostic test that evaluates the mechanical properties of the lungs by superimposing on the lung sound waves of varying frequencies (typically between 5 and 25 Hz) during normal tidal breathing and simultaneously recording the resulting flow and pressure changes at the mouth [Citation58]. The resistance and reactance signals at different frequencies are translated into the mechanical properties of the different lung regions. The resistance measured at 20 Hz, denoted as R20, indicates the resistance of the proximal airways, whereas the resistance measured at 5 Hz, referred to as R5, reflects the overall lung resistance. Thus, the contribution of small airways can be assumed from the difference between these two values (R5-R20) [Citation59]. Furthermore, the reactance measured at 5 Hz (X5) provides valuable information regarding the elastic recoil of small airways arising from the combined influence of tissue elasticity and inertance [Citation60]. Compared with spirometry, IOS is more sensitive than FEF25–75% in detecting SAD in patients with COPD [Citation61]. The severity of SAD estimated using this method increases with GOLD staging and demonstrates a substantial relationship to the extent of hyperinflation in CT and the magnitude of the symptom burden [Citation8]. Furthermore, it has been shown that IOS demonstrates greater sensitivity than spirometry in detecting SAD in smokers presenting with respiratory symptoms and preserved lung function [Citation62–64]. Given its simplicity and its relevant efficacy in detecting SAD at an early stage, IOS is now widely employed by numerous specialist centers in conjunction with spirometry for the diagnosis of high-risk individuals (e.g. smokers) or those with mild COPD.
The IOS technique is a user-friendly procedure that requires minimal cooperation from patients and is particularly suitable for older individuals and those with limited physical reserves and cognitive impairment. Despite its simplicity, the incorporation of this test in standard COPD management protocols presents certain limitations, including the requirement for a laboratory setting, significant intra-subject variability, and absence of established reference values [Citation65].
2.3.4. Nitrogen washout tests
The single-breath nitrogen test is a gas washout technique used to assess the vital capacity of the lungs [Citation66]. Following a single-breath inhalation of 100% oxygen, the test measures the volume of exhaled nitrogen toward the end of expiration, termed the closing volume (CV). This volume identifies the point at which the peripheral airways stop contributing to lung deflation, and is normally assumed to be 0.8 of the VC. The CV represents the commencement of phase IV in the flow-volume curve, whereas the slope of phase III (SIII) serves as an indicator of the ventilation distribution. An increased CV defines premature closure of the small airways caused by peripheral lung injury or dysfunction, which is responsible for air trapping [Citation67]. Furthermore, an increased SIII is related to poor homogeneity of ventilation and compromised gas exchange and is positively associated with FEV1%, RV/TLC, and total diffusion capacity to carbon monoxide (DLCO) [Citation68] ().
Figure 2. The single-breath nitrogen test. During the test, the subject maximally exhales to RV and then inhales a VC of 100% oxygen. From TLC, the subject slowly exhales back to RV while recording the nitrogen concentration on an X-Y axis. Four phases are typically identified: phase I represents pure dead space, phase II represents a combination of dead space and alveolar gas, phase III represents alveolar gas, and phase IV is known as the closing volume (CV). This volume identifies the point at which the peripheral airways stop contributing to lung deflation and is usually assumed to be approximately 0.8. An increased CV indicates premature closure of the small airways due to peripheral lung injury or dysfunction. In particular, the slope of phase III appears to be a sensitive indicator of early changes in the airways or lung parenchyma. An increased slope of phase III is associated with poor homogeneity of ventilation and compromised gas exchange. RV: residual volume. VC: vital capacity. TLC: total lung capacity. Created with BioRender.com.
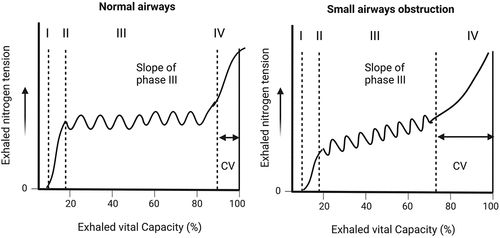
The multiple-breath washout technique is used to evaluate the sequential elimination of a tracer gas, commonly nitrogen or helium, following an initial inhalation of 100% oxygen through a sequence of multiple breaths until its concentration decreases to a fraction of its initial level [Citation69]. This method assesses the general efficiency of gas mixing within the lungs as the process of inert gas washout is impeded when the ventilation distribution becomes less uniform. Notably, multiple-breath washout techniques have demonstrated greater sensitivity than conventional spirometry in detecting SAD in patients with early or mild COPD [Citation46] and in smokers who display preserved spirometry [Citation52]. These methods also offer various additional advantages, as they involve minimal patient cooperation, their interpretation is independent of age, sex, and height, and they have shown reliable reproducibility in patients with stable disease [Citation70].
2.3.5. Imaging
Several imaging techniques are currently available to determine the extent, distribution, and progression of air-trapping indicative of SAD. CT imaging is the most widely studied method, even though the 1 mm high-resolution CT (HRCT) threshold does not allow for direct visualization of more distal airways. However, imaging abnormalities, such as centrilobular nodules, linear branching, mosaic attenuation, bronchiole obstruction, and air trapping, are commonly applied in clinical practice to assess the presence of SAD in patients with COPD [Citation71]. Additionally, several studies have progressively employed micro-CT to evaluate the presence of SAD [Citation72]. In a landmark study of lungs obtained from patients with various GOLD stages of COPD who were subjected to transplantation, the utilization of micro-CT revealed that the narrowing of small airways and the loss of terminal bronchioles occurred prior to the emergence of emphysema Mc [Citation6]. This research, although ex-vivo may provide an understanding of the increase in small airways resistance observed in individuals with early COPD, as opposed to healthy individuals.
The evaluation of distal airway and lung parenchymal function through hyperpolarized magnetic resonance imaging (HP-MRI) is another area of interest [Citation22]. The distribution of hyperpolarized gas (usually Helium or Xenon) may yield dynamic visualization of ventilation deficiency, reflecting airflow inhomogeneity due to locally impaired airway morphology. This technique may be employed to identify SAD in both current and former smokers with normal lung function [Citation73] and the presence of gas trapping due to SAD in patients with mild-to-moderate COPD [Citation74]. HP-MRI is also an effective and sensitive technique for establishing relationships between imaging metrics and functional parameters as well as for assessing treatment outcomes related to small airways [Citation75]. Additionally, the application of multiparametric response mapping, which integrates computed tomography (CT) and helium magnetic resonance imaging (MRI), represents a novel and highly sensitive approach for detecting small airway dysfunction and emphysema in former smokers with normal spirometry, independent of the results of CT scans alone [Citation76].
Other imaging techniques for evaluating small airways include scintigraphy, single-photon emission computed tomography (SPECT), positron emission tomography (PET), and functional respiratory imaging. These methods enable the visualization of structural abnormalities and regional deposition of radiolabeled tracers in the distal airways, which can be used to assess patterns of airflow limitation and drug deposition [Citation77,Citation78].
2.4. Biomarkers
A reliable, quantifiable, and clinically relevant biomarker would be of great value for evaluating SAD in patients with COPD. Despite the absence of a single biomarker that meets these criteria, recent findings on imaging, exhaled breath, bronchoalveolar lavage (BAL), sputum, and blood-based biomarkers may help address some of the current gaps [Citation79].
