Abstract
This state-of-the-art report presents the basic concepts of some of the thermo-hydro (TH) and thermo-hydro-mechanical (THM) wood processes that are in use today, i.e. heat treatment, compression of wood in the longitudinal or transverse direction and wood welding. The reasons for the growing interest in TH and THM techniques are discussed, and the development of the different concepts, from first ideas to current status, is briefly presented. The physical and chemical changes that occur in wood during TH and THM processing according to the latest research are also presented. Finally, developments that are close to or already have an industrial application are presented, and the challenges for further development of the heat treatment, compression and wood welding processes are discussed. The TH processing of wood is based entirely on water and heat, and a THM process incorporates an additional mechanical force. The purpose of wood transformation by a TH or a THM process is to improve the intrinsic wood properties, to acquire a form and functionality desired by engineers without changing its eco-friendly characteristics or hindering its further use in the total material life cycle. Only a few of the recently developed techniques, e.g. heat treatment, wood welding and various densification applications, have been industrialized to some extent. There are many reasons for this relatively low transfer of the research results to a full up-scaled industrial production. Some of them are related to unsolved problems at the laboratory level on small-sized samples and others are related to the scaling-up processes in industry. Furthermore, the ageing of heated wood leads to deterioration with time, in some cases there is an unpleasant odour, the strength of the wood decreases substantially and the wood becomes more brittle. These are new challenges which need to be resolved by the collaboration of researchers from the different scientific domains of academia, research institutes and industry.
Reasons for thermo-hydro-mechanical (THM) processing
Today, forestry and forestry-related industries are sharply in the focus of discussions concerning the major challenges for the future. A great challenge for humankind is to develop a sustainable society. Such a society requires the use of renewable materials, a large reduction in the use of non-renewable natural resources and a large reduction in environmental impacts, including a drastic reduction of greenhouse gas emissions. One way of reducing the emission of carbon dioxide is to use a larger portion of wood products and to increase the life time of these products so that the carbon is stored over a longer period of time. Another possibility is to replace energy-intensive materials with wood and wood-based products (Mahapatra and Gustavsson Citation2008). Forest resources and wood products can, therefore, play an important role in a long-term strategy for sustainable development and lessen man's impact on environment. Replacing non-renewable materials with wood-based ones is hence crucial for the development of a sustainable society, and new knowledge is required to show how resource-efficient processes for wood products with a low environmental impact can be designed and implemented. In the future, wood products also have to be refined so that all the life cycle phases – production, operation, retrofitting and end-of-life – are considered and optimized as a whole, including the energy and material chains from forest to final services. The development of thermo-hydro (TH) and THM techniques and processes for wood can be a key factor in this context.
For the recycling of wood and wood fibres it is important that the components, i.e. chemicals or other materials, added to wood in the different process stages do not prevent the use of the wood in the next cycle or prevent the return of the ash to the natural life cycle. In this respect, the TH and THM processes have great advantages in adding only energy and water to the process.
For wood to be competitive against other materials in a broad sense, the environmental advantages of wood alone are not enough, i.e. that wood shows low emissions of carbon dioxide according to calculations based on life-cycle assessment (LCA) criteria (see Ekvall Citation2006, Gustavsson and Sathre Citation2011). Wood must also be competitive for its technical properties and show not only a high material utilisation while providing a competitive economic yield during usage. Some TH or THM processes (e.g. wood bending) have a high material yield and consume little energy, and thus are economically advantageous compared to the processing of other materials (Navi and Sandberg Citation2012). However, the technical and environmental performance of TH or THM processed wood products is not always competitive with products for other materials and it must therefore be improved.
The results of studies in different fields of TH or THM processing indicate that the properties of wood such as its shape stability, strength, surface hardness (in THM process only) and durability can be improved (Navi and Sandberg Citation2012). Potential areas of application for TH or THM products are now being identified in the building industry, furniture manufacture, with longer service lives of wood products through increased durability and stability. TH and THM processing as a base for improving intrinsic wood properties and developing new product-market combinations are now of great interest in the industry.
The concepts of TH and THM processing
TH is used on wood to enhance wood properties, to dissipate internal stresses, to dry and to soften (plasticize) the wood. Common TH processes are for example heat treatment, the drying of wood under high temperature and of wood-based composites or veneer products. THM processes, on the other hand, are employed in wood shaping and moulding, welding of wood by friction, improving wood quality through densification, embossing and wood bending. A number of these techniques have been known for a long time. The result of the TH or THM processing depends on whether the process is open or closed. It is not possible to adequately control the process parameters, e.g. the moisture content of the elements, during processing in an open system. In a closed system, all the process parameters involved, such as type of medium, time, temperature and humidity can be controlled accurately throughout the treatment. A traditional example is the thermal degradation of wood which leads to totally different results depending on whether air is present or not. Generally, heating without air is called pyrolysis, e.g. producing charcoal in a closed system. Heating with access to air is called combustion where the combustion may be complete (e.g. burning in a fireplace, i.e. an open system) or incomplete (e.g. the production of producer gas or charring in a charcoal stack). The different TH and THM processes can be performed in either an open or a closed process and generally with totally different results.
Heat treatment and the accelerated ageing of wood
Heat-treatment processes have been known for a very long time and they include several different methods. In ancient Africa, natives hardened wooden spears by placing a sharpened straight wooden stick into glowing coals and then pounding the burned end with a rock, repeating this process many times until the end was sharp and hard (Rowell Citation2009). The heat-treatment process can also give changes in acoustic properties of wood and that may be particularly of interest in musical instruments (CEN Citation2008). In most industrialized processes today, heat treatment involves temperatures between 150 and 260°C for times ranging from a few minutes to several hours. The goal when treating wood is to achieve increased dimensional stability and resistance to biological degradation. The heat-treatment process can also be followed by compression in the axial or transverse direction of the wood, and this is discussed in the next two sections. The heat-treatment process is in most cases performed in a vacuum, in air or with an inert gas such as nitrogen. Pre-heated oil can also be used, in which case the oil acts as a heat-transfer medium and also excludes oxygen from the wood. The heat treatment of wood above 300°C is of limited practical value due to the severe degradation of the wood material (Hill Citation2006). Accelerated ageing of wood is a heat-treatment process using a mild temperature, i.e. a treatment temperature in the range of 100–150°C, in most cases under controlled relative humidity and pressure.
During the twentieth century, there have been several reports of wood heat treatment (see Stingl et al. Citation2002, Navi and Sandberg Citation2012). These studies began particularly after it had been observed that wood dried at a high temperature changes colour, has a greater dimensional stability and less hygroscopicity (Tiemann Citation1915, Koehler and Pillow Citation1925, Pillow Citation1929, Stamm and Hansen Citation1937). After the First World War, comprehensive studies were made on the effect of the kiln drying temperatures on the strength of wood for the aviation industry in the USA (Tiemann Citation1920, Wilson Citation1920). Stamm et al. (Citation1946) reported the first systematic experiments on wood heat treatment illustrating an increase in dimensional stability and in the decay resistance of wood treated at temperature between 120 and 320°C. To minimize the oxidation and its effect on strength and to make possible rapid heat transfer, a method of heating wood beneath the surface of molten metal was adopted (Stamm Citation1942). It was also found that the loss in strength per unit anti-shrink efficiency (for definition see Ibach Citation2010) obtained was greater when the wood was heated in the presence of air than when air was excluded (Stamm Citation1956). From this, they developed a product called Staybwood. However, Stamm (Citation1964) later reported that although the dimensional stability as well as the elimination of shape memory of compressed wood was improved by heating, other important properties such as wood strength were reduced to an unacceptable level and the product was never commercialized. Maclean (Citation1951, Citation1953) studied the influence of the heat-treatment conditions on wood properties and found that wood degrades faster when heated in either steam or water than when heated under dry conditions.
In the 1970s, Burmester studied the effects of temperature, pressure, moisture content and time in order to empirically optimize conditions for the heat stabilization of oak, beech, spruce and pine wood, and a heat-treatment method called Feuchte–Wärme–Druck was developed. Within this framework, the optimum conditions for pine wood were found to be a moisture content of 20–30% and a temperature of 160°C for 15 hours in a closed vessel (Burmester Citation1973, Citation1974a,Citationb). Under such conditions, the development of considerable resistance to brown-rot fungus was reported, and the strength reduction was stated to be insignificant.
Heat treatment significantly influences the properties of the wood, e.g. its hygroscopicity, decay resistance, durability, strength and dimensional stability. To increase the knowledge of the chemical changes occurring in heat-treated wood, numerous investigations were started to clarify the chemical degradation of wood constituents during heat treatment. There are a number of difficulties in describing the changes that take place when wood is heated. A range of chemical reactions take place simultaneously, and these are both endothermic and exothermic reactions, making the determination of the onset temperatures for the different reactions nearly impossible. The analysis is complicated further by interactions between reactions involving different constituents. This means that an analysis of the reactions involving an isolated derivative of one of the components can be very different from what actually takes place inside the wood. There are not only interactions between the components inside the wood but also interactions between the wood and the treatment atmosphere. The rate of thermal degradation is different for the different constituents of wood: it is highest for hemicelluloses, much lower for cellulose and lowest for lignin (Stamm Citation1956, Bourgois and Guyonnet Citation1988, Yildiz et al. Citation2006, Esteves et al. Citation2008b). In general, hardwoods are less thermally stable than softwoods, and this is attributable to differences in the hemicellulose content and composition (Hill Citation2006).
Because of the loss of hygroscopic hemicellulose sugars and their conversion to less hygroscopic furan-based polymers (predominantly furfural and hydroxymethylfurfural) during heat treatment, the equilibrium moisture content is reduced to about half the value of the untreated wood (see Teichgräber Citation1966, Giebeler Citation1983, Esteves et al. Citation2006, Tjeerdsma Citation2006). shows the results of a sugar analysis before and after treating aspen with high-pressure steam at 220°C for 8 minutes, presented by Rowell et al. (Citation2009). The data show that at a very early stage of weight loss, the hemicellulose polymers (rhamnan, arabinan) are breaking down while the cellulose remains unchanged.
Table I. Sugar analysis of aspen before and after heat treatment with saturated steam at 220°C in a closed system for 8 minutes (Rowell et al. Citation2009).
In the heat treatment of wood, the chemical structure is transformed by autocatalytic reactions of the cell-wall constituents. It is known that during the heat treatment of wood under moist conditions, carbonic acids, mainly acetic acid, are initially formed as a result of cleavage of the acetyl groups particularly of hemicelluloses (Kollmann and Fengel Citation1965, Dietrichs et al. Citation1978, Bourgois and Guyonnet Citation1988). Depending on the acid concentration and on the temperature, hemicelluloses are hydrolysed into oligomeric and monomeric structures (Klauditz and Stegmann Citation1955, Bobleter and Binder Citation1980, Carrasco and Roy Citation1992). The weight loss from hardwoods is greater than that from softwoods, probably due to the greater content of acetyl groups in hardwoods which release acetic acid during heat treatment which contributes to the acid hydrolysis (MacLean Citation1951, Citation1953, Millett and Gerhards Citation1972, Hillis Citation1975). Subsequently, the monomeric sugar units are dehydrated to aldehydes, furfural being formed from pentoses and hydroxymethylfurfural from the dehydration of hexose sugar units (Burtscher et al. Citation1987, Kaar et al. Citation1991, Ellis and Paszner Citation1994).
Several other investigators like Burmester (Citation1973, Citation1975), Popper et al. (Citation2005), Boonstra et al. (Citation2007), Welzbacher et al. (Citation2007) and Pfriem et al. (Citation2010) have shown that the reduced hygroscopicity accompanies increased dimensional stability and increased durability. The modification also leads to colour changes (Bekhta and Niemz Citation2003) and the development of an intense odour (Peters et al. Citation2008).
In the heat treatment of wood above 150°C, the strength is reduced and the mode of failure of heat-treated wood in mechanical tests is in most cases brittle. The density of wood is decreased by 5–15% during heat treatment, and this of course affects the strength, but it does not explain the entire reduction in strength (see Welzbacher et al. Citation2007, Esteves et al. Citation2008a). The loss in strength is due to a degradation of the cell-wall matrix due to a degradation of the hemicellulose polymer. General guidelines for the reduction in strength are: hardness 5–10%, bending strength 10–20%, modulus of elasticity 5–20% and impact bending 30–80%. This means that heat-treated wood in its current form is not suitable for load-bearing uses (Jämsä and Viitaniemi Citation2001, Militz and Tjeerdsma Citation2001, Rapp and Sailer Citation2001, Vernois Citation2001).
Heat-treated wood may be suitable for outdoor applications above ground due to its greater durability and dimensional stability. Nevertheless, the brown colour of heat-treated wood fades quite quickly when the wood is outdoors and it eventually becomes grey (Jämsä et al. Citation2000), indicating photodegradation and a loss of lignin at the exposed wood surfaces. Heat treatment gives no or a very poor protection against blue stain. There are discrepancies in the literature as to whether or not heat treatment reduces the susceptibility of wood to surface cracking when it is exposed outdoors. Vernois (Citation2001) stated that ‘cracking due to dimensional movement is reduced in comparison with natural wood’. In contrast, Jämsä et al. (Citation2000) found that heat-treated pine and spruce surfaces cracked to the same extent as unmodified wood when exposed outdoors. However, Sandberg (Citation1999) and Sandberg and Söderström (Citation2006) have shown that the tendency of wood to crack during weathering is strongly related to the annual ring orientation in the cross section of the wood. To avoid cracks occurring on wood used outdoors, wood should be selected with the annual ring orientation perpendicular to the exposed wood surface. Differences in the annual ring orientation in the wood used for different studies of heat-treated wood are probably the main reason for the disagreement in the literature as to whether or not heat treatment reduces the tendency of wood to crack when exposed outdoors.
