ABSTRACT
Introduction
In the past two decades, bronchoscopy for peripheral pulmonary lesions (PPLs) has improved its diagnostic yield due to the combination of various instruments and devices. Meanwhile, the application is complex and intertwined.
Areas covered
This review article outlines strategies in diagnostic bronchoscopy for PPLs. We summarize the utility and evidence of key instruments and devices based on the results of clinical trials. Future perspectives of bronchoscopy for PPLs are also discussed.
Expert opinion
The accuracy of reaching PPLs by bronchoscopy has improved significantly with the introduction of combined instruments such as navigation, radial endobronchial ultrasound, digital tomosynthesis, and cone-beam computed tomography. It has been accelerated with the advent of approach tools such as newer ultrathin bronchoscopes and robotic-assisted bronchoscopy. In addition, needle aspiration and cryobiopsy provide further diagnostic opportunities beyond forceps biopsy. Rapid on-site evaluation may also play an important role in decision during the procedures. As a result, the diagnostic yield of bronchoscopy for PPLs has improved to a level comparable to that of transthoracic needle biopsy. The technique and technologies developed in the diagnosis will be carried over to the next step in the transbronchial treatment of PPLs in the future.
Disclaimer
As a service to authors and researchers we are providing this version of an accepted manuscript (AM). Copyediting, typesetting, and review of the resulting proofs will be undertaken on this manuscript before final publication of the Version of Record (VoR). During production and pre-press, errors may be discovered which could affect the content, and all legal disclaimers that apply to the journal relate to these versions also.1. Introduction
After the first flexible bronchofiberscope was reported by Ikeda in 1966, the range of the airways that had been visualized only centrally with the previous rigid bronchoscopes was expanded to the periphery [Citation1]. Since then, bronchoscopy has been progressively developed and used for various purposes.
One of the current uses of bronchoscopy is the diagnosis of peripheral pulmonary lesions (PPLs). The PPLs are defined as localized lesions in peripheral lung areas that cannot be observed directly by bronchoscopy. Although its sampling has conventionally been performed while confirming the location of the target lesion under X-ray fluoroscopy, it was difficult to accurately determine its three-dimensional location, resulting in a low diagnostic yield [Citation2,Citation3]. Nevertheless, it is a relatively safe technique in that it utilizes anatomical structures and has been contrasted with transthoracic needle biopsy (TTNB), which has a higher risk of complications but also a higher diagnostic accuracy.
However, bronchoscopy has made remarkable progress in the past two decades, and the diagnostic yield has improved to a level approaching TTNB, due to the combination of various instruments and devices. At the same time, they are complex and intertwined, and this review article outlines each of them.
2. Navigation
Effective navigation plays a crucial role in bronchoscopic biopsy procedures for PPLs. However, this task is often challenging owing to the intricate branching of the human airway. Missing even a single airway generation can cascade into downstream failures, ultimately leading to unsuccessful localization of the target lesion. Thus, the crux of successful navigation lies in precisely segmenting peripheral airways to delineate a clear pathway from the trachea to the target. This segmentation process can be accomplished either manually or through automated methods utilizing virtual bronchoscopic navigation (VBN) platforms.
2.1. Manual planification
Manual segmentation of complex airway anatomy through meticulous analysis of stacks of computed tomography (CT) slices is feasible but remains a laborious and intricate process [Citation4]. Additionally, the segmented airway images often lack visual correlation with the actual bronchoscopy procedures. A more pragmatic approach to manual segmentation of airway for bronchoscopy procedures is through the bronchial branch technique, pioneered by Kurimoto. This technique concentrates solely on the airway of interest, meticulously analyzing every airway bifurcation and orientation leading to the target lesion, thereby constructing a bronchoscopic roadmap instead of laboriously segmenting the entire airway tree () [Citation5]. Employing this method in bronchoscopy with radial endobronchial ultrasound (R-EBUS) for PPLs, a study by Kho et al., reported a navigation success yield of 98.9% in 98 targets [Citation6]. The pathological diagnostic yield for this cohort was 88.8%, with targets within the first five generations associated with better yield. Similarly, a retrospective study of 48 patients with PPLs found that this method achieved a non-inferior pathological diagnostic yield compared to the VBN group (75.0% vs. 61.9%, p=0.25) [Citation7]. Additionally, the planning time (1.32 vs. 9.79 min, p<0.001) and operating cost were significantly lower in the manual navigation group.
Figure 1. A lung nodule measuring 1.9 cm was located in the apical segment of the right upper lobe. The CT scan in axial reconstruction was rotated 90 degrees counter clockwise to simulate the actual bronchoscopic view (upper panel). The airway and each bifurcation leading to the target were traced and marked from the right upper lobe bronchus into the target segment of RB1biiα (middle panel) employing the manual bronchial branch tracing technique. The actual bronchoscopic view, accessed with an ultrathin bronchoscope (BF-MP190F), was shown in the lower panel with a prophylactic balloon blocker in place (lower panel). The final approach was adjusted with single-plane fluoroscopy, confirming a concentric R-EBUS lesion. Cryobiopsy confirmed lung adenocarcinoma. (Unpublished data obtained from study approved by the Medical Research & Ethics Committee, Ministry of Health Malaysia (NMRR ID-23-03628-QAG) which waived the need for individual consent for this publication.)
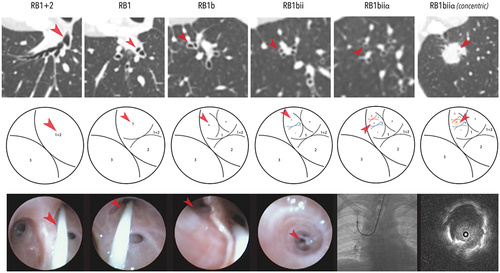
Likewise, in comparison to VBN planning methods, a study by Miyake et al. demonstrated that manual methods were able to identify a complete route to the target lesion more readily in 94% of cases, compared to only 49%–62% with two commercially available VBN platforms [Citation8]. In addition to the bronchial branch tracing method, another manual approach involves utilizing multiplanar CT scan reconstruction to estimate a procedure pathway to the target lesion was previously reported. In a large North American cohort of 348 patients, this approach reported a navigation yield of 95.4%, but with a histological diagnostic yield of only 58.9% [Citation9]. However, while manual planification remains feasible, especially for larger PPLs with bronchus sign, mastering it may involve a steep learning curve, as spatial orientation is crucial, and performance may vary depending on the individual.
2.2. Virtual bronchoscopic navigation
VBN is an imaging modality that reconstructs CT scans into three-dimensional bronchoscopic airway images, enabling bronchoscopists to follow and navigate to the target of interest during procedures. Reports on VBN-guided bronchoscopy date back almost two decades, with a series of 38 target lesions approached successfully in 94.7%, translating to a diagnostic yield of 81.6% using an ultrathin bronchoscope [Citation10]. The V-NINJA study, a prospective multicenter trial in 199 patients, then demonstrated a higher diagnostic yield in the VBN-assisted group than the non-assisted group (80.4% vs. 67.0%, p=0.032) when using a thin bronchoscope with R-EBUS and a guide sheath (GS) [Citation11]. However, a subsequent study with an ultrathin bronchoscope without R-EBUS in 334 patients showed no difference in the diagnostic yields between the VBN and non-VBN groups (67.1% vs. 59.9%, p=0.173) [Citation12]. Since then, various meta-analyses have been reported with conflicting results. One meta-analysis, comprising ten randomized controlled trials (RCTs) and case-control studies, reported a higher diagnostic yield of VBN compared to non-VBN (odds ratio [OR], 1.69; 95% confidence interval [CI], 1.32–2.18). This benefit was particularly notable for bronchus sign positive malignant PPLs measuring 2 cm or less, located in the peripheral third or upper lobes of the lung [Citation13]. Contrastingly, another meta-analysis, which included six RCTs, found an overall similar diagnostic yield with and without VBN (74.17% vs. 69.51%) [Citation14]. However, consistently better results were observed in the VBN group for smaller PPLs measuring 2 cm or less in these two meta-analyses [Citation13,Citation14].
The problem with automated VBN systems lies in their inability to segment the entire airway of interest in totality from trachea to the target lesion due to the frequent poor CT resolution in the peripheral region of the lung. A study measuring the distance from the terminal end of the navigation pathway generated by VBN to target PPL revealed that in electromagnetic navigation bronchoscopy (ENB)-based VBN system, the distance ranged from 14.2 to 17.2 mm, while in the latest robotic-assisted bronchoscopy (RAB)-based VBN system, it was approximately 9.4 mm [Citation15]. This means that during the final approach, an accurate path may still be lacking and may affect the overall accuracy of navigation, especially for smaller PPLs.
2.3. Electromagnetic navigational bronchoscopy
Although both manual methods and VBN can generate a roadmap, both require the bronchoscopist to follow and match it manually during the procedure. ENB, akin to VBN, pre-plans a roadmap before the procedure. However, it has additional function: through electromagnetic sensing, it tracks the bronchoscope and tools during the procedure, overlaying this information onto the VBN map. Current commercially available systems include catheters that allow the placement of additional tools like an R-EBUS probe and sampling devices after localization with the electromagnetic sensor.
The first feasibility study of ENB in an animal model was reported almost two decades ago, demonstrating a registration accuracy of 4.5 mm on average [Citation16]. Subsequently, the same group conducted the first in human trial in year 2006 involving 13 patients, achieving an average navigation accuracy of 5.7 mm and a diagnostic yield of 69% [Citation17]. Since then, numerous reports on ENB have been published in the literature, with varying diagnostic yields. The largest prospective trial, the NAVIGATE study, involved 1,215 subjects, reported a pathological diagnostic yield of 73% and a pneumothorax and bleeding risk of 2.9% and 1.5%, respectively [Citation18]. Notably, several meta-analyses have since then been conducted, showing pooled diagnostic yields ranging from 70.0% (95% CI, 67.1%–72.9%) in year 2012 to 77% (95% CI, 72%–82%) in a recent analysis in year 2020 [Citation19–21].
Despite a gradual increase in the diagnostic yield over the years, it has plateaued at less than 80%, with several issues surrounding the use of ENB. First, there is the limited steerability of tools with large caliber, restricting access to the peripheral regions of the lung. Although some tools offer real-time tip tracking or come with different curvature, maneuverability remains limited. Additionally, during biopsy, vision relies solely on the virtually created target, which can be affected by various factors, notably CT-to-body divergence. A study showed a significant drop in the diagnostic yield (77.2% vs. 44.4%) when the divergence was more than 4 mm [Citation22]. The more recent upgrade of the ENB system, the ILLUMISITETM (Medtronic, Minneapolis, MN, USA), which incorporates real-time imaging to correct CT-to-body divergence, reported a diagnostic yield of 87.2%, with the average corrected distance after tomosynthesis being 15.4 mm (ranging from 0.4 to 29.8 mm) [Citation23]. Other reports on the incorporation of real-time imaging into ENB has reported a good diagnostic yield ranging from 79% to 84 % for target nodule sizes ranging from 15 to 22 mm [Citation24–26].
