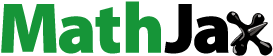
ABSTRACT
Wood is one of the most important building materials. During the service life of wood and derived materials, various degradation factors affect performance. To assess how weathering influenced the material resistance and moisture dynamics of wood, 11 different materials were exposed to natural weathering for 9, 18 and 27 months or artificial accelerated weathering. Afterwards, the moisture performance of wood was determined in line with the Meyer-Veltrup procedure. Weathered samples were also exposed to the brown-rot fungus Gloeophyllum trabeum for 16 weeks. Respective materials were classified into durability classes according to EN 350, and relative resistance dose (Drd rel) was calculated. Weathering resulted in leaching of biologically active extractives, changed surface morphology and increased permeability. All these changes were reflected in decreased relative resistance dose for all tested materials. The largest deceases were determined for thermally modified wood, Scots pine, European larch and sweet chestnut heartwood.
Introduction
Wood in building applications is gaining importance (Gasparri et al. Citation2015). Consumers are choosing wood predominantly due to environmental awareness and positive health impacts (Strobel et al. Citation2017, Lähtinen et al. Citation2019, Malá et al. Citation2019). In order to use wood in moist and outdoor applications, the durability characteristic must meet the specified criteria. Restrictions on many classic biocides due to environmental and health concerns (Humar et al. Citation2006), and negative public opinion on the existing biocides and the use of tropical timber, have altered research philosophies to improve the durability and service life of wood (Militz Citation2020). Unfortunately, the majority of wood species in Europe are not durable (Brischke et al. Citation2013, CEN Citation2016); therefore, we need to understand the performance of wood better or develop alternative treatments to improve relevant properties of wood through processes such as wood modification (Hill Citation2011, Petrič Citation2013).
The majority of durability experiments have been performed on freshly cut wood. When wood is exposed to the weathering, a wide range of biotic and abiotic factors may affect its performance. The chemical structure of the wood surface changes as lignin is depolymerised due to the UV-radiation and subsequently leached during rain events (Petrič Citation2013). This results in increased cellulose content in the surface of weathered wood (Hazneza and Evans Citation2016, Kropat et al. Citation2020). In addition, the surface is frequently more hydrophilic (Žlahtič and Humar Citation2016a), and microcracks form (Van Acker et al. Citation2015). Larger cracks may also form due to dimensional changes, temperature differences and wood heterogeneity (Osawa et al. Citation2019, Alao et al. Citation2020). Moreover, wood in the outdoor exposure is frequently colonised by blue stain fungi (Kržišnik et al. Citation2018a, Lie et al. Citation2019). All of these factors increase permeability and reduce the water resistance of the wood (Žlahtič-Zupanc et al. Citation2018). Recent durability models indicate that wood durability in aboveground applications is considerably affected by water exclusion efficacy (Meyer-Veltrup et al. Citation2017). Therefore, lower water resistance results in greater wood susceptibility to degradation (Bornemann et al. Citation2014).
In addition to water performance, durability is also affected by material resistance (inherent durability). Material resistance is mainly characterised by the presence of biologically active substances such as extractives (naturally present) or biocides (after impregnation). Extractives include a large variety of compounds that can be classified into lipophilic and hydrophilic extractives, i.e. compounds soluble in non-polar and polar solvents, respectively (Vek, Poljanšek, et al. Citation2019, Vek, Vivod, et al. Citation2019). Phenolic compounds are recognised to have a significant impact on decay resistance (Schultz and Nicholas Citation2000, Venäläinen et al. Citation2004). Phenolic extractives are reported as natural compounds with antioxidant, antimicrobial and antifungal properties (Belt et al. Citation2017). However, the leachability of extractives or biocides from wood can result in a decrease in durability and eventual failure. The effect of leaching or weathering prior to durability testing on the natural durability of wood remains unclear (Morris and Stirling Citation2012, Kirker et al. Citation2013, Brischke et al. Citation2014, Thaler, Žlahtič, et al. Citation2014).
The objective of study was to elucidate the influence of natural and artificial weathering on durability classification according to EN 350 (CEN Citation2016) as well as on the material resistance and wetting ability. These two parameters were used to calculate the durability of wood according to the Meyer-Veltrup model (Meyer-Veltrup et al. Citation2017). This model has already been successfully applied and validated on naturally durable wood species and modified wood (Brischke et al. Citation2017, Kržišnik et al. Citation2018b, Humar et al. Citation2019).