Regarding imaging, the application of a novel machine-learning modeling approach in individuals with various COPD stages from the COPDGene study unveiled two pathways of disease progression: a ‘Tissue to airway’ subtype (70.4%), in which air-trapping and emphysema preceded large airways abnormalities and an ‘Airway to tissue’ subtype (29.6%), wherein the opposite occurred [Citation80]. This study recognized two different disease subtypes and identified individuals at risk of developing COPD and highlighted the technique’s potential as a biomarker for early COPD. Recent investigations imply that integrating parametric image response mapping with CT can evaluate small airways at the voxel level, facilitating the earlier identification of individuals at risk of emphysema progression, including those with normal spirometry [Citation81,Citation82].
Fractional exhaled nitric oxide (FeNO) is primarily produced in the bronchial epithelium via inducible nitric oxide synthase (iNOS), and has garnered significant clinical interest as a biomarker of type 2 airway inflammation in asthma [Citation83]. Similar to asthma, FeNO levels may increase in stable COPD patients with evidence of eosinophilic inflammation, although to a lesser degree than in asthma [Citation84]. High FeNO levels have also been linked to an increased risk of acute COPD exacerbation [Citation85] and are predictive of corticosteroid responsiveness [Citation86]. The correlation between FeNO levels and airway eosinophilia in COPD is variable and modest, as current smoking, inhaled corticosteroid (ICS) use, and bacterial infections can decrease FeNO [Citation87]. However, in patients with SAD, FeNO modeling appears to be more sensitive in detecting eosinophilic inflammation and in predicting the risk of lung function decline and response to corticosteroids during acute exacerbation [Citation88,Citation89].
Although BAL provides a robust representation of the small airways, its invasive nature limits its practical application. In contrast, sputum samples, whether induced or spontaneous, may stand as a potential alternative source of biomarkers for small airways dysfunction because of their feasible collection and strong association with clinical parameters [Citation90]. Several inflammatory, oxidative stress, and epithelial damage markers, including cellular cell (CC)-16 protein, soluble receptor for advanced glycation end products (sRAGE), matrix metalloproteases (MMP) –8, –9, and –12, neutrophil elastase, and eosinophil peroxidase, have been investigated in BAL and sputum samples from smokers with or without COPD [Citation91–94]. Although elevated levels of these biomarkers were found to be closely associated with the presence of SAD, additional research is necessary to confirm their site of generation and to establish their clinical utility, validate their diagnostic and predictive value, and optimize generally accepted sample collection and processing protocols. Similarly, although serum biomarkers such as C-reactive protein (CRP), fibrinogen, sRAGE, and interleukin (IL)-6 have been investigated in several cohorts of COPD patients, they are not largely related to small airway inflammatory processes and are highly confounded by other systemic and certain local comorbidities, including infections and idiopathic pulmonary fibrosis [Citation95].
Although neutrophilic airway inflammation is a frequently observed characteristic in patients with COPD, some studies have underlined the potential involvement of eosinophilic airway inflammation in a subset of these patients [Citation96]. A study of bronchial biopsies taken from symptomatic smokers with or without COPD and sputum samples collected during exacerbations have demonstrated increased numbers of infiltrating eosinophils in the small airways and significant associations with blood eosinophil counts compared to never-smokers [Citation97,Citation98]. Although eosinophilic inflammation is heterogeneous among COPD patients, recent evidence has shown that airway eosinophilia is frequently observed in early COPD and can be detected in approximately 20% of these patients, even in the absence of concurrent asthma [Citation99]. The results of this study imply that targeting airway eosinophilia in a subgroup of eosinophilic COPD patients may have a favorable impact on small airway dysfunction and patients’ symptoms.
2.5. Clinical importance
The available evidence indicates that the small airways are the primary site for the onset and progression of airflow obstruction in susceptible smokers [Citation28]. Both preventable and treatable, SAD may have important clinical consequences associated with poor lung function, hyperinflation, and impaired quality of life, if not addressed [Citation43]. Therefore, early identification of individuals with subclinical airflow limitation and elucidation of the underlying pathological mechanisms responsible for airway remodeling and accumulation of inflammatory exudates represent promising targets for therapeutic interventions [Citation100].
Therefore, detection of pre-obstruction SAD is crucial. Although small airways contribute little to airflow resistance in normal lungs, they have been recognized as major component of airflow limitation in patients with COPD, resulting in symptoms that may be present even before overt airway obstruction is detectable using conventional spirometry techniques [Citation101]. Consequently, if SAD is identified early, implementing treatments designed to target small airways may be beneficial in reducing symptoms and enabling patients to maintain their daily activities, improve overall COPD management and progression, and enhance their quality of life [Citation43].
In addition, there is an urgent need to determine whether small airway dysfunction is associated with significant clinical outcomes such as mortality, exacerbation rate, long-term lung function, and quality of life. However, normal ranges for healthy individuals and minimal clinically significant differences need to be established before this can be accomplished. Furthermore, longitudinal studies are required to determine the usefulness of detecting the progression of small airway disease over time.
2.6. Treatment
During the last years, small airways have been a treatment target for novel aerosolized drug formulations designed to penetrate the periphery of the bronchial tree better than conventional ones. The size of the inhaled particles is the main factor that determines the deposition of the drug in the airways, together with other factors, such as the duration and speed of the inhaled aerosol, while the lipophilic/hydrophilic pattern of the drug molecule influences absorption [Citation102,Citation103]. Small particles with a mass mean aerodynamic diameter (MMAD) less than 2,1 µm are classified as extra-fine and found radiologically to reach the small airways, while particles with an MMAD greater than 5 µm are classified as coarse and are mainly deposited in the oropharynx, trachea, and larger airways [Citation104].
Following the outlawing of chlorofluorocarbons (CFC), numerous studies have focused on the development of novel propellants, drug formulations and inhaler devices. Aerosol formulations containing hydrofluoroalkanes (HFA) have emerged as promising alternative propellant molecules, demonstrating greater peripheral lung deposition than those containing CFC [Citation105]. Drug formulations can be inhaled via pressurized metered-dose inhalers (pMDIs), dry powder inhalers (DPIs), and soft mist inhalers (SMIs). Conventional pMDIs and the majority of DPIs produce drug particles that are too large to effectively reach small airways [Citation16]. Respimat ®SMI is used for inhalation of the long-acting muscarinic antagonist (LAMA) tiotropium as well as the ultralong-acting β2–2-agonist (LABA) olodaterol alone or in combination (LAMA/LABA). This is of importance as studies on rat and human small airways have shown that tiotropium effectively inhibits cholinergic contraction, suggesting that M3 muscarinic acetylcholine receptor antagonists primarily inhibit small airway contraction [Citation106].
The fine particle fraction for this SMI is almost double that generated by DPIs, resulting in better drug deposition to the lung periphery and small airways [Citation107–109].
In the past few years, the development of particle formulations with a diameter smaller than 1.5 µm has been prominent [Citation103,Citation110]. Specifically, an extra-fine combination of beclomethasone dipropionate and formoterol (BDP/F) administered via pressurized metered dose inhaler (pMDI) has been introduced to the drug market [Citation105]. This ultrafine solution facilitates the delivery of pharmaceutical particles to both the central and more peripheral airways, thereby maximizing the effect of treatment by increasing peripheral lung deposition compared to the initial dose, while simultaneously decreasing systemic exposure [Citation111]. Furthermore, pMDI does not mandate a profound inspiration for the effective distribution of the drug in the airways due to the presence of the HFA propellant. However, deep inhalation is crucial when dry powder inhalers are used. It is a common practice in clinical settings not to suggest dry powder inhalers (DPIs) to patients who are unable to administer them effectively [Citation112].