3. Confirmation and verification
While the navigation process is crucial in guided bronchoscopy procedures, the verification of navigation is equally essential as it provides real-time feedback on its accuracy. Various imaging-based methods have proven to be helpful in guided bronchoscopy, each offering different levels of accuracy.
3.1. Single-plane fluoroscopy
One of the most commonly utilized imaging modalities for target verification is two-dimensional fluoroscopy, which is widely available worldwide. While useful in certain cases, its main limitation lies in its single-plane visualization. It often fails to confidently ascertain the three-dimensional relationship between the tool and target. Additionally, visualization is poor or almost impossible for small lesions, as well as part-solid or pure ground-glass nodules (GGNs). Furthermore, it struggles when the target lesion is situated in overlapping areas such as the retrocardiac, para-spinal, and diaphragmatic regions. In the early days, transbronchial biopsy (TBB) performed solely under fluoroscopy reported an overall diagnostic yield of 62.4%, with even poorer yield of 35.3% for lesions smaller than 2 cm [Citation27]. Currently, fluoroscopy is frequently used alongside with other advanced verification techniques to maximize the diagnostic yield of PPLs. A recent innovation involves the usage of digital reconstructed radiographs to reconstruct a fluoroscopy image from CT images, with a pre-planned path overlayed onto the virtually constructed fluoroscopy image, providing guidance during the procedure. Studies have demonstrated that this approach, known as virtual fluoroscopy (VF), increases the diagnostic yield of fluoroscopically invisible targets from 51.2% to 77.1% (/c) [Citation28]. Furthermore, VF has been shown to correct R-EBUS orientation to within the lesion in 55.6% of 18 patients with initial sub-optimal R-EBUS orientation [Citation29].
Figure 2. High-resolution computed tomography (a) showing a 9.9-mm part-solid nodule in the right S3 (arrow head). Virtual fluoroscopy, overlaying the target lesion (arrow) and the pre-planned bronchial path toward it, was reconstructed from HRCT: anteroposterior (b) and right anterior 45-degree oblique views (c). The lesion was invisible on actual fluoroscopy: anteroposterior (d) and right anterior 45-degree oblique views (e), but was approached while being matched with the path on the VF. A radial endobronchial ultrasound image of a blizzard sign adjacent to the lesion (upper right corner of d, arc indicating expanded range of the snowstorm appearance) was detected. Cryobiopsy (f) at the location revealed lung adenocarcinoma, which was later proved to be minimally invasive by the partial resection This is one of the cases included in reference 69. (Unpublished data obtained from studies approved by the National Cancer Center Institutional Review Board (No. 2018-090). Comprehensive research consent was obtained from the patient and individual consent for this publication was waived.)
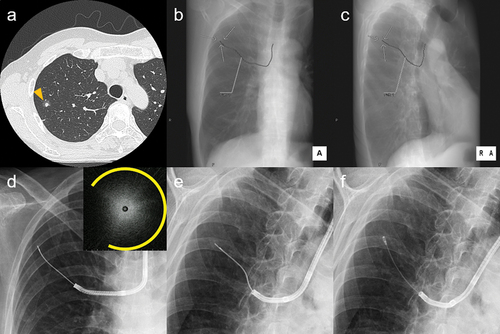
3.2. Radial endobronchial ultrasound
R-EBUS is a probe-based tool that can be inserted into the working channel (WC) of a bronchoscope to offer real-time feedback on the location of the target lesion. The ultrasound image not only confirms navigation but also reveals the relationship of the lesion to the navigated airway, its nature, and, in certain cases, the surrounding vasculature.
Employing R-EBUS for PPL identification was first described in the early 1990s [Citation30,Citation31]. Subsequently, in the early 2000s, R-EBUS-guided TBB for PPLs was proven to be feasible by Herth et al. [Citation32]. In the same period, Kurimoto et al. also described a classification of R-EBUS internal structures of PPLs, where a homogeneous pattern predicts benign pathology, while hyperechoic dots and linear arcs with a heterogeneous pattern predict malignancy [Citation33]. Since then, numerous reports on the performance of R-EBUS in PPLs have been published, leading to various meta-analyses being performed. However, the diagnostic yield has remained fairly plateaued, ranging from 69% to 73% over the last decade [Citation19,Citation34–37].
The main drawbacks of R-EBUS include the necessity of contact between the airway wall and the target lesion for signal detection. Additionally, while R-EBUS offers real-time imaging, the actual biopsy procedure is not guided in real-time. This poses a risk of tool dislodgement occurring between the retrieval of an R-EBUS probe and the actual biopsy procedure. To address this issue, a previous innovation involving a “double-barrel” bronchoscope has shown promise in providing real-time biopsy feedback in two patients by attaching an external catheter to a diagnostic bronchoscope [Citation38]. More recently, the iNodTM (Boston Scientific, Watertown, MA, USA) has been introduced, featuring a rotatable ramped needle coupled with an R-EBUS probe, enabling real-time visualization feedback to the physician during the biopsy procedure. The first in human trial with the prototyped model demonstrated clinical feasibility, with all 23 lesions successfully visualized and sampled under real-time visualization, yielding specimens adequate for cytology evaluation [Citation39]. Lastly, obtaining an R-EBUS signal can be challenging in subsolid nodules, particularly in pure GGNs. The “blizzard” and “mix-blizzard” signs of R-EBUS have been described in pure GGNs and part-solid nodules () [Citation40], but interpretation remains challenging for the wider pulmonology community.
3.3. Digital tomosynthesis
Digital tomosynthesis (DT) is increasingly being adopted as a means of verifying navigation in guided bronchoscopy. It utilizes computer-based reconstruction algorithms to produce radiographic images with depth of field from multiple single-plane X-ray images. These images are captured over a limited range of angles, with an X-ray tube and detector circling around the object [Citation41]. Unlike conventional CT scans, which acquire images over 180 to 360 degrees, digital tomosynthesis utilizes X-ray images acquired over angles as small as 50 degrees [Citation42].
The ILLUMISITETM and LungVisionTM (Body Vision Medical, Campbell, CA, USA) are two of the most widely used commercially available DT systems. After navigation completion on both platforms, digital tomosynthesis images are created by rotating the fluoroscopy C-arm. This enables the assessment of the relationship between the tools and the target lesion. Additionally, in both systems, the actual target location is adjusted and augmented into the single-plane fluoroscopy to correct the real-time lesion position.
The ILLUMISITETM represents the latest upgrade of the ENB system, incorporating real-time DT to correct CT-to-body divergence. One study from North America reported a diagnostic yield of 87.2% with the aid of DT after correcting for CT-to-body divergence, which averaged around 15.4mm [Citation23]. A retrospective study comparing ENB with and without real-time DT demonstrated a significant increase in the diagnostic yield for targets measuring around 15mm, from 54% to 79% [Citation24]. Two other prospective single-arm studies using the ILLUMISTETM reported high diagnostic yields of 83%–84% [Citation25,Citation26]. More recently, a retrospective study compared RAB (143 PPLs) to the ILLUMISITETM (197 PPLs), showing a comparable diagnostic yield of 77% vs. 80%, with similar complication rates [Citation43]. Therefore, further exploration of the utility of DT with ENB is warranted.
For the LungVisionTM, studies have highlighted its efficacy in navigating bronchoscopy procedures. The first-generation system utilized a steering catheter and was employed in 55 patients [Citation44]. This approach achieved a navigation success rate of 93%, as verified by R-EBUS, with an overall diagnostic yield of 92.3%. Additionally, a single-center study showcased a navigation success rate of 96.1%, confirmed by cone-beam CT (CBCT), across 51 patients [Citation45].
3.4. Cone-beam computed tomography
Due to the inherent limitations of DT, particularly in achieving high image resolution and clarity of soft tissue structures such as blood vessels, interest in CBCT has surged within the bronchoscopy community. CBCT offers the advantage of providing high-quality, three-dimensional imaging during bronchoscopy procedures. This capability enables real-time visualization of the precise location of the PPL and its relationship to the tools.
The integration of CBCT alongside other navigational tools has shown promise in achieving favorable diagnostic outcomes. In various studies, CBCT has been coupled with different bronchoscopic techniques to enhance diagnostic accuracy. For instance, a study combining ENB and RAB with cryoprobe under CBCT guidance reported a high diagnostic yield of 90% for PPLs [Citation46]. Similarly, the utilization of thin and ultrathin bronchoscopes in tandem with R-EBUS for PPLs in 20 patients yielded a diagnostic yield of 70% with CBCT verification [Citation47]. Moreover, a large cohort study from the Netherlands reported a substantial diagnostic yield of 76.4% for PPLs using ENB and R-EBUS with CBCT [Citation48]. These findings underscore the efficacy of CBCT in combination with other navigational tools, highlighting its potential to improve diagnostic accuracy in bronchoscopy procedures.
However, the integration of CBCT is not without its limitations. These include concerns about radiation dose exposure, limited availability in some medical facilities, as well as the potential for a prolonged procedure duration. Despite these drawbacks, CBCT remains a valuable tool in enhancing diagnostic accuracy during bronchoscopy procedures moving forward.
3.5. Direct visualization
As of now, most verification techniques are imaging-dependent; therefore, bronchoscopists still need to rely heavily on the quality of imaging as well as address the issue of CT-to-body divergence to verify the navigation accurately. The extreme miniaturization of tools now offers bronchoscopists an exciting opportunity for direct visualization when approaching the furthest reaches of the lung. For example, a commercially available 1.8-mm video-endoscopic probe (Iriscope; Lys Medical, Charleroi, Belgium) has been reported to visualize the extreme periphery of the lung during ENB [Citation49]. Additionally, a prototype 0.97-mm ultra-thin optical fiberscope has demonstrated its ability to access the peripheral lung fields in three resected human lungs [Citation50]. Although these vision probes may provide direct visualization feedback, they face challenges due to the lack of a WC and limited steerability. Moreover, for targets without airway invasion, direct vision remains impossible. Looking ahead, future developments may offer promise with the creation of an outside-the-scope system that is fully detachable and can be attached to a bronchoscope [Citation51]. This innovation could prove valuable in allowing access to the very peripheral regions of the lung under direct visualization when coupled with such vision probes.