Material and methods
The present research is a follow-up of previous studies (Žlahtič and Humar Citation2016a, Citation2016b). Samples prepared and analysed in previous studies were used for the experiment. The preparation of the specimens and ageing procedures are described in detail in respective articles.
The wood species chosen for this research are important in Europe: sweet chestnut heartwood (Castanea sativa), oak heartwood (Quercus sp.), European beech wood (Fagus sylvatica), Scots pine heartwood and sapwood (Pinus sylvestris), European larch heartwood (Larix decidua) and, Norway spruce (Picea abies) (). Norway spruce served as the reference in some novel service life prediction models (Meyer-Veltrup et al. Citation2017). Defect-free cube blocks (1.5 cm × 2.5 cm × 5.0 cm) were cut as prescribed in European standard EN 113 (CEN Citation2006). Some of the materials were treated with a copper-based wood preservative or thermally modified (). The experiment was performed on five replicates per treatment. There were 275 samples prepared for this analysis.
Table 1. Materials and treatments used in respective experiments.
Treatment of specimens
Eleven different materials were prepared for this experiment. The thermal modification was performed for three hours at 230°C on Norway spruce and 215°C on beech wood according to the commercial Silvapro® process for each species (Rep et al. Citation2012). Control and thermally modified wood samples were treated with a commercial copper-ethanolamine (CuEA) formulation (Silvanolin, Silvaprodukt) (Humar and Lesar Citation2008) () to Use Class 3 requirements (aboveground and uncovered) (CEN Citation2013b). Copper treatment was selected as a model treatment to improve the fungicidal properties of non-durable wood (Preston Citation2000). Impregnation was performed using a full-cell process, i.e. 30 min vacuum (8 kPa), 120 min pressure (900 kPa), 15 min vacuum (8 kPa) and 20 min immersion at ambient conditions.
Ageing procedures
Artificial accelerated weathering (AAW) and outdoor ageing, with three different exposure times, were applied. AAW was carried out in a chamber (ATLAS UP, Suntest XXL+, Linsengericht, Germany). Weathering conditions were applied according to EN/ISO 11341 (CEN Citation2004), which simulates outdoor weathering without the contribution of biotic factors since severe UV radiation limits fungal growth. The specimens were exposed to alternating cycles of elevated temperature (38°C) and relative humidity (68%) with UV radiation (0.35 W/m2) and artificial rain for 1000 h. One cycle of AAW lasted for 120 min and consisted of water spray (18 min) and UV radiation (102 min). In addition, three sets of treated and reference specimens were exposed to outdoor weathering in a horizontal position in the field test site of the University of Ljubljana, Ljubljana, Slovenia (N 46°02′55.4 ″, E 14°28′44.6″, 293 m above sea level). The climate in Ljubljana could be summarised by the following parameters: the average temperature 10.4°C, the annual precipitation 1290 mm and a Scheffer Climate Index of 55.3. Actual climate data were previously reported (Žlahtič-Zupanc et al. Citation2018). The first set of exposed material (Out-A) remained outside from January 2014 to October 2014. The second set of materials (Out-B) was exposed for 18 months, from January 2014 until August 2015, while the third set (Out-C) of specimens was exposed for 27 months (May 2016). Climate data during respective periods are summarised in . All samples were air-dried in a desiccator stored in the dark under laboratory conditions until further characterisation.
Table 2. Summary of the climate data during three exposure periods (ARSO Citation2020).
Determination of factors describing inherent durability (kinh)
The agar block test with a pure fungal culture of a G. trabeum was used to assess inherent durability; following the modified procedure CEN/TS 15083-1 (CEN Citation2005). Steam-sterilised specimens were exposed to the brown rot fungus (Gloeophyllum trabeum (Pers.) Murrill (ZIM L018)) in 350-mL glass jars containing 50 mL of potato dextrose agar (DIFCO, Fisher Scientific, Franklin Lakes, NJ, USA). The fungal isolate originated from the fungal collection of the Biotechnical Faculty, University of Ljubljana, and is available on-demand (Raspor et al. Citation1995). This fungal species was chosen as it is more frequently found in above-ground, outdoor applications than Coniophora puteana. Thus we suspected that this fungus would be the most representative degradation organism. One week after inoculation, two random specimens were placed on an HDPE mesh in each jar. The assembled test jars were then incubated (25°C and 80% relative humidity (RH)) for 16 weeks, as prescribed in the standard. After 16 weeks, specimens were cleaned of adhering fungal mycelium, oven-dried at 103 ± 2°C, and weighed to calculate the mass loss. Five replicate specimens for each of the materials were tested. Samples used for assessment of inherent durability were prior fungal decay used for assessment of wetting ability.