For patients who require ICS in addition to bronchodilator therapy, it may be beneficial to target the small airways with extra-fine particle formulations. In a study of patients with severe COPD and a history of exacerbations, extra-fine beclomethasone/formoterol significantly reduced the exacerbation rate and improved lung function compared with formoterol alone [Citation113]. Similarly, in another study, the combination of extra-fine beclomethasone/formoterol significantly reduced air trapping and dyspnea in COPD patients with lung hyperinflation when compared to fluticasone/salmeterol [Citation114]. These studies do not provide sufficient data to determine whether the superior impact on air trapping resulting from the beclomethasone/formoterol combination is attributable to formoterol, to beclomethasone, or to the extra-fine formulation’s ability to reach the small airways. Nevertheless, research has demonstrated that formoterol exhibits a more potent intrinsic efficacy in reversing the carbachol-induced contraction in human small airways when compared to salmeterol [Citation115]. This could support the explanation that the greater efficacy of the extra-fine beclomethasone/formoterol combination may be attributed to its potential pharmacological activity on the small airways.
Another fixed triple combination therapy with beclomethasone, glycopyrronium, and formoterol using extra-fine particles has been evaluated in large clinical trials, demonstrating a significant reduction in COPD exacerbations compared to single or dual bronchodilation therapy, as well as ICS/LABA combination [Citation116–118]. A previous study in patients with moderate to severe COPD GOLD stages 2–4 showed that treatment with a low dose of extra-fine beclomethasone was as effective as treatment with higher doses of coarse fluticasone in reducing exacerbations and was associated with better odds of stability during a two-year follow-up period [Citation119]. Lower doses of ICS achieved with extra-fine particle treatment are also correlated with a lower risk of potential treatment side effects, especially pneumonia in COPD [Citation120]. Another study using functional respiratory imaging (FRI) computer simulations in patients with moderate to severe COPD found that the peripheral airway deposition of an extra-fine combination of beclomethasone/glycopyrronium/formoterol was superior to non-extra-fine formulations of fluticasone furoate/vilanterol/umeclidinium, supporting the better deposition of extra-fine particles in the small airways [Citation121].
The combination of glycopyrronium and formoterol fumarate (GFF) is the only LAMA/LABA drug formulation available through a pMDI using the Aerosphere inhaler. GFF has shown efficacy in COPD in improving lung function and inspiratory capacity (IC) with deposition in the large and small airways [Citation122–124]. GFF is combined with inhaled corticosteroid (ICS) budesonide in a single Aerosphere inhaler for patients who require ICS, and an open-label gamma-scintigraphy study demonstrated that the drug can be successfully deposited in the small airways of patients with moderate and severe COPD [Citation125].
In conclusion, recent advances in inhaled drug delivery have enhanced the delivery of aerosolized medicine to the small airways. Nevertheless, it is essential to recognize that available research on the advantages of drug delivery to small airways in terms of patient-centered outcomes is limited. The different therapeutic options with the potential to target the small airways and their mechanisms of action in the lungs are summarized in .
Table 2. The currently available therapeutic options with the potential to target the small airways and their mechanisms of action in the lungs.
N-acetyl cysteine (NAC) has a mucolytic and antioxidant effect and has been associated with a reduction in exacerbations and symptom improvement in COPD [Citation126]. In one study, a high dose of NAC with 600 mg bid was associated with a significant improvement of FEF25–75% of 0,12 L/s compared to placebo as well as a significant improvement in forced oscillation technique measurements indicating an effect in the small airways [Citation127].
Treatment with dupilumab, a fully human monoclonal antibody against the shared receptor for interleukin-4 and interleukin-13, is the first biologic to demonstrate efficacy in reducing exacerbations and improving lung function in COPD patients with type 2 inflammation as indicated by elevated blood eosinophils [Citation108,Citation128]. In this study and previous studies on severe asthma, dupilumab significantly improved FEF25–75% compared with placebo [Citation129,Citation130].
3. Future steps
Earlier and ongoing research has identified the critical role of the small airways in the pathology and clinical symptoms of patients with COPD. This suggests the need for early recognition to facilitate early intervention and treatment.
It may be beneficial to improve and make methodologies for the early assessment of SAD easily accessible. This includes both physiological- and imaging-based approaches. There is an unmet need for the standardization of spirometry-based measurements and their incorporation, even in portable spirometers, to be readily available for all respiratory physicians and patients, notably in less developed countries where air pollution and biomass represent the main factors for COPD, especially among women. To accomplish the early detection and follow-up of SAD in patients with COPD, we also need to develop sensitive and specific biomarkers for SAD in COPD.
To achieve this, we need to further study the underlying immunopathology of SAD in COPD patients. As seen from the literature in this and similar reviews, there is a gap in our understanding and immunophenotyping of immune cells. Most studies have been conducted when the methodology does not allow immunophenotyping. Future studies should include small airway biopsies from patients with SAD, where methodologies such as spatial transcriptomics and single-cell sequencing can be applied to evaluate the underlying immunopathology.
Of course, upon its recognition, the right therapy must be applied to address SAD. The development of an appropriate inhaled drug delivery system that can reach and deposit in small airways to address inflammation is a critical area for future research. This area includes both drug and inhalation devices development, and notably, a novel approach where patient-specific characteristics such as individual anatomy, breathing pattern, and inhalation technique will be added to the development of a customized therapeutic approach leading to personalized medicine. An area where AI development may be valuable.
4. Conclusion
SAD represents a cardinal feature of COPD that develops early in disease progression. Small airway anatomy and physiological features, together with the characteristics of noxious particles and gases such as smoking, air pollution, and biomass, lead to early inflammation that starts and occurs before the classic symptoms of COPD. Upon its development, it is linked to hyperinflation and, consequently, to exercise-induced dyspnea, a very concerning symptom in patients with COPD, affecting patients’ quality of life. Eventually, SAD leads to the loss of terminal airways and development of emphysema.
The development of SAD is also linked to mucus dysfunction, which further induces respiratory symptoms and reduces quality of life. Furthermore, data suggest a link between microbial dysbiosis and the sensibility of airway infections, often seen in patients with COPD, leading to exacerbations and hospitalizations, and eventually increased morbidity and mortality.
5. Expert opinion
There is an immediate need to recognize SAD early and address it in patients with COPD, even in the very early stages. This is a task for medical practitioners who encounter patients with respiratory symptoms and risk factors of COPD.
Small airways dysfunction and emphysema are linked to a decline in FEV1, especially in individuals with mild-to-moderate COPD [Citation131]. SAD has been found in patients with pre-COPD in the absence of spirometric airway obstruction but with the presence of emphysema [Citation13]. Small airway dysfunction seems to precede the development of emphysema, making it a prime target for the development of preventative treatments that can address the progression of both airway and parenchymal disease [Citation132]. Thus, for each patient with a clinical history indicating COPD, even with an FEV1/FVC ratio > 0.70, a full assessment with an evaluation of SAD should be performed. Patients with Preserved ratio impaired spirometry (PRISm) also have a higher risk of SAD [Citation133]. Thus, we should include the assessment of SAD in all patients under evaluation for COPD, notably in those with the absence of conventional airway obstruction, that is, FEV1/FVC ratio > 0.7.