4. Sampling
As it is a transbronchial approach, the optimal sampling method depends on the location relationship between the lesion and the bronchi. This relationship originated with the Tsuboi classification based on morphological examination of resected lung carcinomas [Citation52], and was later named as a bronchus sign by Gaeta et al. [Citation53]. The bronchus sign is a finding that shows a bronchus leading directly to a lesion on CT, and forceps biopsy or brushing is usually sufficient for its positive cases, whereas needle aspiration may be more effective for its negative cases. The bronchus sign was reported to be a significant factor that influences the R-EBUS visualization and, through it, the diagnostic yield as well [Citation54]. It should be noted, however, that several classifications of bronchus signs have been proposed and are not universal [Citation55]. This section focuses on current commonly practiced tissue sampling methods ().
Figure 3. Differences in tissue specimens from each sampling method in the same patient with lung adenocarcinoma. following needle aspiration (a) to penetrate the bronchial wall, forceps biopsy (b) and cryobiopsy (c) were performed on the mass in the right upper lobe. Each histopathological image is shown in that order so that the magnification is the same between specimens (low-magnification images with magnified images of each square area). The aspiration needle specimen (d) is a so-called cell block, and the apparent volume usually seems large because fragmented cell clusters are mixed with other aspirated material. In contrast, the forceps biopsy and cryobiopsy specimens (e, f) are true tissues, with the latter clearly larger and less crushed than the former. (Unpublished data obtained from studies approved by the National Cancer Center Institutional Review Board (No. 2018-090). Comprehensive research consent was obtained from the patient and individual consent for this publication was waived.)
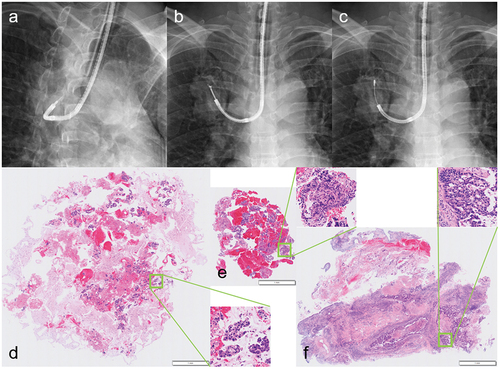
4.1. Forceps biopsy
The most frequently used sampling method has been forceps biopsy, often referred to as TBB, which is the benchmark for the diagnostic accuracy. As the cumulative diagnostic yield by number of sequential biopsies in EBUS-GS was reported to reach a plateau at five or more [Citation56], sampling of that number is often the goal. The American College of Chest Physicians (ACCP) guidelines reported a significant difference in the diagnostic yields, 63% for lesions >2 cm and 34% for lesions <2 cm, respectively [Citation2]. In addition, the yields for peripheral lung cancer using R-EBUS and ENB were 73% and 71%, respectively, and the combinations have been recommended to improve the diagnostic performance.
In fact, in a systematic review and meta-analysis of combined R-EBUS, all studies with known sampling methods performed TBB [Citation36]. In this study, the overall weighted diagnostic yield was 70.6% (95% CI, 68%–73.1%), with yields significantly higher for lesions >2 cm, malignant in nature, bronchus sign positive, and also R-EBUS within as opposed to adjacent to. On the other hand, in a systematic review and meta-analysis of ENB, the pooled diagnostic yield was 64.9% (95% CI, 64.9%–70.3%), with yields higher for larger lesions, bronchus sign positive, combined use of R-EBUS, and so on [Citation20]. In another later systematic review and meta-analysis focused on lung cancer diagnosis, the pooled sensitivity for malignancy was 77% (95% CI, 72%–82%), with the mean distance of the sensor tip to the center of the lesion, the number of tissue sampling methods, and the cancer prevalence as factors associated with that [Citation21].
There was also a systematic review and meta-analysis that examined the association between bronchus signs and the diagnostic yield of guided bronchoscopy including R-EBUS and ENB [Citation57]. The overall weighted yield of the bronchus sign positive cases was 74.1% (95% CI, 68.3%–79.5%), whereas that of negative cases was 49.6% (95% CI, 39.6%–59.5%), with the OR of 3.4 (95% CI, 2.4–5.0).
4.2. Needle aspiration
The utility and safety of transbronchial needle aspiration (TBNA) for PPLs was first reported in 1984 [Citation58], and in a systematic review and meta-analysis of TBNA performed under conventional X-ray fluoroscopy, its overall diagnostic yield was 53% (95% CI, 44%–61%), which was higher than that of TBB when directly compared (60% [95% CI, 49%–71%] vs. 45% [95% CI, 37%–54%]) [Citation59]. In addition, the subgroup analyses revealed higher TBNA yields for cases with bronchus sign positive, with rapid-onsite evaluation (ROSE) performed, of malignant lesions, with lesions >3 cm.
Since the introduction of R-EBUS, it has often been used in conjunction with TBNA. An RCT reported a significantly higher diagnostic yield in the group with TBNA than without (78.4% vs. 60.6%, p=0.015) [Citation60]. In this study, TBNA showed the highest yield (62.5%) among all sampling methods performed, and the yield remained unchanged even when R-EBUS showed adjacent to the lesions (p=0.89). On the other hand, a multivariable analysis of factors that affected the diagnostic yield was conducted in an analysis using the AQuIRE registry, with a primary focus on the yields when R-EBUS and ENB were used or not used [Citation61]. The results showed that TBNA was one of the factors that increased the yield, with only 16.4% of cases in which TBNA was performed, but 9.5% of cases in which TBB was also performed could only be diagnosed by TBNA.
Conventional needles have several limitations due to their nature, and new needles are being introduced. First, a new 21-gauge needle, the PeriView FLEX (Olympus, Tokyo, Japan) was introduced. The needle tip is laser cut to ensure high flexibility, whereas needles are generally rigid and straight. It also has a thinner OD, making it applicable to a small GS and an ultrathin bronchoscope [Citation62]. Second, a new 22-gauge needle, the Compass Steerable Needle (Serpex, Santa Clara, CA, USA) was introduced. The needle tip allows articulation to 70° with 360 degrees of rotation to direct it toward the target lesion [Citation63]. Third, a new 25-gauge needle, the iNodTM which combines R-EBUS transducer with needle indicator was introduced (for its feasibility, see section 3.2).
4.3. Cryobiopsy
Cryoprobes were originally used as one of the debulking tools, causing tissue necrosis through freeze-thaw cycles (i.e., cryotherapy) [Citation64]. Thereafter, frozen endobronchial tumors could be removed along with the probe tip because of their enhanced freezing and tensile strength (i.e., cryoextraction), and cryoprobes have also been applied as a sampling method (i.e., cryobiopsy). In fact, tissue samples obtained in cryoextraction was reported by Hetzel et al. to have extraordinarily good quality in terms of size and artifact-free sample area [Citation65]. A subsequent RCT for endobronchial biopsy revealed that cryobiopsy significantly improved the diagnostic yield compared to conventional forceps biopsy (95.0% vs. 85.1%, p<0.001), without increasing the risk of significant bleeding [Citation66].
The cryobiopsy has also been applied as a lung biopsy, and its efficacy is now well established for interstitial lung diseases (ILDs) [Citation67]. Meanwhile, although they are the same lung biopsy, ILDs do not require targeting due to diffuse shadows, whereas PPLs require it. A systematic review and meta-analysis summarizing early publications of R-EBUS-guided cryobiopsy for PPLs reported similar pooled diagnostic yields compared to TBB (77% [95% CI, 71%–84%] vs. 72% [95% CI, 60%–83%]) [Citation68]. With the exception of one RCT that used a 1.2-mm prototype probe [Citation69], all studies used conventional reusable probes, and their thicker and stiffer properties made them more difficult to guide toward PPLs than forceps, which may be the reason for the lack of difference in the diagnostic yields. Matsumoto et al. devised a technique to overcome this limitation, and reported that additional cryobiopsy to conventional sampling methods improved diagnostic yields from 81.3% to 89.9% [Citation70]. This advantage was the most apparent in lesions where R-EBUS showed adjacent to (from 69.4% to 84.3%), reflecting the characteristics of the entirely circumferential biopsy. Furthermore, the later comparative analysis after balancing baseline characteristics by propensity scores showed a significantly higher diagnostic yield with cryobiopsy than without (89.2% vs. 77.6%; OR, 2.36 [95% CI, 1.65–3.38]; p<0.001) [Citation71]. The subgroup analysis showed that cryobiopsy was effective for most factors, but had limited effect on upper lobe lesions.
Recently, single-use probes that are thinner than reusable probes have become commercially available and offer much better inducibility to PPLs. The results of feasibility studies with multiple settings for PPLs of 30 mm or less have been reported. The diagnostic yield of the 1.7-mm probe alone in combination with a thin bronchoscope was 94% (83.5%–98.8%) [Citation72], whereas that of the 1.1-mm probe alone, mainly in combination with a thin bronchoscope and a small GS, was 90% (95% CI, 78%–97%) [Citation73]. In contrast, Oki et al. performed TBB followed by cryobiopsy using the 1.1-mm probe in combination with an ultrathin bronchoscope, and the yields of TBB, cryobiopsy, and the combination were reported to be 54%, 62%, and 74%, respectively [Citation74]. Furthermore, Nakai et al. reported high safety in addition to the efficacy of a unique cryobiopsy method using the 1.1-mm probe via its WC while wedging a thin bronchoscope [Citation75]. On the other hand, the 1.1-mm cryoprobe is also compatible with ENB and RAB, and the presence of cases that could be diagnosed by cryobiopsy alone was reported in each single-arm cohort study, suggesting that it may boost diagnostic yields of PPLs [Citation76,Citation77].
5. Approach tool
The previous chapters have described navigation, confirmation/verification, and sampling, respectively. With a thorough understanding of these factors, a comprehensive decision on how to diagnose the target lesion should be made based on the instruments at each facility, the characteristics of the lesion, and the purpose of the procedure. It is then necessary to choose what pathway to finally approach the lesion.
5.1. Flexible bronchoscope
There is a wide variety of flexible bronchoscopes with different outer diameters (ODs) and WCs. The thinner the OD, the more peripherally the bronchoscope can be wedged into, but at the same time, the WC becomes smaller, limiting the devices that can be inserted. Sampling devices are often based on a 2.0-mm WC, and many such types exist in the bronchoscope lineup, but if the WC is the same, a thinner OD bronchoscope should be selected for PPLs [Citation78]. In other words, there is little advantage in using a general-purpose type, and a thin bronchoscope would be deemed the standard. Thus, flexible bronchoscopes used for PPLs can be broadly classified into the following four types: in addition to the thin, ultrathin (conventional and newer) and therapeutic ().