Determination of factors describing wetting ability (kwa)
Wetting ability was assessed on five replicate samples of each material. The average relative values of the multiple tests were combined to calculate the wetting ability factor.
Short-term capillary water uptake was carried out on a Tensiometer K100MK2 (Krüss, Hamburg, Germany), according to a modified EN 1609 (CEN Citation2013a). The axial surfaces of the specimens were positioned to be in contact with the distilled water for 200 s. The uptake of water was expressed in g/cm2 based on the final mass of the immersed sample and the exposed surface. Long-term water uptake specimens were oven-dried (103 ± 2°C) until constant mass and weighed. The dry wooden blocks were positioned in a jar and weighted to prevent them from floating before 100 g of distilled water was added per specimen. Specimens were weighed after 24 h, and the MC of five replicate specimens was calculated.
A water vapour sorption test in a water-saturated atmosphere followed by drying above freshly activated silica gel was performed to determine sorption properties. Specimens were oven-dried (103 ± 2°C) and weighed before being placed on mesh in a glass climate chamber with a fan above distilled water. Their mass was determined after 24 h of conditioning, and the respective MC was calculated. Specimens were then conditioned under the same conditions for a further three to four weeks until a constant mass was achieved. After conditioning, specimens were positioned above freshly activated silica gel for 24 h in a closed container. The MC of the wood was calculated according to the procedure described by Meyer-Veltrup (Meyer-Veltrup et al. Citation2017). Five replicate specimens were used for this analysis.
Factor approach for quantifying the resistance dose (DRd)
The data were modelled using procedures described by Meyer-Veltrup et al. (Citation2017) and Isaksson et al. (Citation2013) to predict the field performance of the examined materials. The acceptability of the selected design and material was expressed as follows:
(1)
(1)
The exposure was expressed as an exposure dose (DEd), based on the daily mean values of temperature and wood MC. The material property was stated as the resistance dose (DRd) in days [d], with optimal wood moisture content and wood temperature conditions for fungal decay (Isaksson et al. Citation2013):
(2)
(2) where DEd is the exposure dose [d], and DRd is the resistance dose [d].
The exposure dose DEd was based on the annual dose at a specific geographical location. The respective study focused on the counterpart of the exposure dose, which is the resistance, expressed as resistance dose DRd. This is the product of the critical dose Dcrit and two factors expressing the inherent durability (kinh) and its wetting ability of wood (kwa). The approach is given by EquationEquation (3)(3)
(3) (Isaksson et al. Citation2014) ()
(3)
(3) where Dcrit is the critical dose corresponding to decay rating 1 (slight decay; EN 252 (CEN Citation2015)), kwa is a factor accounting for the wetting ability of the tested materials [–], relative to the reference spruce, and kinh is a factor accounting for the inherent protective properties of the tested wood species against decay [–], relative to the reference wood species Norway spruce. Namely, the inherent durability and wetting ability of the reference wood species were set to 1. Wood species or modified wood with either of these values greater than those determined for Norway spruce had higher values but limited to five.
Table 3. Description of key terms addressed of the Meyer-Veltrup model.
Based on the various moisture tests presented in this paper, the wetting ability factor kwa was calculated. The methodology for calculating kwa followed the Meyer-Veltrup procedure (Meyer-Veltrup et al. Citation2017), except that the she prescribed specimens (0.5 × 1.0 × 10.0 cm3) with different dimensions than those used in the present experiment (1.5 × 2.5 × 5.0 cm3). Sample size has little influence on the model’s result, since the approach was based on relative values. Results from fungal durability tests were used to evaluate the inherent resistance factor kinh. These two factors were used to determine the resistance dose DRd of the 11 wood materials examined in this study. Only brown rot was applied to determine kinh in this research. Terrestrial microcosm tests, white rot durability and in-ground durability tests were not performed, as prescribed by the original Meyer-Veltrup approach (Meyer-Veltrup et al. Citation2017).