It is not recommended to use heat-treated wood in ground contact (Jämsä and Viitaniemi Citation1998, Kamdem et al. Citation1999, Wienhaus Citation1999, Rapp et al. Citation2000, Westin et al. Citation2004). Due to the low-equilibrium moisture content and partial degradation of the wood components, i.e. a large portion of the hemicelluloses are depolymerized, the susceptibility to biological degradation by brown-rot is reduced. Because of low-lignin degradation during heat treatment, there is a poor protection against white-rot that mainly attacks the lignin in wood.
Many artisans suggest that ageing improves the stability of wood and that aged wood has better acoustic properties than fresh wood. Many attempts have been made to clarify the effects of ageing on the wood properties. Natural aging of wood refers to the structural and chemical changes occurring in the wood over time when wood is subjected to unexceptional changes in the climate, UV irradiation, etc., and is not subjected to biodegradation. In general, the stiffness and strength of wood are enhanced by ageing for several hundreds of years, but the wood becomes more fragile and less ductile (Obataya Citation2007). On the other hand, little information is available regarding the acoustic properties of aged wood. All the results available indicate that the acoustic quality of wood is enhanced by ageing, and that such changes are qualitatively different from those due to chemical and thermal treatments.
The effect of natural ageing of wood at low temperatures, i.e. less than 50°, on the physical, mechanical and chemical degradation has been studied by Kohara (Citation1952, Citation1953), and more recently by Erhardt and Mecklenburg (Citation1995), Erhardt et al. (Citation1996), Obataya (Citation2007, Citation2009) and Froidevaux et al. (Citation2012). The results show no or small changes in the mechanical properties during ageing. However, Erhardt et al. (Citation1996) found some chemical changes during ageing. Ganne-Chédeville et al. (Citation2012a,Citationb) have used FTIR-ATR and UV resonance Raman (UVRR) spectroscopy to study chemical changes in naturally aged wood (200–500 years) and accelerated aged wood. They concluded that time alone does not affect the chemistry of wood during ageing. Additional factors such as UV-light, humidity, insects or fungal attack and the origin of the wood influence the changes.
Attempts have been made to reduce the negative effects of heat treatment at high temperatures, such reduced strength and odour, by treating wood at moderate temperatures, i.e. 100–150°C. The main task is to reach the required properties without too long a processing time. In general, most drying treatments, even at mild temperatures, decrease the hygroscopicity of wood, although this effect may be eliminated by saturation with water (Tiemann Citation1941a,Citationb,Citationc). Millett and Gerhards (Citation1972) tested six different species in air at temperatures of 115, 235, 155 and 175°C for periods from 1 to 255 days. They found decreases in weight, in moduli of rupture and in elasticity in bending with continuing exposure at each of the four temperature levels. As expected, the rate of loss of these properties was very sensitive to temperature. The experimental data were summarized in Arrhenius-type graphs to enable properties values to be estimated under other exposure conditions. Ganne-Chédeville et al. (Citation2012b) also found that artificial ageing at 160°C led to a significant change in the lignin structure, a well-known effect in the thermal treatment of wood, whereas treatment at 130°C did not alter the wood structure to any significant extent. Principal component analysis of the UVRR spectra confirmed that the spectrum of wood artificially aged up to 160°C is dissimilar to that of naturally aged wood and also dissimilar to that of unaged wood. Heat-treated wood is very similar to aged wood with respect to its fragile nature and improved stability. However, different effects of dry heating (oven heating) and steaming suggest that careful moisture conditioning is required for ‘true’ accelerated ageing (Obataya Citation2009). To clarify the differences between heat treatment and accelerated ageing it is important to show the effects of humidity on the viscoelastic properties of heat-treated and aged wood.
A pre-study at Linnaeus University in Sweden during 2010 showed that pine heat-treated at 105°C exhibited colour changes to the same degree as that processed at higher temperatures but no appreciable odour and strength reduction. The process time was very long (one year), and this probably affects the economic value of such a process. The result, however, indicates that it is possible to reduce the unwanted odour from heat-treated wood and thus to increase the potential for indoor use. Recently, a international company for interior design stopped the sale of traditional heat-treated wood for indoor use as a result of its odour.
Compression in the fibre direction of wood–wood bending
The concept of changing the shape of wet wood by heating and applying an axial compression force is old. The origins of the technique of wood bending have been the subject of some dispute among experts, but it is clear that wood bending was known in Egypt around 1000 B.C. (Rivers and Umney Citation2005) and probable already as early as 2800 B.C. (Aldred Citation1954). More immediate evidence that the process of bending wood was known to the Egyptians is provided by the paintings in the tomb of the Amenemhat at Beni Hasan, 1971–1928 B.C. It contains an illustration, , of a bowmaker who holds a rod over a receptacle that possibly contains hot water, while straight rods and completed bows are displayed nearby (Ostergard Citation1987).
Figure 1. Bending of bows from a facsimile of a wall painting in the tomb of the nomarch Amenemhat at Beni Hasan, ca. 1971–1928 B.C. A nomarch was the semi-feudal ruler of an Ancient Egyptian province (Newberry Citation1893)
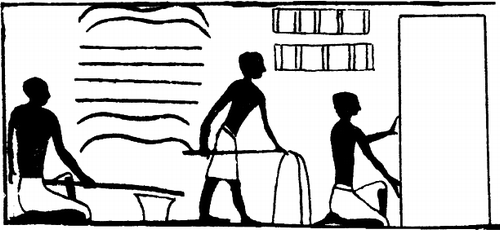
In about 1850, Michael Thonet (1796–1871) developed and applied techniques of bending beech wood treated by steam for furniture manufacture. His invention of the bentwood process and his design of the famous ‘Vienna No. 14 chair’ led his company to become the largest furniture manufacturer in the world. The technique was industrialized quickly in many countries and is still in general use.
The main reason for the difficulty in bending solid wood is the low strain to failure (about 1–2%) in tension. However, when wood is plasticized it becomes more plastic or semi-plastic, and this means that it can be softened and formed so that it keeps its shape after it cools. Wood contains areas of crystalline molecular structure that are difficult to plasticize and it is therefore also called semi-plastic. Its compressibility in the longitudinal direction is then greatly increased, as much as 30–40%, although its ability to elongate under tension is not appreciably affected, . After plasticization, compression parallel to the fibres and bending causes a combination of tensile and compression stresses in the bentwood. Because the compression strain to failure is many times larger than that in tension a curvature, even sharp, can be obtained. The ability of the plasticized wood to be compressed without damage is then being fully utilized. In practice, this means that one has to control the length of the pieces during bending with the help of some type of end stop (strap-and-stop) on the tensile side of the pieces being bent to prevent them from being elongated by more than 1–2%.
Figure 2. Stress-strain (σ–ϵ) diagrams for air-dried non-plasticized beech and plasticized beech. Note that the strain axis has different scales to the right and to the left of the origin (after Prodehl Citation1931a,Citationb). MC, moisture content
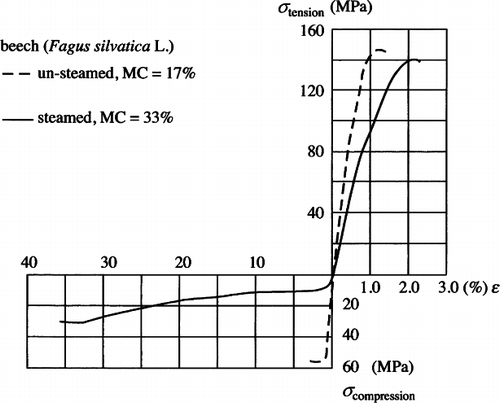
Hanemann (Citation1917, Citation1928) plasticized wood by heating it in low-pressure steam or in hot water, after which the wood was compressed along the fibre direction. In order to avoid bending during compression, the wood was set up in a fixture. The compressed wood was then bent in a cold state and set in the desired curved shape by heating to 80°C. The idea of compressing the wood in the longitudinal direction to improve the bending properties was further developed in the 1930s under the name Patentbiegholz (Kollmann Citation1936). No industrial application came until the 1990s (Morsing Citation2000).
In general, the application of a compressive load in the longitudinal direction of softened wood may lead to the following:
a. | plastic deformation of the cell wall with the formation of slip planes; | ||||
b. | cell wall buckling; | ||||
c. | localised shear-band deformation (at the macro-level); and | ||||
d. | diffuse shear-band deformation (homogeneous). |
These deformations are shown diagrammatically in .
Figure 3. Various modes of deformation of the cell walls of wood under compression in the longitudinal direction; (a) plastic deformation of the cell wall (formation of slip planes), (b) lateral buckling of the cell walls, (c) localised shearing band, (d) diffuse buckling of the cell walls
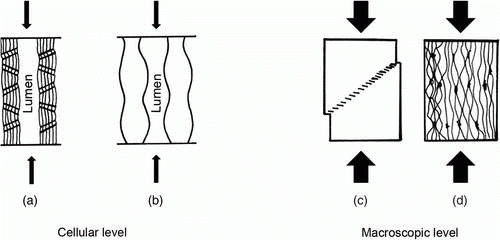
Each type of deformation that is developed is also dependent on the density of the wood. The deformation at the macro level may be explained by various physical mechanisms taking part at the cellular level. Many researchers have observed this behaviour in different kinds of wood. In wood of low density (<300 kg/m3), Easterling et al. (Citation1982) showed that the cause of the local large deformation is the collapse of fibres by rupture at the ends of the cells. This mechanism is shown schematically in b. Kučera and Bariska (Citation1982) have shown that in wood of higher density the local large deformation of wood can be due to a local Euler type buckling in the walls of the cells, illustrated in c, which generally leads to the formation of a shear band as shown in c on the wood macro-level.
Compression in the transverse direction of wood–wood densification
Compressing wood in the transverse direction reduces the void volume of the lumens in the wood material and increases the wood density. This process is commonly called densification, i.e. the untreated wood has been compressed under conditions that do not cause flow of the lignin. Initially, the main aim of wood densification was to improve the mechanical behaviour of wood by eliminating its porosity (Kollmann Citation1955). It is well known that many of the properties of solid wood correlate with its density and can thus be enhanced by increasing the density. Vorreiter (Citation1942) reported that in 1886 the idea of densification of massive wood by applying a compression force was patented by Robert Stöckhart. In the USA, Sears (Citation1900) patented a method for densifying wood for use as matrix-block for character-impressions. Around 1915, the Pfleumer brothers in Austria incidentally found a method for the densification of wood through impregnation with rubber. They developed the idea to an industrialized process for wood densification (Pfleumer and Pfleumer Citation1915, Pfleumer Citation1922). This type of densified wood was used in the aviation industry until 1945, after which it was replaced by aluminium. During the 1920s and 1930s several patents were issued for compressed wood products, e.g. Walsh and Watts (Citation1923), Ollesheimer (Citation1929, Citation1930), Brossman (Citation1931), Olsen (Citation1934) and Esselen (Citation1934).
There are two principal ways of achieving densification in the transverse direction; densification of the whole volume of wood (uniform densification or bulk compression) or densification of a surface layer of the wood (surface densification). The uniform densification process is used during the shaping of wood. One negative effect of compressing the whole wood is, however, the loss in volume. To circumvent this problem, an alternative approach is to laminate bulk compressed wood onto uncompressed wood or other material using an adhesive (Kifetew and Wiklund Citation1999, Kutnar et al. Citation2008, Nilsson et al. Citation2011). The purpose of surface densification is to increase the abrasion resistance and hardness of a wood surface, by compressing only the surfaces of solid wood to a depth of 1–5 mm or only a few cell layers. For decades, many attempts have been made to invent new techniques for producing a form-stable densified wood surface. The main motivation of this work is to utilize low-density woods for flooring where the wood surfaces are exposed to excessive wear. shows micrographs of uniform densification and of a densified surface. Rautkari (Citation2012) has investigated three different methods of surface densification; a lamination technique, where low-density wood was laminated to a high-density compressed wood surface, surface modification where densification was accomplished using a single-sided heated press and with a single-sided heated press assisted by frictional heating. With the lamination technique, it proved easy to generate the desired hard surface, but an adhesive is needed. The difference between compression with and without friction is that when friction is used the process temperature is rather difficult to control, but an even layer of extractives is formed on the surface. Without friction, however, extractive spots are seen on the surface.
Figure 5. Micrograph of uniformly densified spruce latewood cells after compression at 140°C under saturated moisture conditions (Navi and Heger Citation2005). Micrograph of the boundary between the compressed surface and uncompressed regions of wood (Inoue et al. Citation1990)
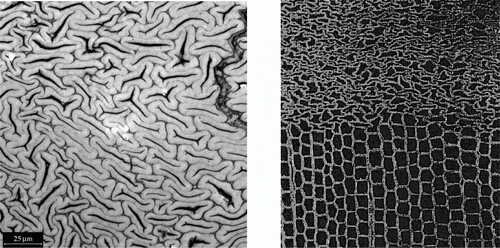
Wood can be densified without a pre-treatment or softening. However, to avoid damage to the wood (buckling of latewood annual rings) it is then very important that the compression is in the radial direction of the wood. Nilsson et al. (Citation2011) have presented a simple solid wood densification technique based on compressing a solid piece of softwood with vertical annual rings and without knots in the radial direction by restraining the tangential expansion. They also suggested a method to mechanically hinder the compression-set recovery. To avoid macro-cracking of untreated wood during compression, semi-isostatic densification has been tested with success (Lindhe Citation1993, Sandberg Citation1998, Blomberg Citation2006). In this method, however, the compression deformation is mainly in the radial direction, which results in a deformed shape of the specimen if the annual rings in the cross section are not parallel to the sides of the specimen. In most of the transverse densification methods presented in the literature, the wood has been softened by steam or chemicals before densification.