Figure 4. Four main types of flexible bronchoscopes for peripheral pulmonary lesions. From left to right: 5.8-mm therapeutic bronchoscope, BF-1TH1200 with a 3.0-mm working channel (WC); 4.2-mm thin bronchoscope, BF-P290 with a 2.0-mm WC; 3.0-mm newer ultrathin bronchoscope, BF-MP290F with a 1.7-mm WC; and 2.8-mm conventional ultrathin bronchoscope, BF-XP260F with a 1.2-mm WC.
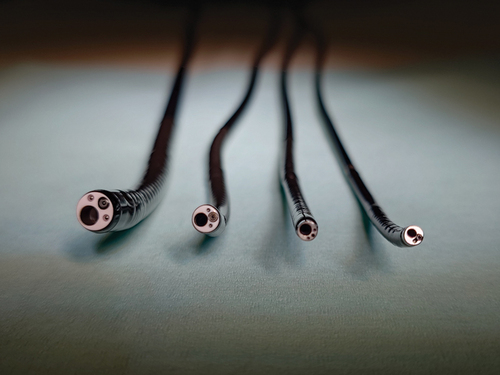
Ultrathin bronchoscopes offer the best selectivity for peripheral airways, but the size of the WC differs significantly between the conventional and newer models, 1.2 mm and 1.7 mm, respectively. The latter size allows insertion of the R-EBUS probe, which currently plays a key role in the diagnosis of PPLs, and the latter should be selected when using the ultrathin bronchoscope. In fact, in an RCT using the conventional ultrathin bronchoscope, it was reported that there was no significant improvement in the diagnostic yield by the use of navigation [Citation12]. This result differs from another RCT conducted in similar settings except for the use of a thin bronchoscope [Citation11], suggesting the importance of combined R-EBUS.
The newer ultrathin bronchoscope began with a report by Oki et al. in 2008 on the utility of the prototype [Citation79], and various comparative studies have been conducted since then. A single-center RCT comparing the ultrathin bronchoscope to a thin bronchoscope combined a GS showed a noninferior histological diagnostic yield (65% vs. 62%; difference in diagnostic yields, 3.6%; 90% CI, -7.5 to 14.7%) [Citation80] (for the detail of EBUS-GS, see section 5.2). A subsequent multicenter RCT in a similar setting demonstrated that the ultrathin bronchoscope had a significantly higher histological diagnostic yield (74% vs. 59%, p=0.044) [Citation81]. Furthermore, a multicenter RCT with a thin bronchoscope (with or without a GS) as a control group also revealed a significantly higher diagnostic yield (note that not limited to a histological yield) for the ultrathin bronchoscope (70.1% vs. 58.7%, p=0.027) [Citation82]. They also reported that of 340 cases in which PPLs were approached with a thin bronchoscope, 87 cases where lesions could not be detected by R-EBUS were switched to the ultrathin bronchoscope, and 50 of them (57.5%) became detectable by R-EBUS [Citation83]. Thus, there is no doubt that the new ultrathin bronchoscope is useful in the diagnosis of PPLs, but at the same time, the diagnostic yields of the thin bronchoscope set as a control were generally lower than the results of numerous systematic reviews and meta-analyses [Citation19,Citation34–37], and we may be cautious about the generalizability of the reports from that study group alone.
Therapeutic bronchoscopes are often too thick to be inserted beyond the level of segmental or subsegmental bronchi, making their use alone for PPLs less meaningful. On the other hand, they are used to insert conventional sampling devices for a 2.0-mm WC through auxiliary devices, such as a GS or extended working channel (EWC).
5.2. Guide sheath and catheter
Since ordinary bronchoscopes have only one WC, it is impossible to sample a lesion while confirming it with R-EBUS. Therefore, the further away from the lesion the tip of the bronchoscope is, the greater the risk of sampling errors when switching from the R-EBUS probe to sampling devices. The GS was developed to overcome this problem, and the utility of the so-called EBUS-GS method in the diagnosis of PPLs was first reported by Kurimoto et al. [Citation84]. In the prospectively enrolled single-arm study, 116 of 150 lesions (77%) were diagnosed by the EBUS-GS. The study also showed that the effect varied greatly depending on the location of the R-EBUS probe, with significantly higher diagnostic yield when the probe within than adjacent to the lesion (87% vs. 42%, p< 0.0001). Thus, when GS is combined, especially if it can be located within the lesion, precise sampling from the lesion confirmed by R-EBUS is possible. For more details on EBUS-GS, refer to the review compiled by Takashima et al. [Citation85].
It should be noted, however, that the use of GS results in a smaller inner diameter, limiting the size of sampling devices that can be inserted. A recently reported RCT comparing a thin bronchoscope with and without a GS reported a significantly higher diagnostic yield of histological specimens in the GS group (55.3% vs. 46.6%, p=0.033) [Citation86]. On the other hand, removal of the GS would allow insertion of a standard-sized forceps for the 2.0-mm WC as a series of procedures. In a retrospective analysis of cases where additional standard-sized forceps biopsies were performed after EBUS-GS, Kunimasa et al. reported that 57 (65%) were successfully diagnosed with EBUS-GS, while 15 of 31 (48%) of the failed cases were successfully diagnosed with the additional standard-sized forceps [Citation87]. In addition, another larger GS is commercially available to allow insertion of sampling devices for 2.0-mm WCs. The large GS requires a bronchoscope with a WC of 2.6 mm or larger, which inevitably has a thicker OD (for the type of flexible bronchoscopes, see section 5.1). As a result, the bronchoscope itself stops centrally, and if the target lesion is in the periphery, the selectivity is poor, and it is often necessary to assist in guiding the GS using guiding devices. Nevertheless, the specimens obtained via the large GS were clearly larger than those obtained via the small GS, and a difference in the success rate of next-generation sequencing requiring a sufficient tumor volume was reported [Citation88]. In short summary, the GS has both advantages and disadvantages, so there is no general answer as to whether it should be used or what the optimal GS size is.
On the other hand, when using ENB, the locatable guide is advanced together with the EWC, a dedicated catheter (for the history of ENB, see section 2.3), to the target lesion. This EWC is designed to allow insertion of sampling devices for 2.0-mm WCs, and requires a bronchoscope with a WC of 2.8 mm or larger. Thus, although it is similar in usability to the large GS, it differs in that the GS is only straight, while some EWCs have curved tips in multiple angle patterns in addition to the straight type. The use of these curved tip types has been reported to improve the angulation and steerability, resulting in a high diagnostic yield [Citation89]. Recently, a new ENB system using a locatable wire, which is thinner than the existing locatable guide and can be inserted into a small GS, has been developed [Citation90,Citation91].
5.3. Robotic-assisted bronchoscopy
When selecting peripheral airways toward PPLs, tapering of bronchi and various branching angles limit the range of insertion with flexible bronchoscopes. In addition, insertion of devices of different thickness and stiffness often changes the flexion of the bronchoscope, which can lead to sampling errors. These are covered to some extent by the skill and experience of the bronchoscopists, but at the same time, they are one of the factors that make equalization difficult. RAB was developed to overcome these limitations, and three platforms are currently commercially available.
The first RAB to be clinically introduced was the MonarchTM (Ethicon, Raritan, NJ, USA), consisting of a 6.0-mm outer sheath and a 4.2-mm inner scope with a 2.1-mm WC. The sheath and scope are independently bendable, and are navigated to the target lesion by applying the ENB principle, with the operation on the controller. In the first study, the inner scope could be inserted distinctly distal via any segmental bronchi using cadavers, compared to a thin bronchoscope with the same OD [Citation92]. In a multicenter pilot and feasibility study, successful lesion localization with R-EBUS was achieved in 96.2% and the diagnostic yield was 74.1% (95% CI, 61%–84%) [Citation93]. Note that the GalaxyTM (Noah Medical, San Carlos, CA, USA) is also a similar platform to the MonarchTM, providing ENB-based navigation, but differs significantly in that it also has built-in DT. In conjunction with this, it also has the ability to compensate for CT-to-body divergence.
The IonTM (Intuitive Surgical, Sunnyvale, CA, USA) consists of a 3.5-mm catheter, which functions as a 2.0-mm WC when the removable camera is removed. Therefore, unlike the other two platforms, airway view cannot be observed at the time of sampling. In addition, the IonTM uses a unique shape-sensing technology to navigate to the target lesion. This allows the shape of the catheter to be measured at high speed and thereby precisely matched to the location information obtained from the prior CT data. In the first in human trial, the target nodule was reached in 96.6%, with the overall diagnostic yield of 79.3% (95% CI, 60.3%–92.0%) [Citation94]. A later prospective cohort study in a cancer center reported similar results with the navigational success rate of 98.7% and the overall diagnostic yield of 81.7% [Citation95].
A recent systematic review and meta-analysis of RAB reported that the pooled diagnostic yield was 84.3% (95% CI, 81.1%–87.2%) [Citation96]. Lesions >2 cm, bronchus sign positive, and R-EBUS within were associated with a statistically significant increase in the ORs of diagnosis.
5.4. Bronchoscopic transparenchymal nodule access and transbronchial access tool
There are certain cases with bronchus sign negative, or even if positive, bronchoscopes cannot be wedged due to its too thin diameter and/or steep branching angle. In such cases, it would be reasonable if a linear approach could be made toward the target lesion, ignoring the bronchial tree. There are two techniques reported to puncture the bronchial wall to create a linear pathway, bronchoscopic transparenchymal nodule access (BTPNA) and transbronchial access tool (TBAT). Since both procedures require the creation of a pathway that avoids blood vessels, navigation systems are applied.
BTPNA is assisted by dedicated VBN, which shows the pathway without any vessels toward the target lesion and the point of entry at the central airway to it. After puncturing it, the created hole is balloon dilated, and a sheath is advanced toward the lesion while dissecting bluntly using an inner stylet along the indicated pathway. Following an evaluation of feasibility and safety of BTPNA in canines [Citation97], a high yield of 90.3% (28 of 31) was demonstrated for nodules implanted in canine lungs [Citation98], and a clinical trial was subsequently conducted in humans. In the first in human trial, the BTPNA procedure was completed in 10 of 12 patients (83%) and biopsies were successfully performed via the created pathway [Citation99]. The two cases where the procedure could not be completed were due to the limited range of motion of the therapeutic bronchoscope, which could not be directed to the entry point.
TBAT applies ENB and shows the pathway extending from the peripheral airway through the lung parenchyma to the target lesion. On the ENB, manually establish the point of the most direct approach from the peripheral airway to the lesion and the zones where vessels pass near the lesion, and navigate from the former to the lesion, avoiding the latter. Referring to that pathway, the peripheral bronchial wall is pierced with a guidewire, the vacated hole is dilated with a catheter, and an EWC is advanced along it toward the lesion. In the feasibility study, TBAT was achieved in 9 of 12 cases (75%), and in the 3 failure cases, the reason was that the EWC was straightened and deflected as soon as the TBAT was deployed [Citation100].