A special category of patients that address the presence of SAD is those with unexpected dyspnea despite conventional normal spirometry, a challenging group of patients who often seek help, and we have difficulties to address [Citation134]. Many of these patients are at a risk of developing COPD. Because of the potential for extensive damage and obliteration of the small airways, which may occur before detection with conventional spirometric tests, specialized tests have been developed to detect early abnormalities and to potentially prevent or delay disease progression. However, traditional imaging techniques may not be sufficiently sensitive to identify the early stages of small airways disease or to differentiate between the contribution of small airways disease and emphysema [Citation11]. In addition, many methods for evaluating the small airways are either intricate or invasive, and currently, there is no universally accepted approach.
Nevertheless, considering the evaluation of SAD in patients with clinical signs and symptoms of COPD, even without airway obstruction, is an important first step in recognizing the presence of SAD. Upon its presence, we should follow it carefully and customize the given therapy for each individual. This is the first and most crucial step in helping parents tackle the development of SAD and, eventually, emphysema.
The potential role of treatments that can reach the lung periphery and increase lung deposition may be a viable option for patients with these early signs of COPD. In fact, the concept of ‘early COPD’ has gained significant importance in the management of the disease, as emphasized by recent guidelines [Citation12]. Small airways appear to constitute the primary site of airway damage and are challenging diagnostic and pharmacological targets, because of their unique anatomy and physiology, as well as our current limited ability to detect early disease. However, the extent of the clinical benefit provided by inhaled therapy for SAD is limited. While some studies suggest that treatment with novel drug formulations, inhalers, and inhalation devices may offer symptomatic relief and reduce exacerbation rates, the specific initial and long-term effects on lung function decline and mortality remain unclear. A better understanding of the causes of early airflow limitation leading to COPD could enable the development of more personalized strategies for managing SAD and preventive treatments that address the underlying pathological processes.
Article highlights
Peripheral airways, also called small airways, are defined as conducting airways with a diameter of less than 2 mm.
Small airways encompass the terminal bronchioles, respiratory bronchioles, and the gas-exchange apparatus comprising the alveolar ducts and alveolar sacs.
Small airways possess a substantial total cross-sectional area of approximately 300 cm2 at the acinar level, which is 90 times larger than that of the main bronchi.
Small airway assessment includes lung function-based and imaging techniques.
Functional techniques include spirometry, with FEV1, FEF25–75%, MMEF, FEV1/SVC, and FEV3/FEV6 as small airway-related parameters; body plethysmography, with FRC%, RV%, and RV/TLC ratio as small airway-related parameters; Impulse Oscillometry, with R5-R20 as the main small airway-related parameter; and Single-breath Nitrogen test and multiple-breath washout techniques.
Imaging techniques include high-resolution CT (HRCT)/micro-CT, hyperpolarized gas magnetic resonance imaging (MRI), Scintigraphy, Single-photon emission computed tomography (SPECT), positron emission tomography (PET), and Functional Respiratory Imaging (FRI).
The critical elements of SAD underlying inflammation in COPD are airway remodeling, mucus plugging, and immune cell infiltration, affecting even bacterial flora.
SAD is the primary site for the onset and progression of airflow obstruction in patients with COPD, and is associated with poor lung function, hyperinflation, and impaired quality of life.
In patients with COPD and SAD, adjustment of inhaled therapy based on smaller particles and appropriate inhaler devices should be the treatment strategy.
Declaration of interest
N Lazarinis reports personal fees (honoraria from AstraZeneca, Chiesi, Sanofi, GSK, and advisory board from AstraZeneca) outside the submitted work. E Fouka reports personal fees (honoraria from AstraZeneca, Boehringer Ingelheim, Chiesi, ELPEN, Specialty Therapeutics, GSK, and Menariri) and support for attending meetings and/or travel (GSK, Menarini, AstraZeneca, Chiesi) outside the submitted work. A Bossios reports a grant from AstraZeneca and lecture fees from Chiesi paid to his institution outside the submitted work. The authors have no other relevant affiliations or financial involvement with any organization or entity with a financial interest in or financial conflict with the subject matter or materials discussed in the manuscript apart from those disclosed.
Reviewer disclosures
Peer reviewers on this manuscript have no relevant financial or other relationships to disclose.
Additional information
Funding
References
- Adeloye D, Song P, Zhu Y, et al. Global, regional, and national prevalence of, and risk factors for, chronic obstructive pulmonary disease (COPD) in 2019: a systematic review and modelling analysis. The Lancet Respir Med. 2022;10(5):447–458. doi: 10.1016/S2213-2600(21)00511-7
- Li X, Cao X, Guo M, et al. Trends and risk factors of mortality and disability adjusted life years for chronic respiratory diseases from 1990 to 2017: systematic analysis for the global burden of disease study 2017. BMJ. 2020 Feb 19 ;368:m234. doi: 10.1136/bmj.m234 Erratum in: BMJ. 2020 Aug 6;370:m3150. doi: 10.1136/bmj.m3150. PMID: 32075787; PMCID: PMC7190065.
- Agustí A, Celli BR, Criner GJ, et al. Global initiative for chronic obstructive lung disease 2023 Report: GOLD executive summary. Eur Respir J. 2023;61(4):2300239. doi: 10.1183/13993003.00239-2023
- Hogg JC, Macklem PT, Thurlbeck WM. Site and nature of airway obstruction in chronic obstructive lung disease. The N Engl J Med (Print). 1968;278(25):1355–1360. doi: 10.1056/NEJM196806202782501
- Hogg JC, McDonough JE, Suzuki M. Small airway obstruction in COPD: new insights based on micro-CT imaging and MRI imaging. Chest. 2013;143(5):1436–1443. doi: 10.1378/chest.12-1766
- McDonough JE, Yuan R, Suzuki M, et al. Small-airway obstruction and emphysema in chronic obstructive pulmonary disease. N Engl J Med. 2011;365(17):1567–1575. doi: 10.1056/NEJMoa1106955
- Koo H-K, Vasilescu DM, Booth S, et al. Small airways disease in mild and moderate chronic obstructive pulmonary disease: a cross-sectional study. The Lancet Respir Med. 2018;6(8):591–602. doi: 10.1016/S2213-2600(18)30196-6
- Crisafulli E, Pisi R, Aiello M, et al. Prevalence of small-airway dysfunction among COPD patients with different GOLD stages and its role in the Impact of disease. Respiration. 2017;93(1):32–41. doi: 10.1159/000452479
- Ritchie AI, Donaldson GC, Hoffman EA, et al. Structural predictors of lung function decline in young smokers with normal spirometry. Am J Respir Crit Care Med. 2024;209(10):1208–1218. doi: 10.1164/rccm.202307-1203OC
- Mead J. The lung’s “quiet zone”. N Engl J Med (Print). 1970;282(23):1318–1319. doi: 10.1056/NEJM197006042822311
- Hogg JC, Paré PD, Hackett T-L. The contribution of small airway obstruction to the pathogenesis of chronic obstructive pulmonary disease. Physiol Rev. 2017;97(2):529–552. doi:10.1152/physrev.00025.2015
- 2024 GOLD report - global initiative for chronic obstructive lung disease - GOLD (goldcopd.Org). 2024.
- Verleden SE, Hendriks JMH, Snoeckx A, et al. Small airway disease in pre-chronic obstructive pulmonary disease with emphysema: a cross-sectional study. Am J Respir Crit Care Med. 2024;209(6):683–692. doi: 10.1164/rccm.202301-0132OC
- Agustí A, Hughes R, Rapsomaki E, et al. The many faces of COPD in real life: a longitudinal analysis of the NOVELTY cohort. ERJ Open Res. 2024;10(1):00895–2023. doi: 10.1183/23120541.00895-2023
- Zhang Y, Zhang H, Su X, et al. Analysis of influencing factors and a predictive model of small airway dysfunction in adults. BMC Pulm Med. 2023;23(1):141. doi: 10.1186/s12890-023-02416-5
- Lavorini F, Pedersen S, Usmani OS. Confusion, and misconceptions related to small airways directed therapy. Chest. 2017;151(6):1345–1355. doi: 10.1016/j.chest.2016.07.035
- Weibel ER. Morphometry of the human lung. New York (NY): Acedemic Press Inc; 1963.