During the densification process, wood is generally heated with high-temperature steam at 110°C, under saturated conditions to reach a softened state. After the wood has been plasticized, it is compressed in the transverse direction and undergoes elastic-visco-plastic deformation, before it is cooled and dried. When the compressed sample is moistened and heated in the absence of any external force, it generally returns to its original shape, to a degree depending on the process parameters, i.e. temperature, moisture content and time, during compression of the wood. The thermo-hydro-plasticity of wood under these conditions of densification is thus only apparent. The phenomenon is known as ‘shape memory’ or ‘compression-set recovery’. The origin of the shape memory is to be found at the level of the cell-wall ultrastructure and molecular structure and an explanation of the phenomenon has been formulated by Norimoto et al. (Citation1993).
There are in principle several ways to prevent the compression-set recovery of densified wood:
• | by hindering the compression-set recovery by mechanically fixing the movements of the wood; | ||||||||||||||||
• | by preventing the compressed wood from being re-softened by avoiding re-humidification of the cell walls and thus making the cell wall inaccessible to water; | ||||||||||||||||
• | by forming covalent cross-links between the wood components in the deformed state by chemical modification; or | ||||||||||||||||
• | by releasing the elastic stresses and strains stored in the microfibrils and matrix during the compression through:
|
illustrates the four stages in the densification of wood at the cellular level of compression in the radial direction and fixation of the shape memory.
Figure 6. Schematic illustration of wood densification in the transverse direction at the cell level, recovery and different methods of fixation of the compression set: (a) initial undeformed state, (b) softening of the wood by steam, (c) densification, (d) cooling, (e) total set recovery, (f) mechanical fixation, (g) chemical fixation or fixation by a THM treatment
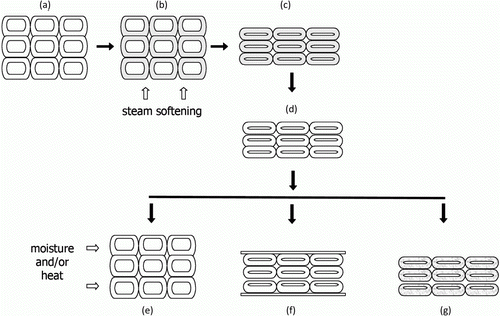
After the early work on wood densification in Europe, much of the research in wood modification, especially within the area of adhesive impregnation, was performed by Alfred Stamm and co-workers at the Forest Products Laboratory in Madison. To overcome the problem of the recovery from compression, Stamm and Seborg (Citation1941) impregnated thin veneers (in the green or dried state) with an aqueous solution of a phenol–formaldehyde pre-polymer (polymer precursor) whose molecules were small enough to penetrate the cell wall together with the water. The pre-polymer swells the cell wall up to 25% more than the swelling in water. After curing, the composite has a final volume roughly equal that of the water-swollen wood. The veneer is dried, but the pre-polymer is not polymerized, and the desired thickness can then be assembled from layers of veneer. Under heat and pressure, the impregnant is polymerized to yield a cohesive composite with an anti-shrink efficiency of 70–75%. Stamm and Seborg called this product Impreg.
Instead of merely gluing the impregnated veneers together, the stack of veneers can be placed in a press with heated platens and, as the composite material is heated, a pressure of up to about 7 MPa can be applied to compress the wood and collapse the cell structure. Heating at about 150°C for 15 to 30 minutes per 25 mm of thickness polymerizes the adhesive. The result was a high-density (up to 1460 kg/m3) product with very low-volume swelling (<4%) when soaked in water. This material has been given the generic name of Compreg. Compreg is an adhesive-treated and compressed wood which is stabilized in its densified form by the presence of an adhesive. Haygreen and Daniels (Citation1969) developed a method of densifying and drying green sapwood. When the product was soaked in water for three weeks, the recovery of the product was found to be between 10 and 15%. To reduce the water absorption, they impregnated the green wood with phenol–formaldehyde. The recovery of the resin-treated wood was then only 1–2%. In the same way, Gabrielli and Kamke (Citation2008) report the greater shape stability of phenol–formaldehyde treated and also acetylated densified wood. In this case, the wood was densified by the viscoelastic thermal compression process (Kamke and Sizemore Citation2005, Kamke Citation2006). Westin et al. (Citation2009) have tested Compreg-type products impregnated by furfurylation or waterborne furan during hot-pressing. The products had hardness higher than any known wood species, high-dimensional stability and decay resistance.
Seborg and Stamm (Citation1941) also succeeded in partially eliminating the shape memory of densified wood by thermo-hydrous treatments. Thin plates of hickory (12 mm thick and 106 mm in diameter) were densified at different moisture contents (6, 12, 20 and 26%) and at temperatures up to 170°C for 20 minutes. Seborg and Stamm showed that after densification at 170°C, the compression-set recovery was about 10%. An essential part of this process was the need to press the wood at a sufficiently high temperature and moisture content to ensure that the lignin flowed during processing, to relieve internal stresses during compression. Later, they improved the process and further decreased the recovery, and they called this product Staypak (Seborg et al. Citation1945, Stamm et al. Citation1948). Staypak is heat-stabilized, compressed wood that has been heated, during the pressing process under conditions such that the compression is not lost when the wood is subsequently swollen. Although it can swell appreciably, it returns to practically the original compressed thickness on drying to the original moisture content. It is thus wood that ‘stays compressed’ (Seborg and Stamm Citation1941). The advantages of Staypak over Compreg lie, however, in its strength characteristics. Although the shear strength parallel to the grain and in a plane perpendicular to the direction of compression of the wood is appreciably lower for Staypak than for Compreg, the impact strength is about double, and the tensile and bending strengths are approximately 25% higher than those of Compreg.
To obtain a stable densified wood (i.e. a densified wood with no shape memory), a post-treatment process involving a THM treatment in two steps can also be used. The transverse densification of the wood (first step) is followed by a second post-treatment step to eliminate the shape memory. The fixation of the compressive deformation of densified wood has been investigated using saturated steam (Tanahashi Citation1990, Inoue et al. Citation1993, Dwianto et al. Citation1997, Ito et al. Citation1998a,Citationb, Navi et al. Citation2000, Navi and Heger Citation2005), by dry heating (Dwianto et al. Citation1997) and also under non-saturated conditions by Heger (Citation2004). These investigations have shown that higher temperature and moisture content greatly reduce the post-treatment time necessary to eliminate the shape memory. For instance, it is necessary to keep the samples in a dryer for two weeks at a temperature of 40°C, for 30 hours at 140°C or for 12 hours at 180°C (see Navi and Sandberg Citation2012). A THM post-treatment of thin densified wood specimens at 200°C with saturated steam for 4 minutes is sufficient to eliminate the shape memory. The hydrolysis of hemicelluloses during THM post-treatment plays an important role in the elimination of shape memory through the dissipation of the stresses stored in the microfibrils and matrix. It should, nevertheless, be noted that to achieve a total elimination of the shape memory, it is necessary for the lignin to be in its rubbery state during the THM treatment.
Numerical simulation of the wood-moulding process is complex and is currently at an early stage of development. Nairn (Citation2006) has carried out an extensive numerical modelling on both softwood and hardwood in transverse compression with good results. He used the material point method which can handle large deformations, can model elastic-plastic cell-wall properties, and automatically takes into account contact between cell walls. It is also easy to discretize micrographs of wood specimens into a numerical model. Ormarsson and Sandberg (Citation2007) have successfully studied deformations in the laminated bending of veneers using finite element analysis.
Wood welding
Wood welding is a mechanical friction process allowing two pieces of wood to be assembled by fusion of the surfaces in contact. The time required for the welding process is short, taking less than a few minutes. The mechanical performance of the pieces joined by friction welding can be as good as that achieved by gluing with conventional adhesives, but it is highly dependent on the process parameters and on the wood species. Furthermore, the welded joint is sensitive to moisture, i.e. there are problems with the water resistance of the joint.
Bevington (Citation1891) invented a rotary welding process to form tubes and to weld the ends of two tubes together. The first connections with plastics were made in the 1940s by Henning (Citation1942). In the 1950s, the friction welding process was also developed in the Soviet Union and Czechoslovakia and, using different techniques, friction welding was developed and applied to numerous materials (Vill and Shternin Citation1957, Vill Citation1959). With these techniques, it is even possible to join different materials, e.g. steel and aluminium. Not until the 1990s were the first attempts made to join wood by means of friction (Suthoff et al. Citation1996, Suthoff and Kutzer Citation1997). The first work on the frictional welding of wood was documented and the process patented by Suthoff et al. (Citation1996) in Germany. This patent and a subsequent one (Suthoff and Kutzer Citation1997) have reported that pieces of wood can be joined together through an oscillatory frictional movement. It has also been suggested that it may be possible to join pieces of timber with a wood dowel using rotary frictional welding. This patent also includes the welding of wood under an inert atmosphere or vacuum. The heat generated during the welding process depends on various parameters such as the welding machine setting (frequency, amplitude, welding pressure) and the wood properties (wood species, surface conditions, moisture content, etc.). These parameters have an important influence on the heat generation rate and consequently on the processing time and on the bond quality. Four different types of oscillatory friction movement are usually used for the welding, as shown in .
Figure 7. Movements, velocities and forces in four different types of frictional welding; (a) linear friction, (b) orbital friction, (c) circular friction and (d) rotary friction welding (Stamm Citation2005)
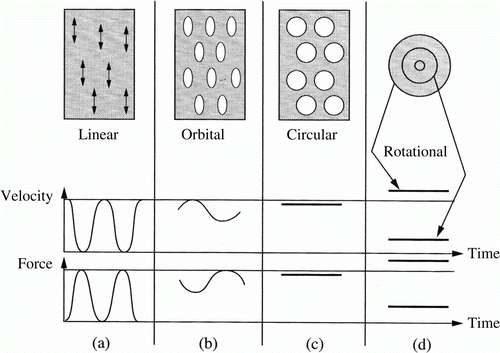
Linear welding is characterized by a linear oscillatory movement. The movement stops and changes direction when reaching the maximum amplitude of the oscillation. In this movement, the velocity and its corresponding frictional force (parallel to the surface) are sinusoidal, as shown in a. The frictional movements in orbital and circular welding are similar. In orbital friction welding, the movement is elliptical so that the force and velocity are sinusoidal, as shown in b. In circular welding, the movement is circular and the velocity and frictional force are constant during the processing, as shown in c. In rotary friction welding, the connection between the pieces is made by dowels and the method is usually called ‘dowel rotary friction welding’, as shown in d. In this method, a cylindrical (or conical) dowel is pressed into predrilled holes while rotating around its axis.
In 2002, the first attempt to weld wood by a linear vibration movement was carried out in Switzerland. Some of the research results are given by Gfeller et al. (Citation2003), Pizzi et al. (Citation2004), Leban et al. (Citation2004, Citation2005), Ganne-Chédeville et al. (Citation2005, Citation2006a,Citationb, Citation2007, Citation2008a,Citationb,Citationc), Stamm (Citation2005), Stamm et al. (Citation2005a,Citationb,Citationc), Boonstra et al. (Citation2006), Stamm et al. (Citation2006). Most of the wood welded by friction is Norway spruce (Picea abies (L.) Karst.) and beech (Fagus sylvatica L.), and the work has mostly been carried out on small samples with dimensions of about 150×20×15 mm, but specimens as large as 1600×40×20 mm have also been welded.
Rotary friction welding was first developed by Pizzi et al. (Citation2004) to join two wood pieces by wooden dowels, and it was shown that the method leads to an effective ligno-bonding between the wooden dowel and the predrilled wood. shows a photograph of cross-sections of a dowel rotary weld.
Figure 8. Cross-sectional profile of a dowel ligno-bonded by rotary friction welding (Ganne-Chédeville et al. Citation2005)
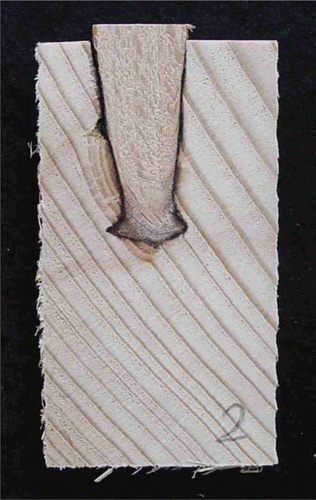
shows interphase of a welded joint of two spruce pieces achieved by circular friction, observed in a confocal microscope. This figure shows that the connected interfacial zone consists of two parts: a middle part of a dense amorphous material ‘fused’ with fragments of densified wood fibres and melted material and a second part of densified fibres surrounding the middle part. The densification of the second part gradually decreases until the un-deformed wood fibres are reached. The total thickness of the middle and second zones is less than 1 mm.