Thus, BTPNA and TBAT differ significantly in terms of whether they approach from the central or peripheral airways. Naturally, approaching from the peripheral airways shortens the pathways required and is simpler as a technique. Especially in the lung periphery, vessels are smaller, and puncturing them carries a lower risk of bleeding. Therefore, the combination of GS and TBNA (so-called GS-TBNA) can reproduce that procedure without the need to recognize blood vessels, and its utility was reported [Citation101].
5.5. Convex-probe endobronchial ultrasound bronchoscope
A convex-probe EBUS (CP-EBUS) bronchoscope provides high diagnostic accuracy if the lesion is within the reach of the bronchoscope. TBNA is usually performed (i.e., EBUS-TBNA) and has been established along with accurate nodal staging of primary lung cancer, taking advantage of its real-time guided sampling performance. A systematic review and meta-analysis reported an excellent pooled sensitivity of 93% (95% CI, 91%–94%) and specificity of 100% (95% CI, 99%–100%) [Citation102]. Although these results are for mediastinal and hilar lymph nodes, PPLs can also be targeted if they are in contact with the central airway. A systematic and meta-analysis review summarizing EBUS-TBNA for such centrally located lung tumors reported a comparable diagnostic yield of 91% (95% CI, 88%-94%) [Citation103]. In addition, a similar diagnostic yield was reported for transesophageal approaches (90% [95% CI, 82%–95%] if such lung tumors are in contact with the esophagus [Citation104].
Thus, while the CP-EBUS bronchoscopic approach is reasonable, the built-in EBUS has a larger tip than conventional flexible bronchoscopes, limiting its reach range via the airway. To overcome this limitation, a new thin CP-EBUS bronchoscope is being developed. The prototype was already reported in 2017, with a study using human ex-vivo lungs showing greater accessibility to the peripheral airways than an existing CP-EBUS bronchoscope [Citation105]. Recently, the results of a study using living pig lungs were also reported, showing that this thin CP-EBUS bronchoscope reached the peripheral airways greater than 5.5-mm and 4.8-mm conventional flexible bronchoscopes in some areas [Citation106]. It is expected that this new model, when introduced into clinical practice in the near future, will enable diagnosis of even more distal PPLs.
6. Rapid on-site evaluation
The combination of ROSE in convex probe EBUS-guided TBNA for hilar/mediastinal lesions has been established and is recommended in the ACCP guidelines because it can reduce the number of TBNA [Citation107]. On the other hand, there are no guidelines that recommend the combination of ROSE during bronchoscopy for PPLs. Nevertheless, it is essentially useful to predict the success or failure of sampling during bronchoscopic procedures. The suggestion from ROSE indicates whether we can terminate or should reattempt the sampling; therefore, it may contribute to improve the diagnostic yield and reduce the operative time.
However, according to the previous studies, it should be noted that the sensitivities and specificities of ROSE for PPLs were 88.6%–98.2% and 65.9%–100%, respectively, with large differences depending on their sampling methods and evaluators [Citation108–111]. In general, ROSE for PPLs is more difficult than that for lymph nodes because of the presence of bronchial ciliated epithelium and inflammatory cells around PPLs, which are sometimes difficult to distinguish from malignant cells. Therefore, evaluators should be careful to avoid false-positive results, which can lead to a decrease in the diagnostic yield.
Recently, some RCTs have been conducted on the combination of ROSE for PPLs. Xu et al. demonstrated an RCT in 152 cases using R-EBUS and reported that the combination of ROSE was significantly associated with the improved diagnostic yield (85.9% vs. 70.3%, p=0.016) and reduced operative time (24.6 vs. 32.4 min, p<0.001) [Citation112]. Another RCT also showed that the diagnostic yield for PPLs significantly improved with ROSE (84.0% vs. 75.5%, p=0.001), with significant impacts on lesions in the right upper lobe and ≤2 cm [Citation113]. Meanwhile, there was no reduction in the operative time (18.6 ± 6.8 vs. 15.4 ± 5.7 min).
Furthermore, a novel method of performing diagnostic RAB and subsequent surgical resection for PPLs in a single general anesthesia has been reported [Citation114]. While this method is very advantageous for malignant lesions such as primary lung cancer in that it eliminates the waiting time between the diagnosis and resection, the intraoperative diagnosis by ROSE is crucial as it is directly related to the subsequent treatment decision. If such a series of procedures becomes more widespread, the role of ROSE will also become more important.
7. Conclusions
Navigation, confirmation/verification, and sampling are complementarily related in the diagnostic bronchoscopy for PPLs (). Proper understanding of these three phases, as well as appropriate selection of approach tools according to the instruments at each facility, the characteristics of the lesion, and the purpose of the procedure, will lead to improved diagnostic accuracy of PPLs. In addition, ROSE may play an important role in decision during the bronchoscopic procedures.
Figure 5. Diagnostic strategies in bronchoscopy for peripheral pulmonary lesions. The navigation, confirmation/verification, and sampling phases are repeated as needed and complement each other in the diagnostic bronchoscopy for peripheral pulmonary lesions (PPLs). The overall view of these phases varies depending on the approach tool selected, and trade-offs (* excluding real-time guided sampling such as using a convex-prove endobronchial ultrasound bronchoscope) can occur between navigation and sampling. Rapid on-site evaluation does not directly affect the diagnostic performance, but it may play a role in deciding subsequent procedures. Optimizing each would improve the diagnostic accuracy of PPLs.
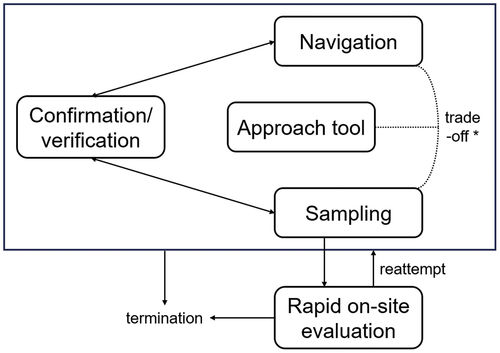
8. Expert opinion
The introduction of the flexible bronchoscope pioneered a direct approach to the peripheral airways via the bronchial tree. At the same time, the possibility of transbronchial diagnosis of PPL has emerged, but semi-blind biopsy under conventional single-plane fluoroscopy has been less accurate and has fallen behind TTNB. In recent years, with the introduction of combined instrument such as navigation, R-EBUS, DT, and CBCT, the accuracy of reaching PPLs has improved dramatically. This has been further accelerated with the advent of approach tools such as ultrathin bronchoscopes and RAB. On the other hand, the definitive diagnosis is based on pathology, which requires precise sampling. The introduction of TBNA has enabled sampling beyond the bronchial wall, and the introduction of cryobiopsy has improved diagnostic opportunities due to its superior quantity and quality of specimens. As a result, the diagnostic yield of bronchoscopy for PPLs has improved to a level comparable to that of TTNB.
Nevertheless, it is also a fact that the dissociation between reaching a lesion and diagnosing that lesion has not been fully bridged due to a variety of factors. Therefore, there is a tendency to recommend sampling using a combination of different devices, while assisted by as many instruments as possible, in order to maximize the diagnostic performance. However, the cost of installing and operating these instruments is high, and only countries and regions with favorable health economies can benefit from them. Since reports with high levels of evidence naturally come from facilities belonging to those developed groups, we need to keep a close eye on real-world data as well. In addition, many of the devices used are disposable or have a limited number of uses, and after use they become medical waste that is difficult to reuse. This is contrary to the globally stated Sustainable Development Goals, and their indiscriminate use can have a negative impact on the environment. Of course, failure to make a diagnosis, leading to increased reexaminations and delays in treatment, would be a downfall, so data on maximizing the effectiveness and minimizing the resources will be needed, depending on the characteristics of the lesion and the purpose of the procedure. It may also be possible to solve the problem if we can get rid of the dependence on pathological diagnosis, and if the time comes when analysis utilizing artificial intelligence can diagnose images obtained by the lesion from some tool inserted into the bronchus.
On the other hand, if limited to the accuracy of reaching PPLs, it has come close to 100% with the above-mentioned advances. The technique and technologies developed in the diagnosis will be carried over to the next step in the transbronchial treatment of PPLs. Various treatment devices are currently under development and promising data are emerging, and further progress and introduction is expected in the future. If diagnostic strategies during the procedure, such as ROSE, are also established, an era will eventually come when PPLs can be diagnosed and treated completely in a single bronchoscopic procedure. Further down the road, there may come a time when most of these series of procedures will be automated.
Article highlights
Bronchoscopy for peripheral pulmonary lesions (PPLs) has progressed in the past two decades, and the diagnostic yield has improved to a level approaching transthoracic needle biopsy.
The crux of successful navigation lies in precisely segmenting peripheral airways to delineate a clear pathway from the trachea to the target. Virtual bronchoscopic navigation and electromagnetic navigational bronchoscopy are representative instruments for it.
As for confirmation/verification tools, various imaging-based methods, such as radial endobronchial ultrasound, digital tomosynthesis, cone-beam computed tomography, have proven to be useful in guided bronchoscopy, providing real-time feedback on its accuracy.
The introduction of needle aspiration and cryobiopsy in addition to forceps biopsy provides additional diagnostic opportunities for PPLs.
Newer ultrathin bronchoscopes and robotic-assisted bronchoscopy allow for more peripheral access compared to conventional bronchoscopy and have shown favorable diagnostic outcomes for PPLs in clinical trials.
Predicting the sampling outcome during bronchoscopy by rapid on-site evaluation may improve the diagnostic yield and reduce the operative time
Abbreviations
BTPNA | = | bronchoscopic transparenchymal nodule access |
CBCT | = | cone-beam computed tomography |
CI | = | confidence interval |
CP-EBUS | = | convex-probe endobronchial ultrasound |
CT | = | computed tomography |
DT | = | digital tomosynthesis |
EBUS | = | endobronchial ultrasound |
EBUS-TBNA | = | endobronchial ultrasound-guided transbronchial needle aspiration |
ENB | = | electromagnetic navigation bronchoscopy |
EWC | = | extended working channel |
GGN | = | ground-glass nodule |
GS | = | guide sheath |
ILD | = | interstitial lung disease |
OD | = | outer diameter |
OR | = | odds ratio |
PPL | = | peripheral pulmonary lesion |
RAB | = | robotic-assisted bronchoscopy |
RCT | = | randomized controlled trial |
R-EBUS | = | radial endobronchial ultrasound |
TBAT | = | transbronchial access tool |
TBB | = | transbronchial biopsy |
TBNA | = | transbronchial needle aspiration |
TTNB | = | transthoracic needle biopsy |
VBN | = | virtual bronchoscopic navigation |
VF | = | virtual fluoroscopy |
WC | = | working channel |
Declarations of Interest
Y Matsumoto has received research funding from Hitachi, Ltd.; honoraria for speakers bureaus from Olympus, AstraZeneca, Novartis, COOK, AMCO, Thermo Fisher Scientific, Erbe Elektromedizin GmbH, Fujifilm, Chugai, Eli Lilly, Merck, Takeda, and Ethicon; consulting fees from Intuitive. SS Kho has received equipment support from Body Vision Medical; honoraria for speakers bureaus from Erbe Elektromedizin GmbH and Chugai. H Furuse has received honoraria for speakers bureaus from Olympus, AstraZeneca, and Erbe Elektromedizin GmbH. All of these have no direct relevance to the content of this article. The authors have no other relevant affiliations or financial involvement with any organization or entity with a financial interest in or financial conflict with the subject matter or materials discussed in the manuscript apart from those disclosed.