The data were analysed with Microsoft Excel and GraphPad prism. For mass loss results (for each type of materials separately), the total variance was analysed using a one-way ANOVA and Duncan’s multiple comparisons test (p = 0.95) at a 5% significance level.
Results and discussion
Mass changes are the most basic indicators of changes in wood specimens during artificial or natural weathering. gives the average mass changes after natural or artificial weathering. Weathering resulted in mass losses caused predominately by leaching of extractives and partially due to degradation and consumption of easily accessible sugars by microorganisms (Thaler, Brischke, et al. Citation2014). Blue stain colonisation can be resolved from the melamine deposits on the surface of weathered wood, which can be easily resolved with the microscope. Mass loss during weathering increased with the duration of weathering (). For example, mass loss of the sweet chestnut specimens (Cs) increased from 2.5% after 9 months of weathering (Out-A) to 5.7% after 27 months of weathering (Out-C). The highest mass losses were observed on larch specimens (Ld), which lost 7.5% after 27 months (Out-C). The lowest mass losses were found with spruce (Pa) and pine sapwood (PsS) specimens, which had average losses of 3.0% after 27 months of weathering (Out-C). Considerable mass loss occurred with thermally modified wood. Mass loss of thermally modified wood was higher than that of non-modified controls, presumably due to the leaching of partially degraded cell wall components (Baysal et al. Citation2014). Artificial weathering resulted in mass losses comparable to 9–18 months of natural weathering ().
Table 4. Effect of outdoor (Out) and artificial (AAW) weathering on mass loss of the specimens.
The test fungus is one of the most frequently found wood decay fungi on softwoods used in outdoor applications in Central Europe, but also degrades hardwoods (Schmidt Citation2006). Mass losses of non-weathered, non-durable wood species spruce, pine-sapwood and beech exceeded 30%, proving that the conditions were suitable for aggressive fungal attack (CEN Citation2005). Mass losses of other wood species were considerably lower. Mass losses of the PaCu, PaTm, PaTmCu, FsTm, Cs and Q are below 3% and thus considered insignificant by the respective standard (CEN Citation2005). Only mass losses of larch (Ld; 5.5) and Scots pine heartwood (PsH; 6.0%) were a bit higher, but still provided good inherent durability of materials against the tested fungus ().
Table 5. Effect of outdoor (Out) and artificial (AAW) weathering on median mass loss of specimens exposed to the brown-rot fungus Gloeophylum trabeum in an agar block test.
Exposed weathered samples produced three types of patterns. Only copper treated thermally modified wood specimens retained inherent durability after 27 months of weathering. This phenomenon can be attributed to the fact that thermally modified wood was treated with biocides. This synergistic effect has already been reported after five years of outdoor testing (Humar et al. Citation2019). The second pattern was noted for wood specimens that exhibited partial durability (Q, Cs, Ld, PSH), thermally modified wood (PaTm, FsTM) and copper treated wood (PaCu). The durability of all respective wood materials decreased after weathering. A very notable decrease was observed for sweet chestnut wood. Non-weathered wood mass loss was 1.0%, while mass loss weathered specimens gradually increased with weathering duration. Mass losses of 27 months weathered sweet chestnut (Out-C) reached 17.9% after exposure to brown rot fungus (). This durability decrease can be presumably attributed to the leaching of extractives during weathering (5.7%; Out-C) (). A statistically significant correlation (r = 0.7978; p < 0.0001) between mass loss during weathering and mass loss during brown-rot decay can be resolved in . A similar relationship was found with other wood species with biologically active extractives like larch, Scots pine heartwood, and oak. The third pattern was noticed with the non-durable wood species beech, spruce and Scots pine sapwood. Fungal degradation of these wood species was considerably greater than found for the other tested materials. However, weathered pine and spruce mass loss were on average lower than the mass loss of non-weathered specimens. We are not sure what is the prime reason for this occurrence, but it could result from other fungi or bacteria present in wood (sapstain) that could have an antagonistic effect on the brown rot fungus (Johnston et al. Citation2016, Jung et al. Citation2018) or leaching of nutrients. The effect of artificial weathering was comparable to 9 months of natural weathering.
