ABSTRACT
Wood is a traditional roofing material in Europe and other parts of the world. In the seventeenth century, wooden roofing was generally used on more important buildings, but today it is mainly used on huts and houses in the Alpine regions. As wooden roofing is expensive, we investigated the possibility of extending the service life of the roofing through material selection and details. The roof of the Golobar cable yarding was covered with shingles made of spruce (Picea abies) and larch (Larix decidua). Part of the spruce roofing was thermally modified and/or treated with a water-wax emulsion. On the underside, we cut grooves in selected roof shingles to increase the specific surface of the wood and to accelerate drying after rainfall. In addition, wood moisture monitoring sensors were installed on the roof shingles. Based on three years of the wood moisture content measurements, it can be concluded that among shingles made of untreated spruce wood, the highest moisture content was recorded for shingles with a rectangular cross-section without additional grooves. The additional grooves positively affected the moisture performance of wood. Treating the wood with Silvacera wax had an even more pronounced effect on the moisture content of the shingles than the grooves.
1. Introduction
Webster’s Dictionary defines a roof as the covering of a building (Anonymous Citation2023). In the past, wooden roofing was one of the most common roofing materials in present-day Slovenia, along with thatch roofing (Šarf Citation1976). It should be considered that the forest cover in Europe and also in Slovenia was much lower in the past than it is today, and wood was, therefore, more valuable (Kaplan et al. Citation2009). It is therefore not surprising that the farmhouses in the seventeenth-century depictions of Valvasor are mostly thatched. Only the more important buildings, such as castles, palaces and churches, were roofed with wood (Valvasor et al. Citation1969). Archaeological finds confirm that wooden shingles were already used in Hallstatt in Upper Austria in the Bronze Age (Kain et al. Citation2020). Wood-based roof systems were used throughout the Alpine region (Palanti et al. Citation2014, Kain et al. Citation2020) and in other European regions from the Black Sea to Karelia (Bucşa and Bucşa Citation2010, Kozlov and Kisternaya Citation2014, Kurek Citation2019). In the twentieth century, wooden roofs were often seen as a sign of poverty and a backward economy and were quickly replaced by brick roofs and later by cement-asbestos roofs (Šarf Citation1976). Brick roofs were introduced in Slovenia on farm buildings only at the end of the nineteenth century. However, wooden roofing proved to be more suitable than thatched roofing for the harsh alpine climate. Thus, wooden roofing remained in Slovenia, mainly on cottages in the Alps and on farms in southern Carinthia, Trenta, in the Gornjesavska valley and on mountain Velika Planina (Cevc et al. Citation1993). The best-known wooden roofing material is split wooden shingles. Shingles were made from the heartwood of spruce (Picea abies), sometimes also from the heartwood of larch (Larix decidua). In some of the southern Slovenian regions (Notranjska and Kočevsko), however, fir wood (Abies alba) was also used for this purpose, predominately due to its availability (Šarf Citation1976). The wood used for shingles is of the highest quality and grows slowly and uniformly. Although shingles today are mainly made from radially split shingles, tangentially split shingles were also used in the past. In addition, to split shingles, sawn shingles or boards are also used for roofing. These are usually longer and may be made of lower-quality wood (Jerin and Povse Citation2017). Traditionally, sawn boards were used for roofing, mainly in valleys where water sources were available to power sawmills. Sawn roof boards were cheaper than split ones. In addition, lower-quality timber could be used for sawing, and better-quality timber was sold. In the mountains, where there were no water sources for power, sawing and transport were expensive and very difficult; split boards were used for roofing. In this way, the farmers and forest owners achieved the best economic efficiency.
The field of wooden roofing is covered by the standard DIN 68119 (DIN Citation2019). According to this standard, shingles can be made from the following species of wood: European beech (Fagus sylvatica), pedunculate oak and sessile oak (Quercus robur and Q. petrea), Norway spruce (Picea abies), silver fir (Abies alba), European and Siberian larch (Larix decidua and Larix sibirica), Scots pine (Pinus sylvestris), yellow cedar (Xanthocyparis nootkatensis), western redcedar (Thuja plicata, Thuja occidentalis) and others. Most of the listed wood species have also been used in Romania and other European countries for cladding historical objects (Bucşa and Bucşa Citation2010). However, in northern regions such as Karelia, where the climate is less optimal for fungal decay, shingles are also made from aspen (Populus tremula) (Kozlov and Kisternaya Citation2014). In the past, shingles could be treated with wood preservatives (Plackett et al. Citation1984). Nowadays, however, the use of biocides is limited due to environmental awareness, rainwater harvesting, and legislative requirements. Therefore, naturally durable species or modified wood are preferred. The length of the cover boards should be between 15 and 80 cm, depending on the type of cover. Since spruce wood splits more easily than larch wood (Wagenführ Citation2014), spruce shingles are generally longer than larch shingles. Today, spruce shingles are generally between 80 and 100 cm long, while larch shingles are generally no longer than 50 cm. The thickness of the shingles varies between 5 and 8 mm (Kranjska Gora) and 12 mm (Velika Planina) (Koželj Citation2020), while sawn planks are thicker (20–25 mm). The tradition of wooden roofing has never completely stopped and in recent years, along with a renaissance of ecological building materials, wooden shingles have been increasingly used again for roofs (Kain et al. Citation2020) as well as for claddings (Rüther and Time Citation2015).