Reviewer Disclosures
Peer reviewers on this manuscript have no relevant financial or other relationships to disclose.
Additional information
Funding
REFERENCES
- Ikeda S, Tsuboi E, Ono R, et al. Flexible bronchofiberscope. Jpn J Clin Oncol. 2010 Sep;40(9):e55–64. 10.1093/jjco/hyq114
- Rivera MP, Mehta AC, Wahidi MM. Establishing the diagnosis of lung cancer: Diagnosis and management of lung cancer, 3rd ed: American College of Chest Physicians evidence-based clinical practice guidelines. Chest. 2013 May;143(5 Suppl):e142S–e165S.
- Bai C, Choi CM, Chu CM, et al. Evaluation of Pulmonary Nodules: Clinical Practice Consensus Guidelines for Asia. Chest. 2016 Oct;150(4):877–893.10.1016/j.chest.2016.02.650
- Reynisson PJ, Scali M, Smistad E, et al. Airway Segmentation and Centerline Extraction from Thoracic CT - Comparison of a New Method to State of the Art Commercialized Methods. PLoS One. 2015 Dec;10(12):e0144282.
- Kurimoto N, Morita K. Bronchial branch tracing. Singapore: Springer; 2020
- Kho SS, Nyanti LE, Chai CS, et al. Feasibility of manual bronchial branch reading technique in navigating conventional rEBUS bronchoscopy in the evaluation of peripheral pulmonary lesion. Clin Respir J. 2021 Jun;15(6):595–603.10.1111/crj.13297
- Zhong CH, Su ZQ, Luo WZ, et al. Hierarchical clock-scale hand-drawn mapping as a simple method for bronchoscopic navigation in peripheral pulmonary nodule. Respir Res. 2022 Sep;23(1):245.10.1186/s12931-022-02160-0
- Miyake K, Morimura O, Inoue T, et al. The Direct Oblique Method: A New Gold Standard for Bronchoscopic Navigation That is Superior to Automatic Methods. J Bronchology Interv Pulmonol. 2018 Oct;25(4):305–314. 10.1097/LBR.0000000000000512
- Chen AC, Loiselle A, Zhou L, Baty J, Misselhorn D. Localization of Peripheral Pulmonary Lesions Using a Method of Computed Tomography-Anatomic Correlation and Radial Probe Endobronchial Ultrasound Confirmation. Ann Am Thorac Soc. 2016 Sep;13(9):1586–92.
- Asano F, Matsuno Y, Shinagawa N, et al. A virtual bronchoscopic navigation system for pulmonary peripheral lesions. Chest. 2006 Aug;130(2):559–566.10.1378/chest.130.2.559
- Ishida T, Asano F, Yamazaki K, et al. Virtual bronchoscopic navigation combined with endobronchial ultrasound to diagnose small peripheral pulmonary lesions: a randomised trial. Thorax. 2011 Dec;66(12):1072–1077. doi: 10.1136/thx.2010.145490
- Asano F, Shinagawa N, Ishida T, et al. Virtual bronchoscopic navigation combined with ultrathin bronchoscopy. A randomized clinical trial. Am J Respir Crit Care Med. 2013 Aug;188(3):327–333.10.1164/rccm.201211-2104OC
- Jiang S, Xie F, Mao X, et al. The value of navigation bronchoscopy in the diagnosis of peripheral pulmonary lesions: A meta-analysis. Thorac Cancer. 2020 May;11(5):1191–1201.10.1111/1759-7714.13373
- Giri M, Puri A, Wang T, et al. Virtual bronchoscopic navigation versus non-virtual bronchoscopic navigation assisted bronchoscopy for the diagnosis of peripheral pulmonary lesions: a systematic review and meta-analysis. Ther Adv Respir Dis. 2021 Jan–Dec;15:17534666211017048.10.1177/17534666211017048
- Akulian JA, Molena D, Wahidi MM, et al. A Direct Comparative Study of Bronchoscopic Navigation Planning Platforms for Peripheral Lung Navigation: The ATLAS Study. J Bronchology Interv Pulmonol. 2022 Jul;29(3):171–178. 10.1097/LBR.0000000000000806
- Schwarz Y, Mehta AC, Ernst A, et al. Electromagnetic navigation during flexible bronchoscopy. Respiration. 2003 Sep–Oct;70(5):516–522.10.1159/000074210
- Schwarz Y, Greif J, Becker HD, et al. Real-time electromagnetic navigation bronchoscopy to peripheral lung lesions using overlaid CT images: the first human study. Chest. 2006 Apr;129(4):988–994.10.1378/chest.129.4.988
- Folch EE, Pritchett MA, Nead MA, et al. Electromagnetic navigation bronchoscopy for peripheral pulmonary lesions: one-year results of the prospective, multicenter NAVIGATE study. J Thorac Oncol. 2019 Mar;14(3):445–458. doi: 10.1016/j.jtho.2018.11.013
- Wang Memoli JS, Nietert PJ, Silvestri GA Meta-analysis of guided bronchoscopy for the evaluation of the pulmonary nodule. Chest. 2012 Aug;142(2):385–393.10.1378/chest.11-1764
- Gex G, Pralong JA, Combescure C, et al. Diagnostic yield and safety of electromagnetic navigation bronchoscopy for lung nodules: a systematic review and meta-analysis. Respiration. 2014;87(2):165–176. doi: 10.1159/000355710
- Folch EE, Labarca G, Ospina-Delgado D, et al. Sensitivity and Safety of Electromagnetic Navigation Bronchoscopy for Lung Cancer Diagnosis: Systematic Review and Meta-analysis. Chest. 2020 Oct;158(4):1753–1769.10.1016/j.chest.2020.05.534
- Makris D, Scherpereel A, Leroy S, et al. Electromagnetic navigation diagnostic bronchoscopy for small peripheral lung lesions. Eur Respir J. 2007 Jun;29(6):1187–1192. 10.1183/09031936.00165306
- Dunn BK, Blaj M, Stahl J, et al. Evaluation of Electromagnetic Navigational Bronchoscopy Using Tomosynthesis-Assisted Visualization, Intraprocedural Positional Correction and Continuous Guidance for Evaluation of Peripheral Pulmonary Nodules. J Bronchology Interv Pulmonol. 2023 Jan;30(1):16–23. 10.1097/LBR.0000000000000839
- Aboudara M, Roller L, Rickman O, et al. Improved diagnostic yield for lung nodules with digital tomosynthesis-corrected navigational bronchoscopy: Initial experience with a novel adjunct. Respirology. 2020 Feb;25(2):206–213.10.1111/resp.13609
- Avasarala SK, Roller L, Katsis J, et al. Sight unseen: diagnostic yield and safety outcomes of a novel multimodality navigation bronchoscopy platform with real-time target acquisition. Respiration. 2022;101(2):166–173. doi: 10.1159/000518009
- Gmehlin CG, Kurman JS, Benn BS Size and vision: Impact of fluoroscopic navigation, digital tomosynthesis, and continuous catheter tip tracking on diagnostic yield of small, bronchus sign negative lung nodules. Respir Med. 2022 Oct;202:106941. 10.1016/j.rmed.2022.106941
- Lai RS, Lee SS, Ting YM, et al. Diagnostic value of transbronchial lung biopsy under fluoroscopic guidance in solitary pulmonary nodule in an endemic area of tuberculosis. Respir Med. 1996 Mar;90(3):139–143. 10.1016/S0954-6111(96)90155-9
- Nakai T, Izumo T, Matsumoto Y, et al. Virtual fluoroscopy during transbronchial biopsy for locating ground-glass nodules not visible on X-ray fluoroscopy. J Thorac Dis. 2017 Dec;9(12):5493–5502. 10.21037/jtd.2017.10.01
- Abe Y, Miyake K, Shiroyama T, et al. Virtual fluoroscopic preprocedural planning using Ziostation2 for transbronchial biopsy: A prospective self-controlled study. Respir Investig. 2023 Mar;61(2):157–163. 10.1016/j.resinv.2022.12.005
- Hürter T, Hanrath P Endobronchial sonography: feasibility and preliminary results. Thorax. 1992 Jul;47(7):565–567.10.1136/thx.47.7.565
- Goldberg BB, Steiner RM, Liu JB, et al. US-assisted bronchoscopy with use of miniature transducer-containing catheters. Radiology. 1994 Jan;190(1):233–237.10.1148/radiology.190.1.8259411
- Herth FJ, Ernst A, Becker HD Endobronchial ultrasound-guided transbronchial lung biopsy in solitary pulmonary nodules and peripheral lesions. Eur Respir J. 2002 Oct;20(4):972–974. 10.1183/09031936.02.00032001
- Kurimoto N, Murayama M, Yoshioka S, et al. Analysis of the internal structure of peripheral pulmonary lesions using endobronchial ultrasonography. Chest. 2002 Dec;122(6):1887–1894.10.1378/chest.122.6.1887
- Steinfort DP, Khor YH, Manser RL, et al. Radial probe endobronchial ultrasound for the diagnosis of peripheral lung cancer: systematic review and meta-analysis. Eur Respir J. 2011 Apr;37(4):902–910.10.1183/09031936.00075310
- Zhan P, Zhu QQ, Miu YY, et al. Comparison between endobronchial ultrasound-guided transbronchial biopsy and CT-guided transthoracic lung biopsy for the diagnosis of peripheral lung cancer: a systematic review and meta-analysis. Transl Lung Cancer Res. 2017 Feb;6(1):23–34. 10.21037/tlcr.2017.01.01
- Ali MS, Trick W, Mba BI, et al. Radial endobronchial ultrasound for the diagnosis of peripheral pulmonary lesions: A systematic review and meta-analysis. Respirology. 2017 Apr;22(3):443–453. 10.1111/resp.12980
- Sainz Zuñiga PV, Vakil E, Molina S, Bassett RL Jr, Ost DE. Sensitivity of Radial Endobronchial Ultrasound-Guided Bronchoscopy for Lung Cancer in Patients With Peripheral Pulmonary Lesions: An Updated Meta-analysis. Chest. 2020 Apr;157(4):994–1011.