Figure 9. Micrograph of a zone of joined and adjacent areas between two pieces of spruce welded by circular friction (Stamm et al. Citation2005a)
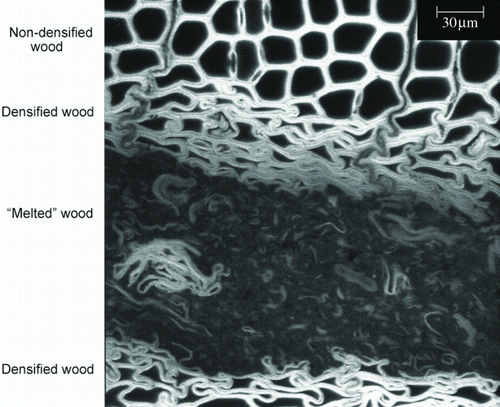
The chemical and physical changes induced by the welding process have been intensively investigated. The thermal energy generated by friction is used to soften the wood constituents, mainly lignin and hemicelluloses. This leads to the detachment of wood cells and the formation of an entangled network of fibres immersed in a matrix of molten material which then solidifies and yields significant adhesion in the interphase of the weld. To understand the thermal reactions, thermal decomposition and alteration of the wood constituents during the welding process, between 140 and 440°C, Stamm et al. (Citation2005c, Citation2006) have studied the formation of the ‘melted’ material by chemical and spectroscopic methods. Their results have shown that the hemicelluloses were degraded to a great extent while the cellulose remained relatively stable. The lignin also underwent distinct changes, as demonstrated by an increase in the number of free phenolic groups and a decrease in the typical bonds between the phenol propane units. They also detected furan derivatives within the volatiles of the smoke gas, arising mainly from hemicelluloses. They concluded that reactions between furfural and other furan derivatives with lignin are the main reactions in the friction zone leading to cross-linking of the ‘melted’ contact material. This material has also been examined by Gfeller et al. (Citation2004), Ganne-Chédeville et al. (Citation2005) and Kanazawa et al. (Citation2005) and it has been shown that the essential chemical modifications involving the polymeric constituents of wood in friction welding occur in the first five to six seconds and that they slow down or even stop afterwards. Analysis by FTIR and CP-MAS 13C NMR of the welded area of wood has shown dehydration and an apparent increase in the crystallinity of the cellulose. Certain hemicellulose degradation has occurred, accompanied by the generation of furfural. Cellulose degradation is very slight. Both analytical techniques show an increase in the proportion of lignin in the welded interphase. Self-condensation of lignin occurs by internal rearrangement, and this continues throughout the welding process. After six seconds of welding, the wood begins to carbonize, and an increase in cross-linking of the lignin network is observed. Ganne-Chédeville et al. (Citation2005) have suggested that to produce the frictional energy necessary for the reaction between furfural and other furan derivatives with lignin and to achive good adhesion between the two wood pieces, the variation in temperature at the interface has to be monitored (Ganne-Chédeville et al. Citation2006a,Citationb). During the welding process, the interface of the wood heats up and creates a ‘melted’ material. After the frictional movement has stopped and during the cooling phase, the ‘melted’ layer solidifies forming a continuous joint between two wood pieces. Pizzi (Citation2012) has suggested that the mechanism of mechanically induced wood vibration welding is due mainly to the melting and flowing of wood intercellular polymer material. This causes the ‘ungluing’ of long wood fibres, and the formation of an entangled network of fibres in the matrix of solidified molten cell-interconnecting material. Thus, a high-density, high-strength wood cell/fibre entanglement network composite with a molten lignin polymer matrix is formed.
Recently, considerable work has been carried out to investigate the potential of wood joining by different frictional techniques. The mechanical performance, particularly the resistance to fracture propagation and the influence of water on fracture toughness has been examined by various tests developed for adhesive joints. Joining by frictional methods still involves certain inconveniences, which need to be overcome before they can be applied in timber construction. Water absorption by friction-welded wood joints lowers the strength of the joints. This is one of the greatest weaknesses of wood welding and its use for constructional purposes. However, Pizzi (Citation2012) has shown that dowel welding can be used for protected exterior joints due to the combination of rosin waterproofing and joint geometry. Welded dowel joints hold together for longer than 500 days on immersion in water. Vaziri (Citation2011) studied the water resistance of welded Scots pine (Pinus sylvestris L.) and found that the water resistance was greater when heartwood was used, due to the higher content of extractives in heartwood. The water resistance was not, however, sufficient to classify the joint as an unprotected exterior grade. In an attempt to explain the behaviour of a welded joint to water, Rhême et al. (Citation2011, Citation2012) have investigated the characteristics of mode I and mode II fracture propagation at various moisture contents. They have shown that the critical energy release rate in both mode I and mode II is a function of the moisture content of the specimen. shows a multi-layer wooden element consisting of eight 6 mm thick lamellae of alternating spruce and beech manufactured by circular friction welding . This welded wooden element could be used instead of a laminated wooden beam or a wooden wall element made of glued boards (Stamm Citation2005).
Figure 10. A wooden multi-layer element made of alternate layers of spruce and beech bonded by circular friction welding (Stamm Citation2005)
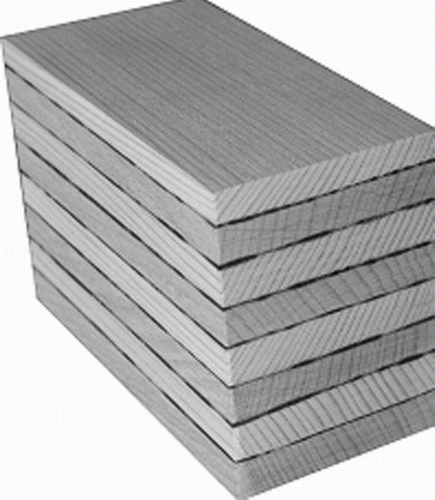
Recently, more studies have concentrated on improving the strength of wood joints by optimizing the welding machine parameters (frequency, amplitude and welding displacement or welding process time), to solve up-scaling problems and improve the quality of large welded joints for application in timber construction. An attempt to use linear friction welding to produce an adhesive-free strand-board of spruce has been described by Bianchi et al. (Citation2012). The method represents an interesting alternative to standard adhesive-bonded hot-pressing technology, but is affected by limitations such as the large amount of volatiles derived from the thermal degradation of wood, and that the maximum reachable mechanical properties are lower than those of the existing standards.
The phenomena that arise on the micro-level of wood during friction welding have an interesting relation to the rather unexplored area of laser treatment of wood. Laser treatment, depending on irradiation parameters, is a form of heat treatment which is very concentrated in space and time and exhibits interesting effects such as a molten surface. This type of molten surface was observed by Sandberg (Citation1999) and Johansson and Sandberg (Citation2007) during preparation of weathered samples of pine and spruce with the help of UV-laser ablation (Seltman Citation1995a,Citationb). Haller et al. (Citation2001) have investigated the effect of laser irradiation on wood with respect to changes in structural, chemical and physical properties, and they have identified the laser parameters which guarantee melting of wood without pyrolysis.
Industrialization of TH/THM concepts
Several TH/THM processes have been industrialized during the last 150 years. Examples are the bending of solid wood, i.e. the Thonet process, laminated bending, softening of logs or sawn wood before veneer slicing, kiln drying of sawn wood and various concepts in board manufacture. Today, there are still advances in these concepts, e.g. to increase productivity or for developing more environment-friendly processes. The following is a presentation of some TH/THM concepts where great progress has been made during recent years and where that progress has in some cases resulted in new or improved industrialized processes.
Heat treatment
The heat treatment of wood has been investigated for many years without any break-through in commercialization. The general discussion about the use of tropical hardwood, the toxicity of wood preservatives, and especially the willingness of sawmills and the wood industry to broaden their product range has led to the industrial development of several heat-treatment processes since the early 2000s.
On the European market, several industrial heat-treatment processes have been introduced. The most common processes are: the Thermowood process, the Plato process, the retification process, le Bois Perdure, the oil-heat treatment (OHT) process. The total capacity of heat-treated wood in Europe is about 200,000 m3/year and Finland alone has a production of 100,000 m3/year. The heat-treatment plants have a capacity between 1000 and 30,000 m3/year. Several different species are used in the process, but spruce is dominant.
Industrial heat-treatment processes typically aim at improving the biological durability of less-durable wood species and at enhancing the dimensional stability of wood or wood-based products, e.g. particle boards. The properties of industrially produced heat-treated wood in general have been intensively investigated by Viitaniemi and Jämsä (Citation1996), Leithoff and Peek (Citation1998), Sailer et al. (Citation2000), Jämsä and Viitaniemi (Citation2001), Rapp and Sailer (Citation2001), Boonstra and Tjeerdsma (Citation2006), and for OSB-strands particularly, Del Menezzi and Tomaselli (Citation2006) and Paul et al. (Citation2006, Citation2007).
The basic difference between these different processes is in their choice of oxygen-excluding and heat-transporting media. The Thermowood process uses steam, and in the Plato process the wood is heated to 150–180°C in an aqueous environment at superatmospheric pressure (including saturated steam as the heating medium) in a first step followed by a drying and heating once again to 150–190°C, but under dry and atmospheric conditions; and finally a conditioning phase. The retification process uses nitrogen and the OHT process use vegetable oils, e.g. rape seed oil, linseed oil or sunflower oil (Rapp and Sailer Citation2001, Rapp et al. Citation2006). However, the treatment conditions are very similar in these processes which operate between 160 and 240°C to modify the chemical composition of the cell wall. Modified heat-treatment processes are also emerging in Denmark, the Wood Treatment Technology (WTT) process working at 160–180°C, and in Austria, the Huber Holz process working at 170–230°C. Both processes have heated steam as medium, but the WTT process can also add oil in the process. Only a few processes, like Plato, have used relatively mild heat treatment to improve dimensional stability and at the same time avoid a deterioration in the mechanical properties of the heat-treated wood. These different heat-treatment processes are covered in detail in Navi and Sandberg (Citation2012).
Advances in wood bending
The industrial production of bent wood for furniture production had its peak in produced volume around 1900. Even then, machinery for bending could make a large number of bends in one operation, and production rates were considerably increased. The development of machines for higher productivity has proceeded throughout the twentieth century. However, these machines are seldom used for the production of multiple (bi-planar) bends.
There has been considerable interest in finding ways to shorten the long processing times for heating (plasticizing) the wood before bending and drying it after bending. Dielectric heating, i.e. radio-wave heating (or high-frequency heating) and micro-wave heating technologies are the most common techniques developed for this purpose. Plasticizing wood with through dielectric heating has been studied for several years by Norimoto (Citation1979), Norimoto et al. (Citation1980), Mori et al. (Citation1984), Norimoto and Hasegawa (Citation1984), Norimoto and Gril (Citation1989) and Eggert (Citation1995). The literature indicates that there have been problems with uneven heating, but with today's knowledge of dielectric methods it should be fully possible to achieve a uniform heating. In Navi and Sandberg (Citation2012), an automatic process for plasticizing, bending and drying solid wood is presented in detail. This technique is now being introduced in industrial applications for in plane bending.
Being able to bend wood in a cold state is especially desirable in small product series and in workshops where it is not possible to provide equipment for conventional bending. The Compwood AS company in Denmark, emanating from the work of Morsing (Citation2000), has developed a machine for the pre-compression of wood in the longitudinal direction to make it bendable in a cold state, as shown in . The procedure consists of three distinct stages: (1) the steamed, softened wood element is longitudinally compressed by about 20%, (2) the applied pressure is then released, after which the wood partially recovers from the compression-set so that the residual deformation is 4–5% and (3) the wood is then allowed to cool at room temperature and dry to a moisture content of 12%. The wood piece can then be polished and processed to the desired cross-sectional shape and surface-finished as a straight piece of material. The advantage of this process is that the wood element can be subsequently bent in a cold state without the application of steaming or jigs. The smallest radius of curvature for 25 mm beech is ca. 230 mm, which may be compared with the value of 330 mm for plasticized beech without any restraining band.
Figure 11. Compwood machine for pre-compression of wood in the longitudinal direction to make it bendable in a cold state (view from above). The operations are: (a) loading, (b) autoclave for plasticizing the wood, (c) automatic input, (d) machine for longitudinal compression, and (e) output of densified wood
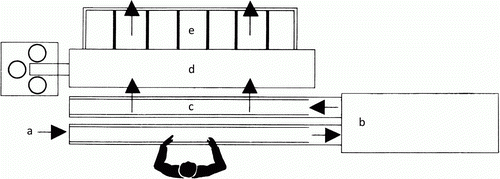
The bendability of the wood can be further increased if, after having been pre-compressed and dried until item 3 in the above list, the material is subjected to a further compression of 20% in the cold state. After the pressure has been released, there is a residual deformation of about 15%. A piece of beech which has been processed in this way can be bent in the cold state to a radius of curvature as little as 100 mm.
Transverse densification or moulding
Densified wood has been produced in Germany since the early 1930s and marketed under the trade name of Lignostone, and a corresponding laminated compressed wood was produced under the trade name of Lignofol. A resin-treated laminated compressed wood under the name of Kunstharzschichtholz has also been in commercial production (Kollmann Citation1936, Stamm Citation1964). Lignostone is still being manufactured in Germany by the Röchling-Gruppe. The compressed and resin-impregnated wood Compreg presented by Stamm and Seborg (Citation1941) is today being produced on an industrial scale in Germany and Poland. Compreg plywood with a density of 1250 kg/m3 can also be found on the market.
The primary goal of recent research on existing wood densification techniques and of the development of new techniques in THM post-densification treatments is to extend them to wooden elements of larger dimensions than was previously considered feasible. Today, in Japan various investigations into the THM moulding of wood are going on. In summary, the processes investigated are commonly based on the two-directional transverse densification of wood elements which employs in four distinct stages: (1) wood plasticization by high-temperature steam, (2) compressive moulding (two-directional shaping), (3) THM post-compression-treatment and (4) cooling. Ito et al. (Citation1998a,Citationb) have developed a system to transform small logs (maximum length and diameter of 340 and 200 mm, respectively) into beams with a square section by two-directional transverse densification, as shown in .