- Hyde DM, Hamid Q, Irvin CG. Anatomy, pathology, and physiology of the tracheobronchial tree: emphasis on the distal airways. J Allergy Clin Immunol. 2009;124(6 Suppl):S72–S77. doi: 10.1016/j.jaci.2009.08.048
- Baker DJ. The structure of the airways and lungs. In: Artificial ventilation: a basic ClinicalGuide. Springer International Publishing; 2020. p. 27–42.
- Virchow JC. Asthma–a small airway disease: concepts and evidence. Pneumologie. 2009;63(Suppl 2):S96–101. doi: 10.1055/s-0029-1214715
- Weibel ER, Sapoval B, Filoche M. Design of peripheral airways for efficient gas exchange. Respir Physiol & Neurobiol. 2005;148(1–2):3–21. doi:10.1016/j.resp.2005.03.005
- McNulty W, Usmani OS. Techniques of assessing small airways dysfunction. Eur Clin Respir J. 2014;1(1):25898. doi: 10.3402/ecrj.v1.25898
- Cosio MG, Hale KA, Niewoehner DE. Morphologic and morphometric effects of prolonged cigarette smoking on the small airways. Am Rev Respir Dis. 1980;122(2):265–221. doi: 10.1164/arrd.1980.122.2.265
- Macklem PT, Proctor DF, Hogg JC. The stability of peripheral airways. Respiration Physiol. 1970;8(2):191–203. doi:10.1016/0034-5687(70)90015-0
- Higham A, Quinn AM, Cançado JED, et al. The pathology of small airways disease in COPD: historical aspects and future directions. Respir Res. 2019;20(1):49. doi:10.1186/s12931-019-1017-y
- Florez-Sampedro L, Song S, Melgert BN. The diversity of myeloid immune cells shaping wound repair and fibrosis in the lung. Regeneration. 2018;5(1):3–25. doi:10.1002/reg2.97
- Dupin I, Thumerel M, Maurat E, et al. Fibrocyte accumulation in the airway walls of COPD patients. Eur Respir J. 2019;54(3):1802173. doi: 10.1183/13993003.02173-2018
- Singh D, Long G, Cançado JED, et al. Small airway disease in chronic obstructive pulmonary disease: insights and implications for the clinician. Curr Opin Pulm Med. 2020;26(2):162–168. doi:10.1097/MCP.0000000000000637
- Olloquequi J, Ferrer J, Montes JF, et al. Differential lymphocyte infiltration in small airways and lung parenchyma in COPD patients. Respir Med. 2010;104(9):1310–1318. doi: 10.1016/j.rmed.2010.03.002
- Turato G, Zuin R, Miniati M, et al. Airway inflammation in severe chronic obstructive pulmonary disease: relationship with lung function and radiologic emphysema. Am J Respir Crit Care Med. 2002;166(1):105–110. doi: 10.1164/rccm.2111084
- Majo J, Ghezzo H, Cosio MG. Lymphocyte population and apoptosis in the lungs of smokers and their relation to emphysema. Eur Respir J. 2001;17(5):946–953. doi: 10.1183/09031936.01.17509460
- Gosman MME, Postma DS, Vonk JM, et al. Association of mast cells with lung function in chronic obstructive pulmonary disease. Respir Res. 2008;9(1):64. doi: 10.1186/1465-9921-9-64
- Booth S, Hsieh A, Mostaco-Guidolin L, et al. A single-cell atlas of small airway disease in chronic obstructive pulmonary disease: a cross-sectional study. Am J Respir Crit Care Med. 2023;208(4):472–486. doi: 10.1164/rccm.202303-0534OC
- Hogg JC, Chu F, Utokaparch S, et al. The nature of small-airway obstruction in chronic obstructive pulmonary disease. N Engl J Med. 2004;350(26):2645–2653. doi: 10.1056/NEJMoa032158
- Leopold PL, O’Mahony MJ, Lian XJ, et al. Smoking is associated with shortened airway cilia. PLOS ONE. 2009;4(12):e8157. doi: 10.1371/journal.pone.0008157
- Hogg JC, Chu FSF, Tan WC, et al. Survival after lung volume reduction in chronic obstructive pulmonary disease: insights from small airway pathology. Am J Respir Crit Care Med. 2007;176(5):454–459. doi: 10.1164/rccm.200612-1772OC
- Polosukhin VV, Richmond BW, Rui-Hong Du. Secretory IgA deficiency in individual small airways is associated with persistent inflammation and remodeling. Am J Respir Crit Care Med. 2017;195(8):1010–1021. doi: 10.1164/rccm.201604-0759OC
- Utokaparch S, Sze MA, Gosselink JV, et al. Respiratory viral detection and small airway inflammation in lung tissue of patients with stable, mild COPD. J Chronic Obstr Pulm Disease. 2014;11(2):197–203. doi: 10.3109/15412555.2013.836166
- Jalalvand F, Su Y-C, Mörgelin M, et al. Haemophilus influenzae protein F mediates binding to laminin and human pulmonary epithelial cells. J Infect Dis. 2013;207(5):803–813. doi: 10.1093/infdis/jis754
- Abdillahi SM, Bober M, Nordin S, et al. Collagen VI is upregulated in COPD and serves both as an adhesive target and a bactericidal barrier for Moraxella catarrhalis. J Innate Immun. 2015;7(5):506–517. doi: 10.1159/000381213
- Ramírez-Venegas A, Torres-Duque CA, Guzmán-Bouilloud NE, et al. Smalla airway disease in copd associated to biomass exposure. Rev Invest Clin. 2019;71(1):70–78. doi: 10.24875/RIC.18002652
- Rivera RM, Cosio MG, Ghezzo H, et al. Comparison of lung morphology in COPD secondary to cigarette and biomass smoke. Int J Tuberc Lung Dis. 2008;12(8):972–977.