- Chen A, Misselhorn D Biopsy of peripheral pulmonary lesions using real-time radial endobronchial ultrasound and a “double-barrel” bronchoscope. J Bronchology Interv Pulmonol. 2009 Jan;16(1):22–24. 10.1097/LBR.0b013e3181966b2b
- Yarmus LB, Mallow C, Pastis N, et al. First-in-human use of a hybrid real-time ultrasound-guided fine-needle acquisition system for peripheral pulmonary lesions: a multicenter Pilot study. Respiration. 2019;98(6):527–533. doi: 10.1159/000504025
- Izumo T, Sasada S, Chavez C, et al. Radial endobronchial ultrasound images for ground-glass opacity pulmonary lesions. Eur Respir J. 2015 Jun;45(6):1661–1668.10.1183/09031936.00167914
- Machida H, Yuhara T, Tamura M, et al. Whole-Body Clinical Applications of Digital Tomosynthesis. Radiographics. 2016 May–Jun;36(3):735–750.10.1148/rg.2016150184
- Dobbins JT 3rd, Godfrey DJ. Digital x-ray tomosynthesis: current state of the art and clinical potential. Phys Med Biol. 2003 Oct;48(19):R65–106.
- Low SW, Lentz RJ, Chen H, et al. Shape-Sensing Robotic-Assisted Bronchoscopy vs Digital Tomosynthesis-Corrected Electromagnetic Navigation Bronchoscopy: A Comparative Cohort Study of Diagnostic Performance. Chest. 2023 Apr;163(4):977–984.10.1016/j.chest.2022.10.019
- Cicenia J, Bhadra K, Sethi S, Nader DA, Whitten P, Hogarth DK. Augmented Fluoroscopy: A New and Novel Navigation Platform for Peripheral Bronchoscopy. J Bronchology Interv Pulmonol. 2021 Apr;28(2):116–123.
- Pritchett MA Prospective Analysis of a Novel Endobronchial Augmented Fluoroscopic Navigation System for Diagnosis of Peripheral Pulmonary Lesions. J Bronchology Interv Pulmonol. 2021 Apr;28(2):107–115. 10.1097/LBR.0000000000000700
- Bhadra K, Setser RM, Condra W, Bader BA, David S. A Cone Beam CT Bronchoscopy Study of the Ultrathin Cryoprobe for Biopsy of Peripheral Lung Lesions. J Bronchology Interv Pulmonol. 2023 Jul. [ Epub ahead of print]
- Casal RF, Sarkiss M, Jones AK, et al. Cone beam computed tomography-guided thin/ultrathin bronchoscopy for diagnosis of peripheral lung nodules: a prospective pilot study. J Thorac Dis. 2018 Dec;10(12):6950–6959.10.21037/jtd.2018.11.21
- Verhoeven RLJ, van der Sterren W, Kong W, Langereis S, van der Tol P, van der Heijden EHFM. Cone-beam CT and Augmented Fluoroscopy-guided Navigation Bronchoscopy: Radiation Exposure and Diagnostic Accuracy Learning Curves. J Bronchology Interv Pulmonol. 2021 Oct;28(4):262–271.
- Mahiat C, Bondue B, Taton O, et al. Emphysema seen through a mini-camera during an electromagnetic navigation bronchoscopy guided by Cone-beam computed tomography. Respiration. 2023;102(7):523–525. doi: 10.1159/000530910
- Kinoshita T, Terai H, Ikemura S, et al. Observation of peripheral airways using ultra-thin fiberscope. Respirology. 2023 Sep;28(9):881–884.10.1111/resp.14547
- Huseini T, Soder SA, Katz A, Mihalik T, Liberman M; Department of Thoracic Surgery and Lung Transplantation Working Group. Evaluation of deployment capability of a novel outside-the-scope, detachable catheter system for ablation of lung lesions in ex vivo human lung models. JTCVS Tech. 2022 Jul;15:147–154.
- Tsuboi E, Ikeda S, Tajima M, et al. Transbronchial biopsy smear for diagnosis of peripheral pulmonary carcinomas. Cancer. 1967 May;20(5):687–698.10.1002/1097-0142(1967)20:5<687::AID-CNCR2820200521>3.0.CO;2-K
- Gaeta M, Pandolfo I, Volta S, et al. Bronchus sign on CT in peripheral carcinoma of the lung: value in predicting results of transbronchial biopsy. AJR Am J Roentgenol. 1991 Dec;157(6):1181–1185. 10.2214/ajr.157.6.1950861
- Guvenc C, Yserbyt J, Testelmans D, et al. Computed tomography characteristics predictive for radial EBUS-miniprobe-guided diagnosis of pulmonary lesions. J Thorac Oncol. 2015 Mar;10(3):472–478. 10.1097/JTO.0000000000000410
- Imabayashi T, Matsumoto Y, Uchimura K, et al. Computed Tomography Bronchus Sign Subclassification during Radial Endobronchial Ultrasound-Guided Transbronchial Biopsy: A Retrospective Analysis. Diagnostics (Basel). 2023 Mar;13(6):1064.10.3390/diagnostics13061064
- Yamada N, Yamazaki K, Kurimoto N, et al. Factors related to diagnostic yield of transbronchial biopsy using endobronchial ultrasonography with a guide sheath in small peripheral pulmonary lesions. Chest. 2007 Aug;132(2):603–608.10.1378/chest.07-0637
- Ali MS, Sethi J, Taneja A, et al. Computed Tomography Bronchus Sign and the Diagnostic Yield of Guided Bronchoscopy for Peripheral Pulmonary Lesions. A Systematic Review and Meta-Analysis. Ann Am Thorac Soc. 2018 Aug;15(8):978–987. 10.1513/AnnalsATS.201711-856OC
- Wang KP, Haponik EF, Britt EJ, et al. Transbronchial needle aspiration of peripheral pulmonary nodules. Chest. 1984 Dec;86(6):819–823.10.1378/chest.86.6.819
- Mondoni M, Sotgiu G, Bonifazi M, et al. Transbronchial needle aspiration in peripheral pulmonary lesions: a systematic review and meta-analysis. Eur Respir J. 2016 Jul;48(1):196–204.
- Chao TY, Chien MT, Lie CH, et al. Endobronchial ultrasonography-guided transbronchial needle aspiration increases the diagnostic yield of peripheral pulmonary lesions: a randomized trial. Chest. 2009 Jul;136(1):229–236.10.1378/chest.08-0577
- Ost DE, Ernst A, Lei X, et al. Diagnostic Yield and Complications of Bronchoscopy for Peripheral Lung Lesions. Results of the AQuIRE Registry. Am J Respir Crit Care Med. 2016 Jan;193(1):68–77.10.1164/rccm.201507-1332OC
- Sumi T, Shijubou N, Sawai T, et al. Transbronchial needle aspiration with endobronchial ultrasonography and ultrathin bronchoscopy for peripheral pulmonary lesions. Respir Investig. 2021 Nov;59(6):766–771. 10.1016/j.resinv.2021.04.004
- Chen AC, Machuzak M, Cheng G, et al. Bronchoscopic evaluation of a steerable needle for simulated tumor targets in the lung periphery: a feasibility study (bullseye). Respiration. 2023;102(5):370–376. doi: 10.1159/000529245
- Du Rand IA, Barber PV, Goldring J, et al. British Thoracic Society guideline for advanced diagnostic and therapeutic flexible bronchoscopy in adults. Thorax. 2011 Nov;66 Suppl 3:iii1–21.
- Hetzel J, Hetzel M, Hasel C, et al. Old meets modern: the use of traditional cryoprobes in the age of molecular biology. Respiration. 2008;76(2):193–197. doi: 10.1159/000135934
- Hetzel J, Eberhardt R, Herth FJ, et al. Cryobiopsy increases the diagnostic yield of endobronchial biopsy: a multicentre trial. Eur Respir J. 2012 Mar;39(3):685–690. 10.1183/09031936.00033011
- Raghu G, Remy-Jardin M, Richeldi L, et al. Am J Respir Crit Care Med. 2022 May;205(9):e18–e47. Idiopathic Pulmonary Fibrosis (an Update) and Progressive Pulmonary Fibrosis in Adults: An Official ATS/ERS/JRS/ALAT Clinical Practice Guideline 10.1164/rccm.202202-0399ST
- Sryma PB, Mittal S, Madan NK, et al. Efficacy of Radial Endobronchial Ultrasound (R-EBUS) guided transbronchial cryobiopsy for peripheral pulmonary lesions (PPL…s): A systematic review and meta-analysis. Pulmonology. 2023 Jan–Feb;29(1):50–64.