Figure 12. Thermo-hydro-mechanical apparatus and the internal moulding block (left) and transformation of a circular trunk to a square-section block by two-dimensional densification (right) (Ito et al. Citation1998a)
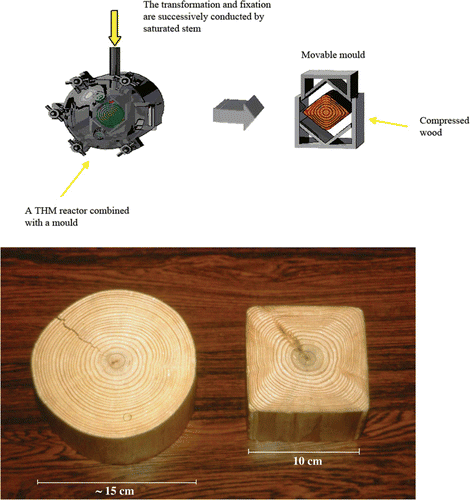
Due to the decrease in availability of the Japanese high-strength hardwoods, Dwianto et al. (Citation1997, Citation1998) have attempted to improve the properties of the more readily available low-density softwood Sugi for flooring and interior wall materials. The wood was compressed to 50% of its original thickness in the radial direction in a wet condition. It was then restrained and dried under heat and an almost complete fixation was achieved with about 4% weight loss of the heat-treated and compressed wood. The most important differences between these two processes reside in the post-treatment stage. In the first technique, the operations of moulding and post-densification are overlapping, whereas in the second technique these two stages occur separately. The problems related to the dimension of the wood elements (scale-related effects) such as the development of cracks and exfoliation of wood during shaping, cooling and drying have apparently not yet been solved. Shigematsu et al. (Citation1998), Kyomori et al (Citation2000) and Tanahashi et al. (Citation2000) estimate that more fundamental knowledge on wood THM behaviour is required before the issues that currently stand in the way of developing a new manufacturing system for ‘compressive moulding of wood under high-pressure steam techniques’ can be overcome. Recently, the Olympus Corporation presented wood products, such as the outer casings of electronic products, manufactured in a three-dimensional compression moulding process for wooden materials (Tatsuya Citation2007). The processed wood has the feel and grain pattern of natural wood, but is much harder than engineering plastics such as acrylonitrile–butadiene–styrene (ABS) and polycarbonate. The material is strong enough to be used to create the outer casings of electronic products, and it can enhance the aesthetic value and pleasure of ownership that these products provide.
In the USA, commercial interest in the harvesting of rapidly grown wood with an innate low density has driven the wood-research community to investigate possible opportunities of employing densified wood products in the structure of composite materials (Kamke Citation2004, Kutnar et al. Citation2009). To densify small low-density hybrid poplar specimens, Kamke and Sizemore (Citation2005) have developed a semi-closed THM reactor. The process of densification and shape-recovery elimination consists of four stages: (1) softening of the wood element through hot-steaming and densification, (2) transient release of steam pressure and mechanical compression for about 100 seconds, so that the specimen partially loses its temperature and moisture content, (3) reapplication of the compression load and increasing the temperature from 175 to 200°C and (4) cooling the specimen to 100°C while still under compression. This semi-closed system might have had some potential advantages over the closed systems developed in Japan and Switzerland, but unfortunately the dimensions of the specimens used for densification were very small. Scaling issues render it difficult to evaluate the interest of this system for wood elements of the size envisaged in building constructions without practical research on elements of a comparable size.
New ideas on tubes and column constructions
Next to material properties, the structural and economic performance of the cross section of a load-bearing structural component is the most important design issue. With the aim of providing engineered wood products for structural applications, tubes or columns of densified wood have been developed at the Institute of Steel and Timber Construction at the Technische Universität Dresden, Germany (Haller et al. Citation2004). The tube has a much larger load-bearing capacity than solid wood. The formed tubes can be optionally reinforced with technical fibres and/or textiles laminated to the outer wood surface, as shown in . The purpose of such a composite confinement is to strengthen the relatively thin-walled sections and to protect the wood against environmentally induced damage. Besides structural applications in civil engineering, the tubes can also be used as water pipes (Putzger et al. Citation2012).
The columns are made from densified glued laminated timber boards of spruce and rolled to a wooden tube (Haller Citation2007). shows the different steps in the manufacture of a tube from sawn wood and from wood with a more or less circular cross section.
Sawn boards at a moisture content of about 12% are softened and densified in the transverse direction in an open system at 130–160°C for about 2 hours. After cooling, the densified boards are glued to a panel with a length which corresponds to the final length of the tube. The boards in the panel can be oriented at an angle α to the longitudinal direction of the tube to achieve a tube with a spiral grain according to the tree stem, which is expected to give a higher load-bearing capacity, although this has not yet been confirmed experimentally. Today, an angle α equal to 0° is used. The panel is heated to a temperature of 80°C and the panel is formed in a mould, as shown in .
Figure 15. Moulding of a densified wood panel to a tube of diameter 450 mm; (a) charging, (b) closing, (c) forming. The thickness of the tube wall is 100 mm
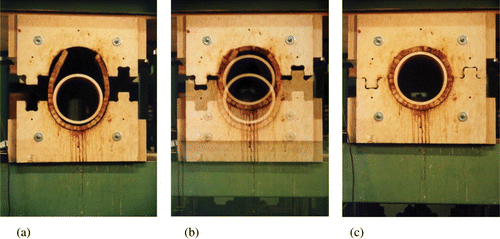
When the process begins with a log, the log is densified in transverse directions to yield a more or less rectangular cross-section. The pith is then sawn away and the pieces are glued together to a panel that has wood with the lowest degree of compression on one side of the panel and wood with the highest degree of compression on the other side of the panel. When the panel is shaped to a tube, the high-density side will be on the outer surface of the tube.
The maximum possible bending radius for the moulding operation is primarily dependent upon the degree of compression and upon the thickness of the panel.
Haller and Wehsener (Citation2004) and Welzbacher et al. (Citation2008) have tested heat treatment (the OHT process) for eliminating the compression-set recovery of large, industrial-scale densified woods. This study showed that oil heated at a temperature above 200°C can completely eliminate compression-set recovery of large densified spruce panels. The resulting product has also shown greater resistance to micro-organism degradation. Because of the greater durability than existing commercial products and the impregnation of densified wood by oil during post-treatment, this type of product is predicted to have potential for outdoor service.
Challenges for the TH and THM processing techniques
Various types of TH/THM processing technique can be utilised to enhance wood properties, to produce eco-friendly new materials and to develop new products. However, altering the way in which wood is treated is known to dramatically alter the way it performs. As an example, an unsuitable high-temperature TH/THM treatment can chemically degrade wood, and lead to structural and mechanical damage, loss of strength and greater brittleness. Another inconvenient feature of high-temperature-heated wood is its rapid ageing. Heat-treatment methods are knowledge-based processes. The modification of mechanical properties, dimensional stability, resistance to micro-organisms, change of the colour and odour are functions of the process parameters and consequences of wood chemical degradation. Any investigation of the combined effects of temperature, humidity, oxygen and time on wood chemical degradation and the corresponding modification of wood properties requires a multidisciplinary approach and sophisticated chemical and mechanical analyses and studies of resistance to micro-organisms. The changes in wood properties have their origin in the chemical reactions of the wood cell constituents produced during processing. There is a need to better understand the chemical degradation of wood to optimize the processing conditions and thereby ensure that treatments meet product needs. The environmental impact of the TH and THM processes is also an area that is not well explored. A life cycle and system perspective study of the different TH and THM processing techniques is necessary so that all the life cycle phases i.e. production, operation, retrofitting and end-of-life are considered and optimized as a whole, including the energy and material chains from natural resources to final services. Establishing a greater understanding through knowledge exchange will promote the effective processing of wood. This is particularly the case in transferring knowledge and experience from the research and development sector to the industrial sector and vice versa.
Heat treatment, densification and wood welding by friction have been in focus in this state-of-the-art review. These processes are at various stages on the way to industrial implementation and therefore they have also different needs with regard to further development. From a technical and industrial point of view, the following areas have been identified as focus areas for the present on-going research or industrialization projects.
For heat-treatment processes:
• | fast methods for quality and process control; | ||||
• | processing techniques to withstand the heat-treated wood brittleness and the presence of dust during, e.g. mechanical processing due to its low moisture content; | ||||
• | adhesive and paint systems adapted to the low moisture content of heat-treated wood; | ||||
• | new material properties of the heat-treated wood such as UV instability, weak surface (low hardness and fibre–fibre failure), corrosion, bleeding new chemicals through the coating (phenols), low screw-withdrawal burglar resistance, cracks in annual ring orientation, etc.; and | ||||
• | new TH treatments, in contrast to heat-treated wood at high temperature, with fewer undesired effects, to produce heat-treated wood suitable for load-bearing elements and for indoor application. |
Heat and mass transfer are involved in almost all TH and THM wood treatments. The transfer phenomena are at the heart of the size effect during up-scaling.
For compression processes:
• | the primary focus of current research in the domain of wood-moulding and wood densification as well as in post-compression treatment of moulded wood by THM is to overcome the challenges being faced in up-scaling. This becomes highly important while the current aims are to broaden the range of densified wood to encompass large-size wood elements for structural application in the construction industry; and | ||||
• | Ingenuity and creativity for implementing the compressed wood elements with shape memory for developing new wood products. |
For wood welding processes:
• | up-scaling laboratory experiments to an industrial scale; | ||||
• | methods to increase the moisture resistance of the welded joint; | ||||
• | methods to decrease the odour of the welded wood material; and | ||||
• | rapid methods for quality/process development. |
During TH and THM treatment, wood undergoes mechano-chemical transformations which depend upon the process parameters, material properties and element size. An investigation of these phenomena requires collaboration between groups from different wood disciplines. An interdisciplinary approach requires the interaction of researchers from scientific domains such as wood mechanics, wood chemistry, physics, material sciences and numerical analysis. This will help to overcome the challenges being faced in scaling-up research findings, as well improving full industrial production and processes, enhancing product properties and developing new wood products.
Acknowledgements
The support of the European Science Foundation for COST Action FP0904 Thermo-Hydro-Mechanical Wood Behaviour and Processing in conferences, meetings, short-term scientific missions and training schools is gratefully acknowledged.
References
- Aldred , C. 1954 . “ Fine wood-work ” . In A History of Technology. Volume 1: From Early Times to Fall of Ancient Empires , Edited by: Singer , C. , Holmyard , E. J. and Hall , A. R. 697 New York , London : Oxford University Press .
- Bekhta , P. and Niemz , P. 2003 . Effect of high temperature on the change in color, dimensional stability and mechanical properties of spruce wood . Holzforschung , 57 : 539 – 546 . doi: 10.1515/HF.2003.080
- Bevington , J. H. 1891 Spinning tubes . US Patent No. 444 721 .
- Bianchi , S. , Placencia Peña , M. I. , Ganne-Chédeville , C. and Sandberg , D. 2012 Softwood strand-boards manufacturing without adhesive using linear friction welding technology . In Current and Future Trends of Thermo-Hydro-Mechanical Modification of Wood. Opportunities for New Markets? Cost Action FP0904 Workshop , 26–28 March , Nancy , France , pp. 142 – 143 .
- Blomberg , J. 2006 Mechanical and physical properties of semi-isostatically densified wood . PhD thesis No. 2006:28, Luleå University of Technology, Sweden .
- Bobleter , O. and Binder , H. 1980 . Dynamischer hydrothermaler Abbau von Holz [Dynamic hydrothermal degradation of wood] . Holzforschung , 34 : 48 – 51 . doi: 10.1515/hfsg.1980.34.2.48
- Boonstra , M. , Pizzi , A. , Ganne-Chédeville , C. , Properzi , M. , Leban , J.-M. and Pichelin , F. 2006 . Vibration welding of heat-treated wood . Journal of Adhesion Science and Technology , 20 : 359 – 369 . doi: 10.1163/156856106776381758
- Boonstra , M. J. and Tjeerdsma , B. F. 2006 . Chemical analysis of heat treated softwoods . Holz als Roh- und Werkstoff , 64 : 204 – 211 . doi: 10.1007/s00107-005-0078-4
- Boonstra , M. J. , van Acker , J. , Tjeerdsma , B. F. and Kegel , E. V. 2007 . Strength properties of thermally modified softwoods and its relations to polymeric structural wood constituent . Annals of Forest Science , 64 : 679 – 690 . doi: 10.1051/forest:2007048
- Bourgois , J. and Guyonnet , R. 1988 . Characterisation and analysis of torrefied wood . Wood Science and Technology , 22 : 143 – 155 . doi: 10.1007/BF00355850
- Brossman , J. R. 1931 Laminated wood product . US Patent No. 1 834 895 .
- Burmester , A. 1973 . Effect of heat-pressure treatments of semi-dry wood on its dimensional stability . Holz als Roh- und Werkstoff , 31 : 237 – 243 . doi: 10.1007/BF02607268
- Burmester , A. 1974a . Erfolgreiche Quellungsvergütung mit einfachen Mitteln. 1. Die Wärme-Druck-Behandlung – ein Verfahren mit guten Voraussetzungen für die Realisierung [Successful reduction of swelling properties with simple means. 1. The heat and pressure treatment – a procedure with good conditions for the realization] . Holz- und Kunststoffverarbeitung , 8 : 534 – 538 .
- Burmester , A. 1974b . Erfolgreiche Quellungsvergütung mit einfachen Mitteln. 2. Die Wärme-Druck-Behandlung – Behandlung von Holzwerkstoffen-technische Durchführung-Wirtschaftlichkeit [Successful reduction of swelling properties with simple means. 2. The heat and pressure treatment – wood treatment process – implementation – economy] . Holz- und Kunststoffverarbeitung , 9 : 610 – 613 .