Figure 1. Correlation between mass loss after weathering and mass loss caused by G. trabeum on sweet chestnut wood.
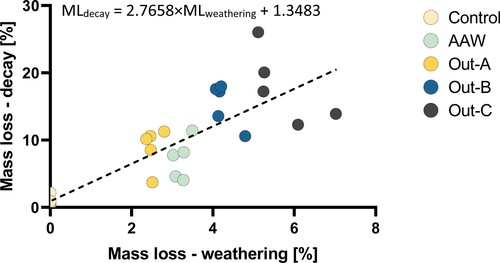
EN 350 standard (CEN Citation2016) enables classification of thermally modified wood and wood treated with biocides to durability classes, based on the median mass loss. As expected, Scots pine, Norway spruce and beech were classified as non-durable species (DC 5). This classification remained relatively constant even after weathering, except for Scots pine sapwood after 18 months of natural or artificial weathering (). On the other hand, the durability of naturally durable heartwoods decreased considerably. For example, the durability class of larch heartwood decreased from DC2 (durable) to DC3 (moderately durable). An even more prominent decrease was found with Scots pine heartwood, which decreased from DC2 to DC5 (not-durable) with weathering. Comparable results were found for sweet chestnut as well (decrease from DC1 to DC4). The durability decrease was also determined for thermally modified wood but was less prominent than for sweet chestnut. Oak (Q) and copper treated wood experienced no decrease in durability classification (). It is likely, that the extractives or copper remained in the wood after weathering, providing inherent durability.
Table 6. Durability classification of the materials based on EN 350 (CEN Citation2016) and factors defining the service life of wood: kinh – inherent durability, kwa – wetting ability and Drd rel – relative resistance dose.
However, durability classification has a few limitations. The durability classification system itself cannot estimate the expected service life of a timber structure (Isaksson et al. Citation2013). Therefore, Meyer-Veltrup et al. (Citation2017) proposed a new method to determine wood durability, based on wetting ability and a factor accounting for the inherent protective properties of the tested wood species against fungal decay. Biologically active extractives do not fully explain material resistance, but moisture tests may help explain some elements of performance. Wood that uptakes less water during rainfall events and/or releases water faster will be more resistant to fungal degradation (Humar et al. Citation2019). Therefore, special attention was given to factor kinh, which describes the wetting ability of wood. Resistance to moisture uptake decreased after weathering for all wood species. Colonisation by blue stain fungi, the formation of microcracks, degradation of pit membranes and degradation of resin could all affect moisture behaviour (Žlahtič-Zupanc et al. Citation2018). The highest decrease in water resistance was found with thermally modified wood (), possibly because increased roughness altered surface chemical composition and produced microcracks (Van Acker et al. Citation2015, Žlahtic et al. Citation2015).
The decrease in inherent durability (kinh) and moisture resistance (kwa) after weathering should decrease relative resistance dose (Drd rel) (). Relative resistance dose of all tested wood species decreased. The smallest decrease was found for Norway spruce wood, where inherent durability decreased by only 4%. The largest decrease occurred with thermally modified beech (FsTm), where Drd rel decreased from 8.3 to 1.5 after 27 months of weathering (Out-C). A similar decrease was noted for Scots pine heartwood, where Drd rel decreased from 5.5 to 0.7. Notable decreases were determined for the sweet chestnut, thermally modified spruce and larch (). This decrease of Drd rel is a result of decreased inherent durability and wetting ability.
Conclusions
Artificial and natural weathering resulted in the leaching of extractives from wood, which negatively influenced inherent durability, as evident from the mass losses caused by G. trabeum. Weathering negatively influenced moisture resistance. This phenomenon was evident for non-treated, copper impregnated and modified wood species. This was reflected in the decreased relative resistance dose and was evident for all tested materials. The most notable decreases were noted for thermally modified wood, Scots pine heartwood, larch and sweet chestnut heartwood.
Acknowledgements
The authors acknowledge the financial support of the Slovenian Research Agency (ARRS) within the research program P4-0015 (Wood and lignocellulosic composites), the infrastructural centre (IC LES PST 0481-09) and for the financing of the PhD of the first author. Part of the published research was also supported by the project financed within the joint program of the Ministry of Agriculture, Forestry and Food and Slovenian Research Agency (V4-2017).