- Schuhmann M, Bostanci K, Bugalho A, et al. Endobronchial ultrasound-guided cryobiopsies in peripheral pulmonary lesions: a feasibility study. Eur Respir J. 2014 Jan;43(1):233–239. 10.1183/09031936.00011313
- Matsumoto Y, Nakai T, Tanaka M, et al. Diagnostic Outcomes and Safety of Cryobiopsy Added to Conventional Sampling Methods: An Observational Study. Chest. 2021 Nov;160(5):1890–1901.10.1016/j.chest.2021.05.015
- Furuse H, Matsumoto Y, Nakai T, et al. Diagnostic efficacy of cryobiopsy for peripheral pulmonary lesions: A propensity score analysis. Lung Cancer. 2023 Apr;178:220–228. 10.1016/j.lungcan.2023.02.022
- Tanaka M, Matsumoto Y, Imabayashi T, et al. Diagnostic value of a new cryoprobe for peripheral pulmonary lesions: a prospective study. BMC Pulm Med. 2022 Jun;22(1):226.10.1186/s12890-022-02003-0
- Kim SH, Mok J, Kim S, et al. Clinical outcomes of transbronchial cryobiopsy using a 1.1-mm diameter cryoprobe for peripheral lung lesions - A prospective pilot study. Respir Med. 2023 Oct;217:107338. 10.1016/j.rmed.2023.107338
- Oki M, Saka H, Kogure Y, et al. Ultrathin bronchoscopic cryobiopsy of peripheral pulmonary lesions. Respirology. 2023 Feb;28(2):143–151.10.1111/resp.14360
- Nakai T, Watanabe T, Kaimi Y, et al. Diagnostic utility and safety of non-intubated cryobiopsy technique using a novel ultrathin cryoprobe in addition to conventional biopsy techniques for peripheral pulmonary lesions. Respiration. 2023;102(7):503–514. doi: 10.1159/000531010
- Benn BS, Gmehlin CG, Kurman JS, et al. Does transbronchial lung cryobiopsy improve diagnostic yield of digital tomosynthesis-assisted electromagnetic navigation guided bronchoscopic biopsy of pulmonary nodules? A pilot study. Respir Med. 2022 Oct;202:106966. 10.1016/j.rmed.2022.106966
- Oberg CL, Lau RP, Folch EE, et al. Novel Robotic-Assisted Cryobiopsy for Peripheral Pulmonary Lesions. Lung. 2022 Dec;200(6):737–745.10.1007/s00408-022-00578-3
- Orr L, Krochmal R, Sonti R, et al. Comparison of the GenCut Core Biopsy System to Transbronchial Biopsy Forceps for Flexible Bronchoscopic Lung Biopsy. J Bronchology Interv Pulmonol. 2022 Apr;29(2):140–145. 10.1097/LBR.0000000000000803
- Oki M, Saka H, Kitagawa C, et al. Novel thin bronchoscope with a 1.7-mm working channel for peripheral pulmonary lesions. Eur Respir J. 2008 Aug;32(2):465–471. 10.1183/09031936.00169107
- Oki M, Saka H, Kitagawa C, et al. Randomized study of endobronchial ultrasound-guided transbronchial biopsy: thin bronchoscopic method versus guide sheath method. J Thorac Oncol. 2012 Mar;7(3):535–541. 10.1097/JTO.0b013e3182417e60
- Oki M, Saka H, Ando M, et al. Ultrathin bronchoscopy with multimodal devices for peripheral pulmonary lesions. A randomized trial. Am J Respir Crit Care Med. 2015 Aug;192(4):468–476. doi: 10.1164/rccm.201502-0205OC
- Oki M, Saka H, Asano F, et al. Use of an Ultrathin vs Thin Bronchoscope for Peripheral Pulmonary Lesions: A Randomized Trial. Chest. 2019 Nov;156(5):954–964.10.1016/j.chest.2019.06.038
- Oki M, Saka H, Himeji D, et al. Value of adding ultrathin bronchoscopy to thin bronchoscopy for peripheral pulmonary lesions: A multicentre prospective study. Respirology. 2023 Feb;28(2):152–158.10.1111/resp.14397
- Kurimoto N, Miyazawa T, Okimasa S, et al. Endobronchial ultrasonography using a guide sheath increases the ability to diagnose peripheral pulmonary lesions endoscopically. Chest. 2004 Sep;126(3):959–965. doi: 10.1378/chest.126.3.959
- Takashima Y, Oki M Endobronchial ultrasound with a guide sheath during bronchoscopy for peripheral pulmonary lesions. Expert Rev Respir Med. 2023 Jul–Dec;17(10):929–936. 10.1080/17476348.2023.2278605
- Oki M, Saka H, Imabayashi T, et al. Guide sheath versus non-guide sheath method for endobronchial ultrasound-guided biopsy of peripheral pulmonary lesions: a multicentre randomised trial. Eur Respir J. 2022 May;59(5):2101678.10.1183/13993003.01678-2021
- Kunimasa K, Tachihara M, Tamura D, et al. Diagnostic utility of additional conventional techniques after endobronchial ultrasonography guidance during transbronchial biopsy. Respirology. 2016 Aug;21(6):1100–1105.10.1111/resp.12813
- Murakami S, Yokose T, Nemoto D, et al. Suitability of Bronchoscopic Biopsy Tissue Samples for Next-Generation Sequencing. Diagnostics (Basel). 2021 Feb;11(3):391.10.3390/diagnostics11030391
- Mukherjee S, Chacey M Diagnostic Yield of Electromagnetic Navigation Bronchoscopy Using a Curved-tip Catheter to Aid in the Diagnosis of Pulmonary Lesions. J Bronchology Interv Pulmonol. 2017 Jan;24(1):35–39. 10.1097/LBR.0000000000000326
- Zheng X, Cao L, Zhang Y, et al. A Novel Electromagnetic Navigation Bronchoscopy System for the Diagnosis of Peripheral Pulmonary Nodules: A Randomized Clinical Trial. Ann Am Thorac Soc. 2022 Oct;19(10):1730–1739. 10.1513/AnnalsATS.202109-1071OC
- Li Y, Chen W, Xie F, et al. Novel electromagnetic navigation bronchoscopy system for the diagnosis of peripheral pulmonary nodules: a prospective, multicentre study. Thorax. 2023 Dec;78(12):1197–1205.10.1136/thorax-2022-219664
- Chen AC, Gillespie CT Robotic Endoscopic Airway Challenge: REACH Assessment. Ann Thorac Surg. 2018 Jul;106(1):293–297. 10.1016/j.athoracsur.2018.01.051
- Chen AC, Pastis NJ Jr, Mahajan AK, et al. Robotic Bronchoscopy for Peripheral Pulmonary Lesions: A Multicenter Pilot and Feasibility Study (BENEFIT). Chest. 2021 Feb;159(2):845–852.
- Fielding DIK, Bashirzadeh F, Son JH, et al. First human use of a new robotic-assisted fiber optic sensing navigation system for small peripheral pulmonary nodules. Respiration. 2019;98(2):142–150. doi: 10.1159/000498951
- Kalchiem-Dekel O, Connolly JG, Lin IH, et al. Shape-Sensing Robotic-Assisted Bronchoscopy in the Diagnosis of Pulmonary Parenchymal Lesions. Chest. 2022 Feb;161(2):572–582.10.1016/j.chest.2021.07.2169
- Ali MS, Ghori UK, Wayne MT, ShostakE, De Cardenas J. Diagnostic Performance and Safety Profile of Robotic-assisted Bronchoscopy: A Systematic Review and Meta-Analysis. Ann Am Thorac Soc. 2023 Dec;20(12):1801–1812.
- Silvestri GA, Herth FJ, Keast T, et al. Feasibility and safety of bronchoscopic transparenchymal nodule access in canines: a new real-time image-guided approach to lung lesions. Chest. 2014 Apr;145(4):833–838.10.1378/chest.13-1971
- Sterman DH, Keast T, Rai L, et al. High yield of bronchoscopic transparenchymal nodule access real-time image-guided sampling in a novel model of small pulmonary nodules in canines. Chest. 2015 Mar;147(3):700–707.10.1378/chest.14-0724
- Herth FJ, Eberhardt R, Sterman D, et al. Bronchoscopic transparenchymal nodule access (BTPNA): first in human trial of a novel procedure for sampling solitary pulmonary nodules. Thorax. 2015 Apr;70(4):326–332.10.1136/thoraxjnl-2014-206211
- Bowling MR, Brown C, Anciano CJ Feasibility and Safety of the Transbronchial Access Tool for Peripheral Pulmonary Nodule and Mass. Ann Thorac Surg. 2017 Aug;104(2):443–449. 10.1016/j.athoracsur.2017.02.035
- Hayama M, Izumo T, Chavez C, et al. Additional transbronchial needle aspiration through a guide sheath for peripheral pulmonary lesions that cannot be detected by radial EBUS. Clin Respir J. 2017 Nov;11(6):757–764.10.1111/crj.12413
- Gu P, Zhao YZ, Jiang LY, et al. Endobronchial ultrasound-guided transbronchial needle aspiration for staging of lung cancer: a systematic review and meta-analysis. Eur J Cancer. 2009 May;45(8):1389–1396.10.1016/j.ejca.2008.11.043
- Kuijvenhoven JC, Leoncini F, Crombag LC, et al. Endobronchial ultrasound for the diagnosis of centrally located lung tumors: a systematic review and meta-analysis. Respiration. 2020;99(5):441–450. doi: 10.1159/000500363
- Korevaar DA, Colella S, Spijker R, et al. Esophageal endosonography for the diagnosis of intrapulmonary tumors: a systematic review and meta-analysis. Respiration. 2017;93(2):126–137. doi: 10.1159/000452958
- Patel P, Wada H, Hu HP, et al. First Evaluation of the New Thin Convex Probe Endobronchial Ultrasound Scope: A Human Ex Vivo Lung Study. Ann Thorac Surg. 2017 Apr;103(4):1158–1164. 10.1016/j.athoracsur.2016.09.023
- Ishiwata T, Inage T, Gregor A, et al. Preclinical evaluation of thin convex probe endobronchial ultrasound-guided transbronchial needle aspiration for intrapulmonary lesions. Transl Lung Cancer Res. 2022 Jul;11(7):1292–1301.10.21037/tlcr-22-120
- Wahidi MM, Herth F, Yasufuku K, et al. Technical Aspects of Endobronchial Ultrasound-Guided Transbronchial Needle Aspiration: CHEST Guideline and Expert Panel Report. Chest. 2016 Mar;149(3):816–835.10.1378/chest.15-1216
- Chen CH, Cheng WC, Wu BR, et al. Improved diagnostic yield of bronchoscopy in peripheral pulmonary lesions: combination of radial probe endobronchial ultrasound and rapid on-site evaluation. J Thorac Dis. 2015 Dec;7(Suppl 4):S418–25.10.3978/j.issn.2072-1439.2015.12.13
- Izumo T, Matsumoto Y, Sasada S, et al. Utility of rapid on-site cytologic evaluation during endobronchial ultrasound with a guide sheath for peripheral pulmonary lesions. Jpn J Clin Oncol. 2017 Mar;47(3):221–225. 10.1093/jjco/hyw180
- Shikano K, Ishiwata T, Saegusa F, et al. Feasibility and accuracy of rapid on-site evaluation of touch imprint cytology during transbronchial biopsy. J Thorac Dis. 2020 Jun;12(6):3057–3064.10.21037/jtd-20-671
- Wan T, Li Y, Hu Q, et al. Diagnostic value of rapid on-site evaluation during endobronchial ultrasound with a guide sheath for peripheral pulmonary lesions. Cytopathology. 2020 Jan;31(1):16–21.10.1111/cyt.12776
- Xu C, Liu W, Wang W, et al. Diagnostic value of endobronchial ultrasound combined with rapid on-site evaluation of transbronchial lung biopsy for peripheral pulmonary lesions. Diagn Cytopathol. 2021 Jun;49(6):706–710. 10.1002/dc.24725
- Qi JC, Liao L, Zhao Z, et al. Impact of rapid on-site evaluation combined with endobronchial ultrasound and virtual bronchoscopic navigation in diagnosing peripheral lung lesions. BMC Pulm Med. 2022 Mar;22(1):117.10.1186/s12890-022-01917-z
- Brownlee AR, Watson JJJ, Akhmerov A, et al. Robotic navigational bronchoscopy in a thoracic surgery practice: Leveraging technology in the management of pulmonary nodules. JTCVS Open. 2023 Jul;16:1–6.10.1016/j.xjon.2023.07.004