- Burmester , A. 1975 . Zur Dimensionsstabilisierung von Holz [The dimensional stabilization of wood] . Holz als Roh- und Werkstoff , 33 : 333 – 335 . doi: 10.1007/BF02612789
- Burtscher , E. , Bobleter , O. , Schwald , W. , Concin , R. and Binder , H. 1987 . Chromatographic analysis of biomass reaction products produced by hydrothermolysis of Poplar wood . Journal of Chromatography , 390 : 401 – 412 . doi: 10.1016/S0021-9673(01)94391-2
- Carrasco , F. and Roy , C. 1992 . Kinetic study of dilute-acid prehydrolysis of xylan-containing biomass . Wood Science and Technology , 26 : 189 – 208 .
- Del Menezzi , C. H. S. and Tomaselli , I. 2006 . Contact thermal post-treatment of oriented strandboard to improve dimensional stability: A preliminary study . Holz als Roh- und Werkstoff , 64 : 212 – 217 . doi: 10.1007/s00107-005-0052-1
- Dietrichs , H. H. , Sinner , H. and Puls , J. 1978 . Potential of steaming hardwoods and straw for feed and food production . Holzforschung , 32 : 193 – 199 . doi: 10.1515/hfsg.1978.32.6.193
- Dwianto , W. , Inoue , M. and Norimoto , M. 1997 . Fixation of compressive deformation of wood by heat treatment . Journal of the Japan Wood Research Society (Nippon Mokuzai Gakki) , 43 : 303 – 309 .
- Dwianto , W. , Norimoto , M. , Morooka , T. , Tanaka , F. and Inoue , M. 1998 . Radial compression of sugi wood (Cryptomeria japonica D. Don) . Holz als Roh- und Werkstoff , 56 : 403 – 411 . doi: 10.1007/s001070050342
- Easterling , K. E. , Harrysson , R. , Gibson , L. J. and Ashby , M. F. 1982 . On the mechanics of balsa and other woods . Proceedings of the Royal Society , A383 ( 1784 ) : 31 – 41 .
- Eggert , O. 1995 Untersuchung der Einflussgrössen beim liegen von Vollholz [Investigation of the influencing factors on solid wood]. PhD thesis, Institut für Werkzeugmaschinen der Universität Stuttgart .
- Ekvall , T. 2006 Miljöaspekter på val av stommaterial i byggnader. Kompletterande kartläggning av kunskapsläget [Environmental considerations for the choice of framing materials in buildings. Additional mapping of the state of knowledge]. (Stockholm: IVL Swedish Environmental Research Institute), Report No. B1663 (in Swedish) .
- Ellis , S. and Paszner , L. 1994 . Activated self-bonding of wood and agricultural residues . Holzforschung , 48 : 82 – 90 . doi: 10.1515/hfsg.1994.48.s1.82
- Erhardt , D. and Mecklenburg , M. F. 1995 Accelerated vs natural aging: Effect of aging conditions on the aging process of cellulose . In P. B. Vandiver . Material Issues in Art and Archaeology IV: Proceedings from the 4th Symposium on Materials Issues in Art and Archaeology , May 16–21 , Cancun Mexico, Material Research Society Symposium Proceedings , Cambridge : Cambrige University Press , Vol. 352 , pp. 247 – 270 , available at: http://dx.doi.org/10.1557/PROC-352-247
- Erhardt , D. , Mecklenburg , M. F. , Tumosa , C. S. and Olstad , T. M. 1996 New versus old wood: Differences and similarities in physical, mechanical, and chemical properties . In J. Brigdland ICOM 11 th Triennial Meeting ( Paris : The International Council of Museums [ICOM] ), Vol. 2 , 903 – 910 .
- Esselen , G. J. 1934 Wood treatment and product . US Patent No. 1 952 664 .
- Esteves , B. , Domingos , I. and Pereira , H. 2008a . Pine wood modification by heat treatment in air . BioResources , 3 ( 1 ) : 142 – 154 .
- Esteves , B. , Domingos , I. , Velez Marques , A. , Nunes , L. and Pereira , H. 2006 Variation of dimensional stability and durability of eucalypt wood by heat treatment . In J. C. Fernando Proceedings of ECOWOOD 2006, 2nd International Conference on Environmentally-Compatible Forest Products Oporto , , Portugal : Fernado Pessoa University pp. 185 – 194 .
- Esteves , B. , Graca , J. and Pereira , H. 2008b . Extractive composition and summative chemical analysis of thermally treated eucalypt wood . Holzforschung , 62 : 344 – 351 . doi: 10.1515/HF.2008.057
- European Committee for Standardization (CEN) . 2008 . CEN/TS 15679 Thermal Modified Timber – Definitions and Characteristics Brussels : European Committee for Standardization .
- Froidevaux , J. , Volkmer , T. , Ganne-Chédeville , C. , Gril , J. and Navi , P. 2012 . Viscoelastic behaviour of aged and non-aged spruce wood in the radial direction . Wood Material Science and Engineering , 7 : 1 – 12 . doi: 10.1080/17480272.2011.629735
- Gabrielli , C. and Kamke , F. A. 2008 . Treatment of chemically modified wood with VTC process to improve dimensional stability . Forest Products Journal , 58 : 82 – 86 .
- Ganne-Chédeville , C. , Duchanois , G. , Pizzi , A. , Leban , J.-M. and Pichelin , F. 2008a . Predicting the thermal behaviour of wood during linear welding using the finite element method . Journal of Adhesion Science and Technology , 22 : 1209 – 1221 . doi: 10.1163/156856108X323688
- Ganne-Chédeville , C. , Duchanois , G. , Pizzi , A. , Pichelin , F. , Properzi , M. and Leban , J.-M. 2008b . Wood welded connections: Energy release rate measurement . Journal of Adhesion Science and Technology , 22 : 169 – 179 . doi: 10.1163/156856108X306939
- Ganne-Chédeville , C. , Jääskeläinen , A.-S. , Froidevaux , J. , Huges , M. and Navi , P. 2012a Naturally and artificially aged wood: A comparsion of spectrochemical data with multivariable analysis . In Current and Future Trends of Thermo-Hydro-Mechanical Modification of Wood. Opportunities for New Markets? Cost Action FP0904 Workshop , 26–28 March , Nancy , France , pp. 28 – 30 .
- Ganne-Chédeville , C. , Jääskeläinen , A.-S. , Froidevaux , J. , Huges , M. and Navi , P. 2012b . Natural and artificial ageing of spruce wood as observed by FTIR-ATR and UVRR spectroscopy . Holzforschung , 66 ( 2 ) : 163 – 170 .
- Ganne-Chédeville , C. , Leban , J.-M. , Properzi , M. , Pichelin , F. and Pizzi , A. 2006a . Temperature and density distribution in mechanical vibration wood welding . Wood Science and Technology , 40 : 72 – 76 . doi: 10.1007/s00226-005-0037-6
- Ganne-Chédeville , C. , Pizzi , A. , Thomas , A. , Leban , J.-M. , Bocquet , J.-F. , Despres , A. and Mansouri , H. 2005 . Parameter interactions, two-block welding and the wood nail concept in wood dowels welding . Journal of Adhesion Science and Technology , 19 : 1157 – 1174 . doi: 10.1163/156856105774429037
- Ganne-Chédeville , C. , Properzi , M. , Leban , J.-M. , Pizzi , A. and Pichelin , F. 2008c . Wood welding: Chemical and physical changes according to the welding time . Journal of Adhesion Science and Technology , 22 : 761 – 773 .
- Ganne-Chédeville , C. , Properzi , M. , Pizzi , A. , Leban , J.-M. and Pichelin , F. 2006b . Parameters of wood welding: A study with infrared thermography . Holzforschung , 60 : 434 – 438 .
- Ganne-Chédeville , C. , Properzi , M. , Pizzi , A. , Leban , J.-M. and Pichelin , F. 2007 . Edge and face linear vibration welding of wood panels . Holz als Roh- und Werkstoff , 65 : 83 – 85 . doi: 10.1007/s00107-006-0125-9
- Gfeller , B. , Pizzi , A. , Zanetti , M. , Properzi , M. , Pichelin , F. , Lehmann , M. and Delmotte , L. 2004 . Solid wood joints by in situ welding of structural wood constituents . Holzforschung , 58 : 45 – 52 . doi: 10.1515/HF.2004.007
- Gfeller , B. , Zanetti , M. , Properzi , M. , Pizzi , A. , Pichelin , F. , Lehmann , M. and Delmotte , L. 2003 . Wood bonding by vibrational welding . Journal of Adhesion Science and Technology , 17 : 1573 – 1589 . doi: 10.1163/156856103769207419
- Giebeler , E. 1983 . Dimensionsstabilisierung von Holz durch eine Feuchte-Wärme-Drück-Behandlung [Dimensional stabilization of wood by moisture-heat-treatment] . Holz als Roh- und Werkstoff , 41 : 87 – 94 . doi: 10.1007/BF02608498
- Gustavsson , L. and Sathre , R. 2011 . Energy and CO2 analysis of timber substitution in construction . Climatic Change , 105 ( 1/2 ) : 129 – 153 . doi: 10.1007/s10584-010-9876-8
- Haller , P. 2007 Manufacturing of wooden profiles by forming . In P. Navi and A. Guidom Proceedings of Third International Symposium on Wood Machining – Fracture Mechanics and Micromechanics of Wood Composites With Regard to Wood Machining , 21–23 May Lausanne , , Switzerland : PPUR , pp. 159 – 162 .
- Haller , P. , Beyer , E. , Wiedemann , G. , Panzner , M. and Wust , H. 2001 Experimental study of the effect of a laser beam on the morphology of wood surfaces . In P. Navi First International Conference of the European Society for Wood Mechanics , 19–21 April , Lausanne , Switzerland .
- Haller , P. and Wehsener , J. 2004 . Festigkeitsuntersuchungen an Fichtenpressholz (FPH) [Mechanical properties of densified spruce] . Holz als Roh- und Werkstoff , 62 : 452 – 454 .
- Haller , P. , Wehsener , J. and Ziegler , S. 2004 Wood profile and method for the production of the same . US Patent No. 10/479439 .
- Hanemann , M. 1917 Holzaufbereitungsverfahren [Wood treatment process]. Deutsches Reichs Reichpatentamt, Patentschrift, No. 318197 .
- Hanemann , M. 1928 Verfahren und Vorrichtung zur Herstellung von weichbiegsamem Holz [Method and apparatus for producing soft and flexible wood]. Deutsches Reichs Reichpatentamt, Patentschrift, No. 458923 .
- Haygreen , J. G. and Daniels , D. H. 1969 . The simultaneous drying and densification of sapwood . Wood and Fiber Science , 1 : 38 – 53 .
- Heger , F. 2004 Etude du phénomène de l’élimination de la mémoire de forme du bois densifié par post-traitement thermo-hydro-mécanique [Study of the mechanisms of elimination of the memory form of densified wood by post-processing thermo-hydro-mechanics]. PhD. thesis No. 3004, EPFL, Lausanne, Switzerland .
- Henning , A. 1942 . Das Schweissen thermoplastischer Kunststoffe [The welding of thermoplastic materials] Deutsche Reich Patent 739 340, 1938 . Kunststoffe , 32 : 103 – 109 .
- Hill , C. A. S. 2006 . Wood Modification: Chemical, Thermal and Other Processes , Chichester , , England : John Wiley & Sons .
- Hillis , W. E. 1975 . The role of wood characteristics in high temperature drying . Journal of the Institute of Wood Science , 7 : 60 – 67 .
- Ibach , R. E. 2010 Specialty treatments . In Wood Handbook – Wood as an Engineering Material. Chapter 19. Forest Products Laboratory . General Technical Report FPL-GTR-190 Madison , WI : Forest Products Laboratory, U.S. Department of Agriculture, Forest Service , pp. 19-1 – 19-16 .
- Inoue , M. , Norimoto , M. , Otsuka , Y. and Yamada , T. 1990 . Surface compression on coniferous wood lumber. Part I. A new technique to compress the surface layer . Mokuzai Gakkaishi , 36 : 969 – 975 .
- Inoue , M. , Norimoto , M. , Tanahashi , M. and Rowell , R. M. 1993 . Steam or heat fixation of compressed wood . Wood and Fiber Science , 25 : 224 – 235 .
- Ito , Y. , Tanahashi , M. , Shigematsu , M. and Shinoda , Y. 1998a . Compressive-molding of wood by high-pressure steam-treatment: Part 2. Mechanism of permanent fixation . Holzforschung , 52 : 217 – 221 . doi: 10.1515/hfsg.1998.52.2.217
- Ito , Y. , Tanahashi , M. , Shigematsu , M. , Shinoda , Y. and Otha , C. 1998b . Compressive-molding of wood by high-pressure steam-treatment: Part 1. Development of compressively molded squares from thinnings . Holzforschung , 52 : 211 – 216 . doi: 10.1515/hfsg.1998.52.2.211
- Jämsä , S. , Ahola , P. and Viitaniemi , P. 2000 . Long-term natural weathering of coated Thermowood . Pigment and Resin Technology , 29 : 68 – 74 . doi: 10.1108/03699420010317807
- Jämsä , S. and Viitaniemi , P. 1998 Heat treatment of wood. Better durability without chemicals . In : Proceedings from Nordiske Trebeskyttelsesdager , 47 – 51 .
- Jämsä , S. and Viitaniemi , P. 2001 Heat treatment of wood: Better durability without chemicals . In A. O. Rapp Review on Heat Treatments of Wood . Proceedings of the special seminar of COST Action E22 , 9 February , Antibes , France , pp. 21 – 26 .