Disclosure statement
No potential conflict of interest was reported by the author(s).
Additional information
Funding
References
- Alao, P., Visnapuu, K., Kallakas, H., Poltimäe, T. and Kers, J. (2020) Natural weathering of bio-based facade materials. Forests, 11(6), 1–12. doi:10.3390/f11060642
- ARSO (2020) Agencija Republike Slovenije za okolje / Slovenian environmental agency. http://meteo.arso.gov.si/met/sl/archive/.
- Baysal, E., Degirmentepe, S. and Simsek, H. (2014) Some surface properties of thermally modified Scots pine after artificial weathering. Maderas: Ciencia y Tecnologia, 16(3), 355–364. doi:10.4067/S0718-221X2014005000028
- Belt, T., Hänninen, T. and Rautkari, L. (2017) Antioxidant activity of Scots pine heartwood and knot extractives and implications for resistance to brown rot. Holzforschung, 71(6), 527–534. doi:10.1515/hf-2016-0232
- Bornemann, T., Brischke, C. and Alfredsen, G. (2014) Decay of wooden commodities – moisture risk analysis, service life prediction and performance assessment in the field. Wood Material Science and Engineering, 9(3), 144–155. doi:10.1080/17480272.2014.904431
- Brischke, C., Hesse, C., Meyer-Veltrup, L. and Humar, M. (2017) Studies on the material resistance and moisture dynamics of common juniper, English yew, Black cherry, and Rowan. Wood Material Science and Engineering, 13(4), 222–230. doi:10.1080/17480272.2017.1356371
- Brischke, C., Meyer, L., Alfredsen, G., Humar, M., Francis, L., Flæte, P. O. and Larsson-Brelid, P. (2013) Prirodna trajnost drva izloženoga iznad zemlje – pregled istraživanja. Drvna Industrija, 64(2), 113–129. doi:10.5552/drind.2013.1221
- Brischke, C., Welzbacher, C. R., Gellerich, A., Bollmus, S., Humar, M., Plaschkies, K., Scheiding, W., Alfredsen, G., Van Acker, J. and De Windt, I. (2014) Wood natural durability testing under laboratory conditions: Results from a round-robin test. European Journal of Wood and Wood Products, 72(1). doi:10.1007/s00107-013-0764-6
- CEN (2004) European Standard EN 11341 – Paints and varnishes – Artificial weathering and exposure to artificial radiation – Exposure to filtered xenon-arc radiation.
- CEN (2005). European Standard CEN/TS 15083-1 – Durability of wood and wood-based products. Determination of the natural durability of solid wood against food-destroying fungi, test methods. Basidiomycetes.
- CEN (2006) European standard EN 113 Wood preservatives: Test method for determining the protective effectiveness against wood destroying basidiomycetes: Determination of the toxic values. European Committee for Standardization.
- CEN (2013a) European Standard 1609 – Thermal insulating products for building applications – Determination of short term water absorption by partial immersion.
- CEN (2013b) European Standard EN 335, Durability of wood and wood-based products – Use classes: Definitions, application to solid wood and wood-based products (Brussels: European Committee for Standardization).
- CEN (2015) European Standard EN 252 – Field test method for determining the relative protective effectiveness of a wood preservative in ground contact.
- CEN (2016) European Standard EN 350 – Durability of wood and wood-based products. Testing and classification of the durability to biological agents of wood and wood-based materials.
- Gasparri, E., Lucchini, A., Mantegazza, G. and Mazzucchelli, E. S. (2015) Construction management for tall CLT buildings: From partial to total prefabrication of façade elements. Wood Material Science and Engineering, 10(3), 256–275. doi:10.1080/17480272.2015.1075589
- Hazneza, S. and Evans, P. D. (2016) End-grain erosion of Douglas fir wood during natural weathering. International Wood Products Journal, 7(1), 3–11. doi:10.1080/20426445.2015.1104076
- Hill, C. A. S. (2011) Wood modification: An update. BioResources, 6(2), 918–919. doi:10.15376/biores.6.2.918-919
- Humar, M., Kržišnik, D., Lesar, B. and Brischke, C. (2019) The performance of wood decking after five years of exposure: Verification of the combined effect of wetting ability and durability. Forests, 10(10). doi:10.3390/f10100903
- Humar, M. and Lesar, B. (2008) Fungicidal properties of individual components of copper-ethanolamine-based wood preservatives. International Biodeterioration and Biodegradation, 62(1), 46–50. doi:10.1016/j.ibiod.2007.06.017
- Humar, M., Peek, R. D. and Jermer, J. (2006) Regulations in the European Union with emphasis on Germany, Sweden and Slovenia. In T. G. Townsend, and H. Solo-Gabriele (eds.) Environmental Impacts of Treated Wood 1st Edition (Boca Raton: CRC Press), p. 520.