- Usmani OS, Dhand R, Lavorini F, et al. Why we should target small airways disease in our management of chronic obstructive pulmonary disease. Mayo Clinic Proc. 2021;96(9):2448–2463. doi: 10.1016/j.mayocp.2021.03.016
- Elbehairy AF, Parraga G, Webb KA, et al. Mild chronic obstructive pulmonary disease: why spirometry is not sufficient! Expert Rev Respir Med. 2017;11(7):549–563. doi:10.1080/17476348.2017.1334553
- Chetta A, Pisi R, Aiello M, et al. Small airway dysfunction and flow and volume bronchodilator responsiveness in patients with chronic obstructive pulmonary disease. Int J Chron Obstruct Pulmon Dis. 2015;10:1191–1197. doi: 10.2147/COPD.S82509
- Saint-Pierre M, Ladha J, Berton DC, et al. Is the slow vital capacity clinically useful to uncover airflow limitation in subjects with preserved FEV1/FVC ratio? Chest. 2019;156(3):497–506. doi: 10.1016/j.chest.2019.02.001
- McFadden ER, Linden DA. A reduction in maximum mid-expiratory flow rate. A spirographic manifestation of small airway disease. Am J Med. 1972;52(6):725–737. doi:10.1016/0002-9343(72)90078-2
- Stockley JA, Ismail AM, Hughes SM, et al. Maximal mid-expiratory flow detects early lung disease in α1-antitrypsin deficiency. Eur Respir J. 2017;49(3):1602055. doi: 10.1183/13993003.02055-2016
- Morris ZQ, Coz A, Starosta D. An isolated reduction of the FEV3/FVC ratio is an indicator of mild lung injury. Chest. 2013;144(4):1117–1123. doi:10.1378/chest.12-2816
- Graham BL, Steenbruggen I, Miller MR, et al. Standardization of spirometry 2019 update. An official American thoracic society and European respiratory society technical statement. Am J Respir Crit Care Med. 2019;200(8):e70–e88. doi: 10.1164/rccm.201908-1590ST
- Bonini M, Usmani OS. The role of the small airways in the pathophysiology of asthma and chronic obstructive pulmonary disease. Ther Adv Respir Dis. 2015;9(6):281–293. doi:10.1177/1753465815588064
- Dilektasli AG, Porszasz J, Casaburi R, et al. A novel spirometric measure identifies mild COPD unidentified by standard criteria. Chest. 2016;150(5):1080–1090. doi: 10.1016/j.chest.2016.06.047
- Stringer WW, Porszasz J, Bhatt SP, et al. Physiologic insights from the COPD genetic epidemiology study. Chronic Obstr Pulm Dis. 2019;6(3):256–266. doi: 10.15326/jcopdf.6.3.2019.0128
- Glaab T, Vogelmeier C, Buhl R. Outcome measures in chronic obstructive pulmonary disease (COPD): strengths and limitations. Respir Res. 2010;11(1):79. doi:10.1186/1465-9921-11-79
- D’Ascanio M, Viccaro F, Calabrò N, et al. Assessing static lung hyperinflation by whole-body plethysmography, helium dilution, and impulse oscillometry system (IOS) in patients with COPD. Int J Chron Obstruct Pulmon Dis. 2020;15:2583–2589. doi: 10.2147/COPD.S264261
- Celli BR, Cote CG, Marin JM, et al. The body-mass index, airflow obstruction, dyspnea, and exercise capacity index in chronic obstructive pulmonary disease. N Engl J Med. 2004;350(10):1005–1012. doi: 10.1056/NEJMoa021322
- Marin JM, Carrizo SJ, Gascon M, et al. Inspiratory capacity, dynamic hyperinflation, breathlessness, and exercise performance during the 6-minute-walk test in chronic obstructive pulmonary disease. Am J Respir Crit Care Med. 2001;163(6):1395–1399. doi:10.1164/ajrccm.163.6.2003172
- Konstantinos Katsoulis K, Kostikas K, Kontakiotis T. Techniques for assessing small airways function: possible applications in asthma and COPD. Respir Med. 2016;119:e2–e9. doi:10.1016/j.rmed.2013.05.003
- Stockley JA, Cooper BG, Stockley RA, et al. Small airways disease: time for a revisit? Int J Chron Obstruct Pulmon Dis. 2017;12:2343–2353. doi:10.2147/COPD.S138540
- Bickel S, Popler J, Lesnick B, et al. Impulse oscillometry: interpretation and practical applications. Chest. 2014;146(3):841–847. doi:10.1378/chest.13-1875
- Wei X, Shi Z, Cui Y, et al. Impulse oscillometry system as an alternative diagnostic method for chronic obstructive pulmonary disease. Medicine (Baltimore). 2017;96(46):e8543. doi: 10.1097/MD.0000000000008543
- Li L-Y, Yan T-S, Yang J, et al. Impulse oscillometry for detection of small airway dysfunction in subjects with chronic respiratory symptoms and preserved pulmonary function. Respir Res. 2021;22(1):68. doi: 10.1186/s12931-021-01662-7
- Chiu H-Y, Hsiao Y-H, Su K-C, et al. Small airway dysfunction by impulse oscillometry in symptomatic patients with preserved pulmonary function. J Allergy Clin Immunol Pract. 2020;8(1):229–235.e223. doi: 10.1016/j.jaip.2019.06.035
- Lu L, Peng J, Zhao N, et al. Discordant spirometry and impulse oscillometry assessments in the diagnosis of small airway dysfunction. Front Physiol. 2022;13:892448. doi: 10.3389/fphys.2022.892448
- Chetta A, Facciolongo N, Franco C, et al. Small airways disease, and extra-fine formulations in asthma and chronic obstructive pulmonary disease: windows for new opportunities. Ther Clin Risk Manag. 2022;18:965–979. doi: 10.2147/TCRM.S369876
- Santus P, Radovanovic D, Pecchiari M, et al. The relevance of targeting treatment to small airways in asthma and COPD. Respir Care. 2020;65(9):1392–1412. doi: 10.4187/respcare.07237
- Milic-Emili J, Torchio R, D’Angelo E. Closing volume: a reappraisal (1967–2007). Eur J Appl Physiol. 2007;99(6):567–583. doi: 10.1007/s00421-006-0389-0
- Boeck L, Gensmer A, Nyilas S, et al. Single-breath washout tests to assess small airway disease in COPD. Chest. 2016;150(5):1091–1100. doi: 10.1016/j.chest.2016.05.019
- Stanojevic S, Bowerman C, Robinson P. Multiple breath washout: measuring early manifestations of lung pathology. Breathe. 2021;17(3):210016. doi:10.1183/20734735.0016-2021
- Daynes E, Greening N, Owers-Bradley J, et al. The validity of shortened multiple-breath washout testing using sulfur hexafluoride in the assessment of patients with COPD. ERJ Open Res. 2021;7(3):00379–2020. doi: 10.1183/23120541.00379-2020
- Lynch DA. Imaging of small airways disease and chronic obstructive pulmonary disease. Clin Chest Med. 2008;29(1):165–179, vii.doi:10.1016/j.ccm.2007.11.008
- Tanabe N, Shima H, Sato S, et al. Direct evaluation of peripheral airways using ultra-high-resolution CT in chronic obstructive pulmonary disease. Eur J Radiol. 2019;120:108687. doi: 10.1016/j.ejrad.2019.108687
- Pike D, Kirby M, Guo F, et al. Ventilation heterogeneity in ex-smokers without airflow limitation. Acad Radiol. 2015;22(8):1068–1078. doi: 10.1016/j.acra.2015.04.006
- Capaldi DPI, Zha N, Guo F, et al. Pulmonary imaging biomarkers of gas trapping and emphysema in COPD: (3)He MR imaging and CT parametric response maps. Radiology. 2016;279(2):597–608. doi: 10.1148/radiol.2015151484
- Serajeddini H, Eddy RL, Licskai C, et al. FEV1 and MRI ventilation defect reversibility in asthma and COPD. Eur Respir J. 2020;55(3):1901947. doi: 10.1183/13993003.01947-2019
- MacNeil JL, Capaldi DPI, Westcott AR, et al. Pulmonary imaging phenotypes of chronic obstructive pulmonary disease using multiparametric response maps. Radiology. 2020;295(1):227–236. doi: 10.1148/radiol.2020191735
- Bajc M, Chen Y, Wang J, et al. Identifying the heterogeneity of COPD by V/P SPECT: a new tool for improving the diagnosis of parenchymal defects and grading the severity of small airways disease. Int J Chron Obstruct Pulmon Dis. 2017;12:1579–1587. doi: 10.2147/COPD.S131847
- De Backer J, Vos W, Vinchurkar S, et al. The effects of extrafine beclometasone/formoterol (BDP/F) on lung function, dyspnea, hyperinflation, and airway geometry in COPD patients: novel insight using functional respiratory imaging. J Aerosol Med Pulm Drug Deliv. 2015;28(2):88–99. doi: 10.1089/jamp.2013.1064
- Chukowry PS, Spittle DA, Turner AM. Small airways disease, biomarkers and COPD: where are we? Int J Chron Obstruct Pulmon Dis. 2021;16:351–365. doi:10.2147/COPD.S280157
- Young AL, Bragman FJS, Rangelov B, et al. Disease progression modeling in chronic obstructive pulmonary disease.Am j respir Crit Care Med. 2020;201(3):294–302.