- Johansson , J. and Sandberg , D. 2007 Preparation of wood with pulsed UV-laser ablation for characterisation of the wood structure . In P. Navi and A. Guidoum Proceedings of the Third International Symposium on Wood Machining , 21–23 May , Lausanne , Switzerland , pp. 191 – 194 .
- Kaar , W. E. , Cool , L. G. , Merriman , M. M. and Brink , D. L. 1991 . The complete analysis of wood polysaccharides using HPLC . Journal of Wood Chemistry and Technology , 11 : 447 – 463 . doi: 10.1080/02773819108051086
- Kamdem , D. P. , Pizzi , A. , Guyonnet , R. and Jermannaud , A. 1999 Durability of heat-treated wood . International Research Group on Wood Protection, Document No. IRG/WP/99-40145 .
- Kamke , F. A. 2004 A novel structural composite from low density wood . In X. Zhou, C. Mei, J. Jin and X. Xu (eds.) Proceedings of the 7th Pacific Rim Bio-Based Composites Symposium , Oct. 31–Nov. 2 , Nanjing , China , pp. 176 – 185 .
- Kamke , F. A. 2006 . Densified radiata pine for structural composites . Maderas. Ciencia y Tecnología , 8 : 83 – 92 .
- Kamke , F. A. and Sizemore , H. 2005 Viscoelastic thermal compression of wood . US Patent Application No. US2005/006004AI .
- Kanazawa , F. , Pizzi , A. , Properzi , M. , Delmotte , L. and Pichelin , F. 2005 . Parameters influencing wood-dowel welding by high-speed rotation . Journal of Adhesion Science and Technology , 19 : 1025 – 1038 . doi: 10.1163/156856105774382444
- Kifetew , G. and Wiklund , M. 1999 Hårdpress (Press for densification). Swedish Patent Application No. 9901796-4 .
- Klauditz , W. and Stegmann , G. 1955 . Beiträge zur Kenntnis des Ablaufes und der Wirkung thermischer Reaktionen bei der Bildung von Holzwerkstoffen [Contributions to the knowledge of the sequence and the effect of thermal reactions in the formation of wood materials] . Holz als Roh- und Werkstoff , 13 : 434 – 440 . doi: 10.1007/BF02604722
- Koehler , A. and Pillow , M. Y. 1925 . Effect of high temperatures on the mode of fracture of a softwood . Southern Lumberman , 121 : 219 – 221 .
- Kohara , J. 1952 Studies on the durability of wood I: Mechanical properties of old timbers . Bulletin of Kyoto Prefectural University , 2 , 116 – 131 (in Japanese) .
- Kohara , J. 1953 Studies on the durability of wood V: Shrinkage and swelling of old timbers of 300–1300 years old . Bulletin of Kyoto Prefectural University , 5 , 81 – 88 ( in Japanese ).
- Kollmann , F. 1936 . Technologies des Holzes [Wood technology] , Berlin : Verlag von Julius Springer .
- Kollmann , F. 1955 . Technologies des Holzes und Holzwerkstoffe. Zweiter Band [Wood and wood based materials] , Berlin , Göttingen : Springer-Verlag .
- Kollmann , F. and Fengel , D. 1965 . Änderungen der chemischen Zusammensetzung von Holz duerch thermische Behandlung [Changes in the chemical composition of wood by thermal treatment] . Holz als Roh- und Werkstoff , 23 : 461 – 468 .
- Kučera , L. J. and Bariska , M. 1982 . On the fracture morphology in wood. Part 1: A SEM study of deformations in wood of spruce and aspen upon ultimate axial compression load . Wood Science and Technology , 16 : 241 – 259 . doi: 10.1007/BF00353147
- Kutnar , A. , Kamke , F. A. and Sernek , M. 2008 . The mechanical properties of densified VTC wood relevant for structural composites . Holz als Roh- und Werkstoff , 66 : 439 – 446 . doi: 10.1007/s00107-008-0259-z
- Kutnar , A. , Kamke , F. A. and Sernek , M. 2009 . Density profile and morphology of viscoelastic thermal compressed wood . Wood Science and Technology , 43 : 57 – 68 . doi: 10.1007/s00226-008-0198-1
- Kyomori , K. , Shigematsu , M. , Onwona-Agyeman , S. and Tanahashi , M. 2000 Virtual models of the molding process of wood compressed by the high-pressure steam technique – Towards the development of an aid-system for designing and manufacturing . In H. Thwaites Proceedings of the 6th International Conference on Virtual Systems and MultiMedia (VSMM 2000) , 3–6 October , Ogaki, Gifu , Japan , pp. 403 – 408 .
- Leban , J.-M. , Pizzi , A. , Properzi , M. , Pichelin , F. , GelHaye , P. and Rose , C. 2005 . Wood welding: A challenging alternative to conventional wood gluing . Scandinavian Journal of Forest Research , 20 : 534 – 538 . doi: 10.1080/02827580500432305
- Leban , J.-M. , Pizzi , A. , Wieland , S. , Zanetti , M. , Properzi , M. and Pichelin , F. 2004 . X-ray microdensitometry analysis of vibration-welded wood . Journal of Adhesion Science and Technology , 18 : 673 – 685 . doi: 10.1163/156856104839310
- Leithoff , H. and Peek , R.-D. 1998 Hitzebehandlung – eine Alternative zum chemischen Holzschutz [Heat treatment - an alternative to chemical treatment] . In Tagungsband zur 21. Holzschutz-Tagung [Proceedings of the 21st Wood Protection Conference.] München : Deutsche Gesellschaft für Holzforschung e.V. (DGfH) , 21–23 April , pp. 97 – 108 .
- Lindhe , C. 1993 Process for producing hard elements of wood . Swedish patent Application No. 931118 .
- MacLean , J. D. 1951 . Rate of disintegration of wood under different heating conditions . American Wood Preservers’ Association Proceedings , 47 : 155 – 168 .
- MacLean , J. D. 1953 . Effect of steaming on the strength of wood . American Wood Preservers’ Association Proceedings , 49 : 88 – 112 .
- Mahapatra , K. and Gustavsson , L. 2008 . Multi-storey timber buildings: Breaking industry path dependency . Building Research & Information , 36 : 638 – 648 . doi: 10.1080/09613210802386123
- Militz , H. and Tjeerdsma , B. 2001 Heat treatment of wood by the Plato process . In A. O. Rapp Review on Heat Treatments of Wood . Proceedings of the special seminar of COST Action E22 , 9 February , Antibes , France , pp. 27 – 37 .
- Millett , M. A. and Gerhards , C. C. 1972 . Accelerated aging: Residual weight and flexural properties of wood heated in air at 115°C to 175°C . Wood Science , 4 : 193 – 201 .
- Mori , M. , Nakazwaa , S. , Norimoto , M. , Yamada , T. , Hirano , M. , Takaoka , M. and Muranaka , T. 1984 . Measurement of internal temperature of wood piece during microwave irradiation by fiber-optical temperature sensor . Wood Industry , 39 : 600 – 603 .
- Morsing , N. 2000 . Densification of wood: The influence of hygrothermal treatment on compression of beech perpendicular to the grain . PhD thesis, Series R No. 79, Department of Structural Engineering and Materials, Technical University of Denmark .
- Nairn , J. A. 2006 . Numerical simulations of transverse compression and densification in wood . Wood and Fiber Science , 38 : 576 – 591 .
- Navi , P. , Girardet , F. and Heger , F. 2000 Thermo-hydro-mechanical post-treatment of densified wood . In P. D. Evans Proceedings of 5 th Pacific Rim Bio-Based Composites Symposium , 10–13 December , Canberra , Australia , pp. 439 – 447 .
- Navi , P. and Heger , F. 2005 Comportement thermo-hydromécanique du bois [Behaviour of thermo-hydro-mechanical processed wood] (Lausanne, Switzerland: Presses polytechniques et universitaires romandes) .
- Navi , P. and Sandberg , D. 2012 . Thermo-Hydro-Mechanical Processing of Wood , Lausanne , , Switzerland : EPFL Press .
- Newberry , P. E. 1893 . Archaeological Survey of Egypt, Part I. Beni Hasan , Edited by: Griffith , F. L. London : Kegan Paul, Trench, Trübner & Co Ltd. .
- Nilsson , J. , Johansson , J. , Kifetew , G. and Sandberg , D. 2011 . Shape stability of modified engineering wood product subjected to moisture variation . Wood Material Science and Engineering , 6 : 132 – 139 . doi: 10.1080/17480272.2011.552122
- Norimoto , M. 1979 . Wood bending by microwaves . Wood Research Review , 14 : 13 – 26 .
- Norimoto , M. and Gril , J. 1989 . Wood bending using microwave heating . Journal of Microwave Power and Electromagnetic Energy , 24 : 203 – 212 .
- Norimoto , M. and Hasegawa , K. 1984 Method and apparatus for shaping wood material into a predetermined configuration . US Patent No. 4469156 .
- Norimoto , M. , Ota , C. , Akitsu , H. and Yamada , T. 1993 . Permanent fixation of bending deformation in wood by heat treatment . Wood Research , 79 : 23 – 33 .
- Norimoto , M. , Wada , H. , Hasegawa , K. and Iida , I. 1980 . Wood bending utilizing microwave heating . Journal of Society of Rheology, Japan , 8 : 166 – 171 .
- Obataya , E. 2007 Characteristics of aged wood and Japanese traditional coating technology for wood protection . Actes de la journée d’étude Conserver aujourd'hui: Les “vieillissements” du bois, Cité de la Musique , 2 February , Paris , pp. 26 – 43 .
- Obataya , E. 2009 Effects of ageing and heating on the mechanical properties of wood . In L. Uzielli , Wood Science for Conservation of Cultural Heritage . Proceedings of the International Conference held by COST Action IE0601 , 8–10 November , Florence , Italy , pp. 16 – 23 .
- Ollesheimer , L. J. 1929 Compressed laminated fibrous product and process of making the same . US Patent No. 1 707 135 .
- Ollesheimer , L. J. 1930 Compressed laminated product and process of making the same . US Patent No. 1 747 906 .
- Olsen , A. G. 1934 Process of shrinking wood . US Patent No. 1 981 567 .
- Ormarsson , S. and Sandberg , D. 2007 . Numerical simulation of hot-pressed veneer products – moulding – spring-back – distortion . Wood Material Science and Engineering , 2 : 130 – 137 . doi: 10.1080/17480270801945355
- Ostergard , D. E. 1987 . Bent Wood and Metal Furniture 1850–1946 , New York : The American Federation of Arts .
- Paul , W. , Ohlmeyer , M. and Leithoff , H. 2007 . Thermal modification of OSB-strands by a one-step heat pre-treatment – Influence of temperature on weight loss, hygroscopicity and improved fungal resistance . Holz als Roh- und Werkstoff , 65 : 57 – 63 . doi: 10.1007/s00107-006-0146-4
- Paul , W. , Ohlmeyer , M. , Leithoff , H. , Boonstra , M. J. and Pizzi , A. 2006 . Optimising the properties of OSB by a one-step heat pre-treatment process . Holz als Roh- und Werkstoff , 64 : 227 – 234 . doi: 10.1007/s00107-005-0073-9
- Peters , J. , Fischer , K. and Fischer , S. 2008 . Characterization of emissions from thermally modified wood and their reduction by chemical treatment . BioResources , 3 : 491 – 502 .
- Pfleumer , H. 1922 Verfahren und Vorrichtung zur Herstellung von Preßlingen aus Holz [Method and apparatus for the production of moldings made of wood] . Deutsches Reich Reichspatentamt, Patentschrift Nr. 375 673 .
- Pfleumer , F. and Pfleumer , H. 1915 Verfahren zum Verdichten von Holz [A method for compressing wood] . Kaiserliches Patentamt, Patentschrift Nr. 291 945 .
- Pfriem , A. , Zauer , M. and Wagenführ , A. 2010 . Alteration of the unsteady sorption behavior of maple (Acer pseudoplatanus L.) and spruce (Picea abies (L.) Karst.) due to thermal modification . Holzforschung , 64 : 235 – 241 . doi: 10.1515/hf.2010.029
- Pillow , M. Y. 1929 . Effect of high temperatures on the mode of fracture and other properties of a hardwood . Southern Lumberman , 137 : 58 – 60 .
- Pizzi , A. 2012 Wood joints adhesion and performance in mechanical friction welding of wood without adhesives . In Current and Future Trends of Thermo-Hydro-Mechanical Modification of Wood. Opportunities for New Markets? Cost Action FP0904 Workshop , 26–28 March , Nancy , France , pp. 46 – 47 .
- Pizzi , A. , Leban , J.-M. , Kanazawa , F. , Properzi , M. and Pichelin , F. 2004 . Wood dowel bonding by high-speed rotation welding . Journal of Adhesion Science and Technology , 18 : 1263 – 1278 . doi: 10.1163/1568561041588192
- Popper , R. , Niemz , P. and Eberle , G. 2005 . Untersuchungen zum Sorptions- und Quellungsverhalten von thermisch behandeltem Holz [Investigations on sorption and swelling properties of thermally treated wood] . Holz als Roh- und Werkstoff , 63 : 135 – 148 . doi: 10.1007/s00107-004-0554-2
- Prodehl , A. 1931a . Zur Holzbiegetechnik [About the wood bending technology] . Zeitschrift des Vereines deutscher Ingenieure , 75 : 1217 – 1222 .
- Prodehl , A. 1931b Untersuchungen über das Biegen gedämpften Holzes [Studies on the bending of steamed wood]. PhD thesis , Sachsischen Technischen Hochschule zu Dresden .