- Isaksson, T., Brischke, C. and Thelandersson, S. (2013) Development of decay performance models for outdoor timber structures. Materials and Structures/Materiaux et Constructions, 46(7), 1209–1225. doi:10.1617/s11527-012-9965-4
- Isaksson, T., Thelandersson, S., Jermer, J. and Brischke, C. (2014) Beständighet för utomhusträ ovan mark. In Guide för utformning och materialval (Rapport TVBK–3066).
- Johnston, S. R., Boddy, L. and Weightman, A. J. (2016) Bacteria in decomposing wood and their interactions with wood-decay fungi. FEMS Microbiology Ecology, 92(11), 1–12. doi:10.1093/femsec/fiw179
- Jung, S. J., Kim, N. K., Lee, D. H., Hong, S. I. and Lee, J. K. (2018) Screening and evaluation of streptomyces species as a potential biocontrol agent against a wood decay fungus, Gloeophyllum trabeum. Mycobiology, 46(2), 138–146. doi:10.1080/12298093.2018.1468056
- Kirker, G. T., Blodgett, A. B., Arango, R. A., Lebow, P. K. and Clausen, C. A. (2013) The role of extractives in naturally durable wood species. International Biodeterioration and Biodegradation, 82, 53–58. doi:10.1016/j.ibiod.2013.03.007
- Kropat, M., Hubbe, A. M. and Laleicke, F. (2020) Natural, accelerated, and simulated weathering of wood: A review. BioResources, 15(4), 9998–1062.
- Kržišnik, D., Lesar, B., Thaler, N. and Humar, M. (2018a) Influence of natural and artificial weathering on the colour change of different wood and wood-based materials. Forests, 9(8), 1–22. doi:10.3390/f9080488
- Kržišnik, D., Lesar, B., Thaler, N. and Humar, M. (2018b) Performance of bark beetle damaged Norway spruce wood against water and fungal decay. BioResources, 13(2). doi:10.15376/biores.13.2.3473-3486
- Lähtinen, K., Harju, C. and Toppinen, A. (2019) Consumers’ perceptions on the properties of wood affecting their willingness to live in and prejudices against houses made of timber. Wood Material Science and Engineering, 14(5), 325–331. doi:10.1080/17480272.2019.1615548
- Lie, S. K., Thiis, T. K., Vestøl, G. I., Høibø, O. and Gobakken, L. R. (2019) Can existing mould growth models be used to predict mould growth on wooden claddings exposed to transient wetting? Building and Environment, 152, 192–203. doi:10.1016/j.buildenv.2019.01.056
- Malá, D., Sedliačiková, M., Drábek, J., Jelačić, D. and Minárová, M. (2019) Consumer perception of environmentally sustainable products of Slovak wood processing enterprises. Drvna Industrija, 70(4), 407–418. doi:10.5552/drvind.2019.1922
- Meyer-Veltrup, L., Brischke, C., Alfredsen, G., Humar, M., Flæte, P. O., Isaksson, T., Brelid, P. L., Westin, M. and Jermer, J. (2017) The combined effect of wetting ability and durability on outdoor performance of wood: Development and verification of a new prediction approach. Wood Science and Technology, 51(3), 615–637. doi:10.1007/s00226-017-0893-x
- Militz, H. (2020) Wood modification research in Europe. Holzforschung, 74(4), 333. doi:10.1515/hf-2020-0050
- Morris, P. I. and Stirling, R. (2012) Western red cedar extractives associated with durability in ground contact. Wood Science and Technology, 46(5), 991–1002. doi:10.1007/s00226-011-0459-2
- Osawa, T., Maeda, K., Tsunetsugu, Y., Shida, S. and Shida, S. (2019) Influence of surface checks on wood moisture content during wetting and re-drying. European Journal of Wood and Wood Products, 77(4), 681–689. doi:10.1007/s00107-019-01423-w
- Petrič, M. (2013) Surface modification of wood: A critical review. Reviews of Adhesion and Adhesives, 1(2), 216–247. doi:10.7569/RAA.2013.097308
- Preston, A. F. (2000) Wood preservation. Forest Products Journal 2, 50(9), 1–12.