- Chen B, Liu Z, Lu J, et al. Deep learning parametric response mapping from inspiratory chest CT scans: a new approach for small airway disease screening. Respir Res. 2023;24(1):299. doi: 10.1186/s12931-023-02611-2
- Wang JM, Bell AJ, Ram S, et al. Topologic parametric response mapping identifies tissue subtypes associated with emphysema progression. Acad Radiol. 2024;31(3):1148–1159. doi: 10.1016/j.acra.2023.08.003
- Escamilla-Gil JM, Fernandez-Nieto M, Acevedo N, et al. Understanding the cellular sources of the fractional exhaled nitric oxide (FeNO) and its role as a biomarker of type 2 inflammation in asthma. Biomed Res Int. 2022;2022:1–9. doi: 10.1155/2022/5753524
- Vincken S, Sylvia V, Daniel S, et al. The role of FeNO in stable COPD patients with eosinophilic airway inflammation. Respir Med. 2021;181:106377. doi: 10.1016/j.rmed.2021.106377
- Alcázar-Navarrete B, Ruiz Rodríguez O, Conde Baena P, et al. Persistently elevated exhaled nitric oxide fraction is associated with increased risk of exacerbation in COPD. Eur Respir J. 2018;51(1):1701457. doi: 10.1183/13993003.01457-2017
- Antus B, Barta I, Horvath I, et al. Relationship between exhaled nitric oxide and treatment response in COPD patients with exacerbations. Respirology. 2010;15(3):472–477. doi: 10.1111/j.1440-1843.2010.01711.x
- Lu Z, Huang W, Wang L, et al. Exhaled nitric oxide in patients with chronic obstructive pulmonary disease: a systematic review and meta-analysis. Int J Chron Obstruct Pulmon Dis. 2018;13:2695–2705. doi:10.2147/COPD.S165780
- Berry M, Hargadon B, Morgan A, et al. Alveolar nitric oxide in adults with asthma: evidence of distal lung inflammation in refractory asthma. Eur Respir J. 2005;25(6):986–991. doi: 10.1183/09031936.05.00132404
- Fan X, Zhao N, Yu Z, et al. Clinical utility of central and peripheral airway nitric oxide in aging patients with stable and acute exacerbated chronic obstructive pulmonary disease. Int J Gen Med. 2021;14:571–580. doi: 10.2147/IJGM.S284688
- Holz O, Waschki B, Roepcke S, et al. Potential prognostic value of biomarkers in lavage, sputum and serum in a five year clinical follow-up of smokers with and without COPD. BMC Pulm Med. 2014;14(1):30. doi: 10.1186/1471-2466-14-30
- Chen M, Xu K, He Y, et al. CC16 as an inflammatory biomarker in induced sputum reflects chronic obstructive pulmonary disease (COPD) severity. Int J Chron Obstruct Pulmon Dis. 2023;18:705–717. doi: 10.2147/COPD.S400999
- Robinson AB, Johnson KD, Bennion BG, et al. RAGE signaling by alveolar macrophages influences tobacco smoke-induced inflammation. Am J Physiol-Lung Cellular And Mol Physiol. 2012;302(11):L1192–L1199. doi:10.1152/ajplung.00099.2012
- Ostridge K, Williams N, Kim V, et al. Relationship between pulmonary matrix metalloproteinases and quantitative CT markers of small airways disease and emphysema in COPD. Thorax. 2016;71(2):126–132. doi: 10.1136/thoraxjnl-2015-207428
- Barnes PJ, Chowdhury B, Kharitonov SA, et al. Pulmonary biomarkers in chronic obstructive pulmonary disease. Am J Respir Crit Care Med. 2006;174(1):6–14. doi: 10.1164/rccm.200510-1659PP
- Pignatti P, Visca D, Cherubino F, et al. Do blood eosinophils strictly reflect airway inflammation in COPD? comparison with asthmatic patients. Respir Res. 2019;20(1):145. doi: 10.1186/s12931-019-1111-1
- David B, Bafadhel M, Koenderman L, et al. Eosinophilic inflammation in COPD: from an inflammatory marker to a treatable trait. Thorax. 2021;76(2):188–195. doi:10.1136/thoraxjnl-2020-215167
- Lacoste JY, Bousquet J, Chanez P, et al. Eosinophilic and neutrophilic inflammation in asthma, chronic bronchitis, and chronic obstructive pulmonary disease. J Allergy Clin Immunol. 1993;92(4):537–548. doi: 10.1016/0091-6749(93)90078-T
- Maetani T, Tanabe N, Sato A, et al. Association between blood eosinophil count and small airway eosinophils in smokers with and without COPD. ERJ Open Res. 2023;9(5). doi: 10.1183/23120541.00235-2023
- Abdo M, Pedersen F, Trinkmann F, et al. Association of airway eosinophilia with small airway dysfunction in patients with mild and at risk for COPD. Int J Chron Obstruct Pulmon Dis. 2022;17:1403–1408. doi: 10.2147/COPD.S366911
- Soriano JB, Polverino F, Cosio BG. What is early COPD and why is it important? Eur Respir J. 2018;52(6):1801448. doi: 10.1183/13993003.01448-2018
- Polverino F, Soriano JB. Small airways and early origins of COPD: pathobiological and epidemiological considerations. Eur Respir J. 2020;55(3):1902457. doi: 10.1183/13993003.02457-2019
- Usmani OS, Barnes PJ. Assessing and treating small airways disease in asthma and chronic obstructive pulmonary disease. Ann Med. 2012;44(2):146–156. doi:10.3109/07853890.2011.585656
- Usmani OS. Treating the small airways. Respiration. 2012;84(6):441–453. doi:10.1159/000343629
- Hillyer EV, Price DB, Chrystyn H, et al. Harmonizing the nomenclature for therapeutic aerosol particle size: a proposal. J Aerosol Med Pulm Drug Deliv. 2018;31(2):111–113. doi: 10.1089/jamp.2017.1396
- Dhillon S, Keating GM. Beclometasone dipropionate/formoterol: in an HFA-propelled pressurised metered-dose inhaler. Drugs. 2006;66(11):1475–1483; discussion 1484. doi: 10.2165/00003495-200666110-00005
- Brown SM, Koarai A, Sturton RG, et al. A role for M(2) and M(3) muscarinic receptors in the contraction of rat and human small airways. Eur J Pharmacol. 2013;702(1–3):109–115. doi: 10.1016/j.ejphar.2013.01.054
- Dahl R, Kaplan A. A systematic review of comparative studies of tiotropium Respimat® and tiotropium HandiHaler® in patients with chronic obstructive pulmonary disease: does inhaler choice matter? BMC Pulm Med. 2016;16(1):135. doi:10.1186/s12890-016-0291-4
- Dahl R, Engel M, Dusser D, et al. Safety and tolerability of once-daily tiotropium Respimat(®) as add-on to at least inhaled corticosteroids in adult patients with symptomatic asthma: a pooled safety analysis. Respir Med. 2016;118:102–111. doi: 10.1016/j.rmed.2016.07.001
- Blair HA. Tiotropium/Olodaterol: a review in COPD. Drugs. 2019;79(9):997–1008. doi: 10.1007/s40265-019-01133-w