- Putzger , R. , Eckhard , R. , Eichhorn , S. , Nendel , K. and Haller , P. 2012 Investigation on fibre reinforced moulded wood pipes for the conduction of brine at high temperature and pressure . In Current and Future Trends of Thermo-Hydro-Mechanical Modification of Wood. Opportunities for New Markets? Cost Action FP0904 Workshop , 26–28 March , Nancy , France , pp. 174 – 176 .
- Rapp , A. O. , Brischke , C. and Welzbacher , C. R. 2006 . Interrelationship between the severity of heat treatments and sieve fractions after impact ball milling: A mechanical test for quality control of thermally modified wood . Holzforschung , 60 : 64 – 70 . doi: 10.1515/HF.2006.012
- Rapp , A. O. and Sailer , M. 2001 Oil heat treatment of wood in Germany: State of the art . In A. O. Rapp Review on Heat Treatments of Wood . Proceedings of the special seminar of COST Action E22, Antibes , 9 February , France , pp. 47 – 64 .
- Rapp , A. O. , Sailer , M. and Westin , M. 2000 Innovative Holzvergütung – neue Einsatzbereiche für Holz [Innovative improvement of wood] . In Tagungsband zur 17. Dreiländer-Holztagung 2000. Schweizerische Arbeitsgemeinschaft für Holzforschung [Proceedings of the 17th three countries - Wood Meeting 2000th Swiss Group for Holzforschung] Zürich : Holz ART , pp. 153 – 160 .
- Rautkari , L. 2012 Surface modification of solid wood using different techniques . PhD Thesis No. 4/2012, Department of Forest Products Technology, School of Chemical Technology, Aalto University .
- Rhême , M. , Botsis , J. , Cugnoni , J. and Parviz , N. 2011 Fracture mechanics characteristics of welded joint of wood . In P. Navi and A. Roth Mechano-Chemical Transformations of Wood During THM Processing. Cost Action FP0904 Workshop , 16–18 February , Biel , Switzerland , pp. 117 – 118 .
- Rhême , M. , Botsis , J. , Cugnoni , J. and Parviz , N. 2012 Mode I and II fracture characteristics of welded wood bonds . In Current and Future Trends of Thermo-Hydro-Mechanical Modification of Wood. Opportunities for New Markets? Cost Action FP0904 Workshop , 26–28 March , Nancy , France , pp. 116 – 117 .
- Rivers , S. and Umney , N. 2005 Conservation of Furniture Butterworth-Heinemann , Oxford : Elsevier .
- Rowell , R. M. 2009 Heat-Treated Wood (AccessScience, McGraw-Hill Companies). Accessed 25 April 2012, available at: www.accessscience.com%2fcontent.aspx%3fsearchStr%3dRowell%26id%3dYB090002
- Rowell , R. M. , Ibach , R. E. , McSweeny , J. and Nilsson , T. 2009 . Understanding decay resistance, dimensional stability and strength changes in heat treated and acetylated wood . Wood Material Science and Engineering , 4 : 14 – 22 . doi: 10.1080/17480270903261339
- Sailer , M. , Rapp , A. O. , Leithoff , H. and Peek , R.-D. 2000 . Vergütung von Holz durch Anwendung einer Öl-Hitze-Behandlung [Upgrading of wood by application of an oil-heat treatment] . Holz als Roh- und Werkstoff , 58 : 15 – 22 . doi: 10.1007/s001070050379
- Sandberg , D. 1998 Inverkan av isostatisk komprimering på cellstrukturen . [Influence of iso-static compression on the wood cell structure] (Stockholm: KTH Royal Institute of Technology, Wood Technology and Processing), Report No. TRITA-TRÄ R-98-35 ISSN 1104-2117 .
- Sandberg , D. 1999 . Weathering of radial and tangential wood surfaces of pine and spruce . Holzforschung , 53 : 355 – 364 . doi: 10.1515/HF.1999.059
- Sandberg , D. and Söderström , O. 2006 . Crack formation due to weathering of radial and tangential sections of pine and spruce . Wood Material Science and Engineering , 1 : 12 – 20 . doi: 10.1080/17480270600644407
- Sears , C. 1900 Process of preparing wood matrices . US Patent No. 646 547 .
- Seborg , R. M. , Millet , M. A. and Stamm , A. J. 1945 . Heat stabilized compressed wood: Staypack . Mechanical Engineering , 67 : 25 – 31 .
- Seborg , R. M. and Stamm , A. J. 1941 . The compression of wood . Mechanical Engineering , 63 : 211 – 213 .
- Seltman , J. 1995a . Abtragen von Holzoberflächen durch UV-bestrahlung [Removal of wood surfaces by UV irradiation] . Holz als Roh- und Werkstoff , 53 : 100 doi: 10.1007/BF02716401
- Seltman , J. 1995b . Freilegen der Holzstruktur durch UV Bestrahlung [Opening the wood structure by UV-irradiation] . Holz als Roh- und Werkstoff , 53 : 225 – 228 . doi: 10.1007/s001070050076
- Shigematsu , M. , Fukaya , M. , Sasaki , Y. and Tanahashi , M. 1998 Three dimensional image analysis on molding process of wood compressed by high-pressure steam technique . In H. Thwaites Futurefusion: Application Realities for the Virtual Age . Proceedings of 4th International Conference on Virtual Systems and Multimedia, VSMM'98 , 18–20 November , Gifu , Tokyo pp. 372 – 379 .
- Stamm , A. J. 1942 Antishrink treatment for wood . US Patent No. 2 296 316 .
- Stamm , A. J. 1956 . Thermal degradation of wood and cellulose . Industrial and Engineering Chemistry , 48 : 413 – 417 . doi: 10.1021/ie51398a022
- Stamm , A. J. 1964 . Wood and Cellulose Science , New York : Ronald Press .
- Stamm , B. 2005 Development of friction welding of wood –physical, mechanical and chemical studies . PhD thesis No. 3396 , Ecole Fédérale de Lausanne , Switzerland .
- Stamm , A. J. , Burr , H. K. and Kline , A. A. 1946 . Staybwood. Heat-stabilized wood . Industrial and Engineering Chemistry , 38 : 630 – 634 . doi: 10.1021/ie50438a027
- Stamm , A. J. and Hansen , L. A. 1937 . Minimizing wood shrinkage and swelling. Effect of heating in various gasses . Industrial and Engineering Chemistry , 29 : 831 – 833 . doi: 10.1021/ie50331a021
- Stamm , B. , Natterer , J. and Navi , P. 2005a . Joining wood by friction welding . Holz als Roh- und Werkstoff , 63 : 313 – 320 . doi: 10.1007/s00107-005-0007-6
- Stamm , B. , Natterer , J. and Navi , P. 2005b . Joining of wood layers by friction welding . Journal of Adhesion Science and Technology , 19 : 1129 – 1139 . doi: 10.1163/156856105774429046
- Stamm , A. J. and Seborg , R. M. 1941 . Resin-treated, laminated, compressed wood . Transaction of the American Institute of Chemical Engineers , 37 : 385 – 398 .
- Stamm , A. J. , Seborg , R. M. and Millet , M. A. 1948 Method of forming compressed wood structures . US Patent No. 2 453 679 .
- Stamm , B. , Windeisen , E. , Natterer , J. and Wegener , G. 2005c . Thermal behaviour of polysaccharides in wood during friction welding . Holz als Roh- und Werkstoff , 63 : 388 – 389 . doi: 10.1007/s00107-005-0002-y
- Stamm , B. , Windeisen , E. , Natterer , J. and Wegener , G. 2006 . Chemical investigation on the thermal behaviour of wood during friction welding . Wood Science and Technology , 40 : 615 – 627 . doi: 10.1007/s00226-006-0097-2
- Stingl , R. , Patzelt , M. and Teischinger , A. 2002 . “ Ein- und Rückblick in ausgewählte Verfahren der thermischen Modifikation [A review of selected processes of thermal modification] ” . In Lignovisionen, Band 3: Modifiziertes Holz. Eigenschaften und Märkte [Lignovisionen, Volume 3: Modified wood. Properties and Markets] , Edited by: Teischinger , A. and Stingl , R. 57 – 99 . Wien : Institut für Holzforschung (ihf) und Verband Holzwirte Österreichs (VHÖ), Universität für Bodenkultur .
- Suthoff , B. and Kutzer , H.-J. 1997 Verfahren zum reibschweiβartigen Verbinden von Holz [Method for joining wood]. Offenlegungungsschrift DE 19746 782 A1. Deutsches Patent und Markenamt .
- Suthoff , B. , Schaaf , A. , Hentschel , H. and Franz , U. 1996 Verfahren zum reibschweiβartigen Fügen von Holz [Method for joining wood]. Offenlegungungsschrift DE 196 20 273 A1 . Deutsches Patent und Markenamt .
- Tanahashi , M. 1990 . Characterization and degradation mechanisms of wood components by steam explosion and utilization of exploded wood . Wood Research , 77 : 49 – 117 .
- Tanahashi , M. , Kyomori , K. , Natsume , Y. , Okawa , S. , Shigematsu , M. and Onwona-Agyeman , S. 2000 Development of aggregated-woods and assembled-woods by compressive molding process from small logs under high-pressure steam with and without any adhesives . In : P. D. Evans 5th Pacific Rim Bio-Based Composites Symposium , Canberra , Australia , pp. 456 – 462 .
- Tatsuya , S. 2007 Method of processing wood . US Patent No. 7 296 604 B2 .
- Teichgräber , R. 1966 . Beitrag zur Kenntnis der Eigenschaftsänderungen des Holzes beim Dämpfen [On the alteration of the properties of wood during steaming] . Holz als Roh- und Werkstoff , 24 : 548 – 551 . doi: 10.1007/BF02610360
- Tiemann , H. D. 1915 . The effect of different methods of drying on the strength of wood . Lumber World Review , 28 : 19 – 20 .
- Tiemann , H. D. 1920 The Kiln Drying of Woods for Airplanes Washington , DC : National Advisory Committee for Aeronautics , Report No. 65 .
- Tiemann , H. D. 1941a . Does temperature injure wood? . Southern Lumberman , 163 ( 2047 ) : 49 – 50 .
- Tiemann , H. D. 1941b . Does temperature injure wood? . Southern Lumberman , 163 ( 2049 ) : 49 – 51 .
- Tiemann , H. D. 1941c . Does temperature injure wood? Reduction in hygroscopicity . Southern Lumberman , 163 ( 2051 ) : 55 – 57 .
- Tjeerdsma , B. F. 2006 . Heat Treatment of Wood – Thermal Modification , Wageningen , , The Netherlands : SHR Timber Research .
- Vaziri , M. 2011 Water resistance of Scots pine joints produced by linear friction welding . PhD thesis , Luleå University of Technology .
- Vernois , M. 2001 Heat treatment of wood in France – State of the art . In A. O. Rapp , Review on Heat Treatments of Wood . Proceedings of the special seminar of COST Action E22, 9 February, Antibes , France , pp. 39 – 46 .
- Viitaniemi , P. and Jämsä , S. 1996 Puun modifiointi lämpökäsittelyllä [Modification of wood with heat treatment] (Technical Research Centre of Finlad, VTT), Report No. 184 .
- Vill , V. I. 1959 Friction welding of metals (translated title) ( Moskow-Leningrad: Mashgiz ).
- Vill , V. I. and Shternin , L. A. 1957 The basic technology of friction welding (translated title) . Glavelektroadard , 4 .
- Vorreiter , L. 1942 . Gehärtete und mit Metall oder Öl getränkte Hölzer [Densified and with oil or metal treated wood] . Holz als Roh- und Werkstoff , 5 : 59 – 69 . doi: 10.1007/BF02605309
- Walsh , F. J. and Watts , R. L. 1923 Composite lumber . US Patent No. 1 465 383 .
- Welzbacher , C. R. , Brischke , C. and Rapp , A. O. 2007 . Influence of treatment temperature and duration on selected biological, mechanical, physical, and optical properties of thermally modified timber . Wood Material Science and Engineering , 2 : 66 – 76 . doi: 10.1080/17480270701770606
- Welzbacher , C. R. , Wehsener , J. , Rapp , A. O. and Haller , P. 2008 . Thermo-mechanical densification combined with thermal modification of Norway spruce (Picea abies Karst) in industrial scale. Dimensional stability and durability aspects . Holz als Roh- und Werkstoff , 66 : 39 – 48 . doi: 10.1007/s00107-007-0198-0
- Westin , M. , Rapp , A. O. and Nilsson , T. 2004 Durability of pine modified by 9 different methods . International Research Group on Wood Protection, Document No. IRG/WP/04-40288 .
- Westin , M. , Sterely , M. , Rossi , F. and Herve , J.-J. 2009 . Compreg-type products by furfurylation during hot-pressing . Wood Material Science and Engineering , 4 ( 1/2 ) : 67 – 75 . doi: 10.1080/17480270903350314
- Wienhaus , O. 1999 . Modifizierung des Holzes durch eine milde Pyrolyse – abgeleitet aus den allgemeinen Prinzipien der Thermolyse des Holzes [Modification of wood by mild pyrolysis - derived from the general principles of the thermolysis of wood] . Wissenschaftliche Zeitschrift der Technischen Universität Dresden , 48 ( 2 ) : 17 – 22 .
- Wilson , T. R. C. 1920 The Effect of Kiln Drying on the Strength of Airplane Woods Washington , DC : National Advisory Committee for Aeronautics , Report No. 68 .
- Yildiz , S. , Gezer , E. D. and Yildiz , U. C. 2006 . Mechanical and chemical behavior of spruce wood modified by heat . Building and Environment , 41 : 1762 – 1766 . doi: 10.1016/j.buildenv.2005.07.017