- Raspor, P., Smole Mozina, S., Podjavorsek, J., Pohleven, F., Gogala, N., Nekrep, F. V., Rogelj, I. and Hacin, J. (1995) ZIM : Culture Collection of Industrial Microorganisms (ZIM) Ljubljana (Ljubljana: University of Ljubljana).
- Rep, G., Pohleven, F. and Kosmerl, S. (2012) Development of the industrial kiln for thermal wood modification by a procedure with an initial vacuum and commercialisation of modified Silvapro wood. In M. H. Jones, M. P. D. Jones, H. Militz, M. Petrič, and F. Pohleven (eds.) Proceedings of the 6th European Conference on Wood Modification (Ljubljana: University of Ljubljana), pp. 11–17.
- Schmidt, O. (2006) Wood and Tree Fungi: Biology, Damage, Protection, and use (Berlin Heidelberg: Springer-Verlag). https://doi.org/10.1007/3-540-32139-X.
- Schultz, T. P. and Nicholas, D. D. (2000) Naturally durable heartwood: Evidence for a proposed dual defensive function of the extractives. Phytochemistry, 54(1), 47–52. doi:10.1016/S0031-9422(99)00622-6
- Strobel, K., Nyrud, A. Q. and Bysheim, K. (2017) Interior wood use: Linking user perceptions to physical properties. Scandinavian Journal of Forest Research, 32(8), 798–806. doi:10.1080/02827581.2017.1287299
- Thaler, N., Brischke, C., Žlindra, D., Vek, V. and Humar, M. (2014) Changes in mechanical and chemical properties of wood exposed outdoors. Proceedings IRG Annual Meeting, IRG/WP(14–20550), 12.
- Thaler, N., Žlahtič, M. and Humar, M. (2014) Performance of recent and old sweet chestnut (Castanea sativa) wood. International Biodeterioration and Biodegradation, 94, 141–145. doi:10.1016/j.ibiod.2014.06.016
- Van Acker, J., Van den Bulcke, J., De Windt, I., Colpaert, S. and Li, W. (2015) Moisture dynamics of modified wood and the relevance towards decay resistance. 8th European Conference on Wood Modification (ECWM8), 44–55.
- Vek, V., Poljanšek, I. and Oven, P. (2019) Efficiency of three conventional methods for extraction of dihydrorobinetin and robinetin from wood of black locust. European Journal of Wood and Wood Products, 77(5), 891–901. doi:10.1007/s00107-019-01430-x
- Vek, V., Vivod, B., Poljanšek, I. and Oven, P. (2019) Vsebnost ekstraktivov v skorji in lesu robinije (Robinia pseudoacacia L.). Acta Silvae et Ligni, 119, 13–25. doi:10.20315/asetl.119.2
- Venäläinen, M., Harju, A. M., Saranpää, P., Kainulainen, P., Tiitta, M. and Velling, P. (2004) The concentration of phenolics in brown-rot decay resistant and susceptible Scots pine heartwood. Wood Science and Technology, 38(2), 109–118. doi:10.1007/s00226-004-0226-8
- Žlahtič, M. and Humar, M. (2016a) Influence of artificial and natural weathering on the hydrophobicity and surface properties of wood. BioResources, 11(2), 4964–4989. doi:10.15376/biores.11.2.4964-4989
- Žlahtič, M. and Humar, M. (2016b) Influence of artificial and natural weathering on the moisture dynamic of wood. BioResources, 11(1), 4964–4989. doi:10.15376/biores.12.1.117-142
- Žlahtič-Zupanc, M., Lesar, B. and Humar, M. (2018) Changes in moisture performance of wood after weathering. Construction and Building Materials, 193, 529–538. doi:10.1016/j.conbuildmat.2018.10.196
- Žlahtič, M., Thaler, N. and Humar, M. (2015) Upijanje vode toplinski modificirane norveške smreke. Drvna Industrija, 66(4), 273–279. doi:10.5552/drind.2015.1421