- Pirina P, Foschino Barbaro MP, Paleari D, et al. Small airway inflammation and extrafine inhaled corticosteroids plus long-acting beta2-agonists formulations in chronic obstructive pulmonary disease. Respir Med. 2018;143:74–81. doi:10.1016/j.rmed.2018.08.013
- Fabbri LM, Nicolini G, Olivieri D, et al. Inhaled beclometasone dipropionate/formoterol extra-fine fixed combination in the treatment of asthma: evidence and future perspectives. Expert Opin Pharmacother. 2008;9(3):479–490. doi:10.1517/14656566.9.3.479
- Papi A, Scichilone N, Benfante A, et al. Impact of extrafine formulations of inhaled corticosteroids/long-acting beta-2 agonist combinations on patient-related outcomes in asthma and COPD. Patient Relat Outcome Meas. 2014;5:153–162. doi: 10.2147/PROM.S55276
- Wedzicha JA, Singh D, Vestbo J, et al. Extrafine beclomethasone/formoterol in severe COPD patients with history of exacerbations. Respir Med. 2014;108(8):1153–1162. doi: 10.1016/j.rmed.2014.05.013
- Nicolini G, Crisafulli T, Nicolini C, et al. Effects of beclomethasone/formoterol fixed combination on lung hyperinflation and dyspnea in COPD patients. Int J Chron Obstruct Pulmon Dis. 2011;6:503–509. doi: 10.2147/COPD.S23746
- Sturton RG, Trifilieff A, Nicholson AG, et al. Pharmacological characterization of indacaterol, a novel Once daily inhaled β 2 adrenoceptor agonist, on small airways in human and rat precision-cut lung slices. J Pharmacol Exp Ther. 2008;324(1):270–275. doi:10.1124/jpet.107.129296
- Singh D, Papi A, Corradi M, et al. Single inhaler triple therapy versus inhaled corticosteroid plus long-acting β2-agonist therapy for chronic obstructive pulmonary disease (TRILOGY): a double-blind, parallel group, randomised controlled trial. The Lancet. 2016;388(10048):963–973. doi: 10.1016/S0140-6736(16)31354-X
- Vestbo J, Papi A, Corradi M, et al. Single inhaler extrafine triple therapy versus long-acting muscarinic antagonist therapy for chronic obstructive pulmonary disease (TRINITY): a double-blind, parallel group, randomised controlled trial. The Lancet. 2017;389(10082):1919–1929. doi: 10.1016/S0140-6736(17)30188-5
- Papi A, Vestbo J, Fabbri L, et al. Extrafine inhaled triple therapy versus dual bronchodilator therapy in chronic obstructive pulmonary disease (TRIBUTE): a double-blind, parallel group, randomised controlled trial. The Lancet. 2018;391(10125):1076–1084. doi: 10.1016/S0140-6736(18)30206-X
- Price DS, Postma D, Roche N, et al. Comparing the effectiveness of small-particle versus large-particle inhaled corticosteroid in COPD. Int J Chron Obstruct Pulmon Dis. 2014;9:1163–1186. doi: 10.2147/COPD.S68289
- Sonnappa S, Martin R, Israel E, et al. Risk of pneumonia in obstructive lung disease: a real-life study comparing extra-fine and fine-particle inhaled corticosteroids. PLOS ONE. 2017;12(6):e0178112. doi: 10.1371/journal.pone.0178112
- Usmani OS, Scichilone N, Mignot B, et al. Airway deposition of extrafine inhaled triple therapy in patients with COPD: a model approach based on functional respiratory imaging computer simulations. Int J Chron Obstruct Pulmon Dis. 2020;15:2433–2440. doi: 10.2147/COPD.S269001
- Ferguson GT, Rodriguez-Roisin R, Reisner C, et al. Pharmacokinetics of glycopyrronium/formoterol fumarate dihydrate delivered via metered dose inhaler using co-suspension delivery technology in patients with moderate-to-very severe COPD. Int J Chron Obstruct Pulmon Dis. 2018;13:945–953. doi:10.2147/COPD.S154988
- Doty A, Schroeder J, Vang K, et al. Correction to: drug delivery from an innovative LAMA/LABA Co-suspension delivery technology fixed-dose combination MDI: evidence of consistency, robustness, and reliability. AAPS PharmScitech. 2018;19(6):2753. doi: 10.1208/s12249-017-0916-9
- Grillet P-E, Le Souder C, Rohou J, et al. Glycopyrrolate and formoterol fumarate for the treatment of COPD. Expert Rev Respir Med. 2021;15(1):13–25. doi:10.1080/17476348.2020.1807946
- Usmani O, Roche N, Wahab E, et al. A scintigraphy study of budesonide/glycopyrrolate/formoterol fumarate metered dose inhaler in patients with moderate-to-very severe chronic obstructive pulmonary disease. Respir Res. 2021;22(1):261. doi: 10.1186/s12931-021-01813-w
- Poole P, Chong J, Cates CJ. Mucolytic agents versus placebo for chronic bronchitis or chronic obstructive pulmonary disease. Cochrane Lib. 2015. doi: 10.1002/14651858.CD001287.pub5
- Tse HN, Raiteri L, Wong KY, et al. High-dose N-acetylcysteine in stable COPD: the 1-year, double-blind, randomized, placebo-controlled HIACE study. Chest. 2013;144(1):106–118. doi: 10.1378/chest.12-2357
- Bhatt SP, Rabe KF, Hanania NA, et al. Dupilumab for COPD with type 2 inflammation indicated by eosinophil counts. N Engl J Med. 2023;389(3):205–214. doi: 10.1056/NEJMoa2303951
- Castro M, Rabe KF, Corren J, et al. Dupilumab improves lung function in patients with uncontrolled, moderate-to-severe asthma. ERJ Open Res. 2020;6(1):00204–2019. doi: 10.1183/23120541.00204-2019
- Pelaia C, Lombardo N, Busceti MT, et al. Short-term evaluation of dupilumab effects in patients with severe asthma and nasal polyposis. J Asthma Allergy. 2021;14:1165–1172. doi: 10.2147/JAA.S328988
- Bhatt SP, Soler X, Wang X, et al. Association between functional small airway disease and FEV1 decline in chronic obstructive pulmonary disease. Am J Respir Crit Care Med. 2016;194(2):178–184. doi: 10.1164/rccm.201511-2219OC
- Pompe E, Moore CM, Mohamed Hoesein FAA, et al. Progression of emphysema and small airways disease in cigarette smokers. Chronic Obstr Pulm Dis. 2021;8(2):198–212. doi: 10.15326/jcopdf.2020.0140
- Zhao N, Wu F, Peng J, et al. Preserved ratio impaired spirometry is associated with small airway dysfunction and reduced total lung capacity. Respir Res. 2022;23(1):298. doi: 10.1186/s12931-022-02216-1
- Sharpe AL, Reibman J, Oppenheimer BW, et al. Role of small airway dysfunction in unexplained exertional dyspnoea. ERJ Open Res. 2023;9(3):00603–2022. doi: 10.1183/23120541.00603-2022