In Slovenia, wooden roofing has a traditionally strong presence in Trenta valley in Slovenia. Residential, commercial and agricultural buildings are covered with wooden roofs. Among others things, the lower station of the Golobar cable yarding is also covered with wood. In Slovenia, timber harvesting by cable car has a long tradition. The Pantz cable yarding, located on the road from Bled to Bohinj, is the oldest still existing forestry cableway in Europe. In its 82 years of operation, it has transported over half a million cubic metres of wood and charcoal (Brate Citation1988). The Golobar cable yarding is best known which connected the 1250-metre-high Golobar Mountain with the Soča Valley (Kozorog Citation1992). In the period from 1930 to 1943, 8 circular cable yarding were in operation in the region, some for only a few years, others including the Golobar cable yarding. The tradition of building cable yarding in the Soča Valley dates back to military cableways during World War I. The Golobar cable yarding was one of the last functioning cableways in the country and was in regular operation until 1968. The lower station is one of the few surviving structures of its kind in the country, while the upper station and supporting pillars have collapsed. In 1989, the Golobar cableway was declared as a technical monument and protected. The Golobar cable yarding is located in a gorge above the Soča River and is exposed to harsh climatic conditions with approximately 2000mm of annual precipitation (ARSO Citation2023). This object provides an excellent opportunity to monitor the moisture content and performance of the wood.
The main cause of the failure of wooden roofs is wood decay, which develops on moist wood (Marais et al. Citation2022). Monitoring the moisture content of materials and products therefore allows us to assess the threat at a given micro-location, monitor the behaviour of materials and predict the service life of wood and wooden objects (Brischke and Thelandersson Citation2014). In addition to susceptible wood or comparable lignocellulosic materials, the following factors are required for wood-decaying fungi to grow: oxygen, a slightly acidic environment (pH between 4.0 and 5.5), temperature (between 3 and 40°C) and suitable wood moisture content (Eaton and Hale Citation1993, Schmidt Citation2006, Zabel and Morrell Citation2020). Fungi can decompose wood above a specific moisture content, but if the wood is too dry or too moist, the biochemical processes of wood decomposition do not occur. Various studies on wood-degrading fungi have shown that the minimum moisture content of wood suitable for decomposition depends on the fungal species and the wood and ranges between 25% and 30% (fibre saturation) (Schmidt Citation2006, Brischke and Alfredsen Citation2020). Many authors (Stienen et al. Citation2014, Meyer et al. Citation2016, Zabel and Morrell Citation2020) have shown that fungi can colonise and subsequently decompose wood even at moisture contents below fibre saturation if sufficient water is available. In extreme cases, it has been shown that mycelium can grow on wood with a moisture content of 17.4%. The optimal temperature for fungal decay is between 20 and 30°C (Teodorescu et al. Citation2017). However, fungi can also decompose wood in a broader range between 3 and 40°C. At lower temperatures, water freezes, while at higher temperatures, protein denaturation occurs sooner or later, and wood degradation stops or slows down considerably (Stienen et al. Citation2014). Temperature and humidity can be referred to as material climate (Isaksson and Thelandersson Citation2013). This should be considered the most important parameter in predicting the service life of wood (Brischke et al. Citation2008, Pouska et al. Citation2016, Kržišnik et al. Citation2018). Therefore, it is vital to monitor the moisture content of wood and link it with the service life (Fredriksson et al. Citation2016).
Enabling moisture exclusion is the principle of protection by design. If wood is kept dry, decay cannot occur. The principle of protection by design is based on the umbrella and boot approach. An umbrella (roof) prevents the wood from being wetted by precipitations, while boots (steel anchors) prevent capillary water absorption from the ground (MacKenzie Citation2007). However, protecting the roof seems to be more challenging, as the roof material acts like an umbrella for the whole structure. The idea of protection by design of the wooden roof is to increase the surface-to-volume ratio of the elements. This speeds up drying and limits water accumulation between the roof layers (Jones and Brischke Citation2017).
This paper describes the moisture performance of the wooden roof shingles and some structural elements on the partially renovated Golobar cable yarding. The renovated roof is fitted with wooden planks made of different wood-based materials (thermally modified wood, wax-treated wood, Norway spruce and European larch wood). In addition, the surface of the respective elements has been prepared differently to increase the surface volume ratio. The data from the moisture monitoring data will form the basis for the development of an optimal timber roof solution in the respective Soča Valley. Based on the moisture dynamics of the wood and literature data on the durability of wood, it is possible to estimate the service life and, thus the maintenance costs of the individual solutions.
2. Materials and methods
2.1. Materials used for roofing
Ten types of wooden shingles (boards) were used for the roof of the Golobar cable yarding, as shown in and and and . The thickness of the shingles was 22 mm, following the local tradition. The roof shingles were mainly (9 out of 10 materials) made of Norway spruce (Picea abies), with a smaller proportion of European larch (Larix decidua) as a reference. Respective wood species were chosen, as they are the key wood species in the area and are traditionally used for roofing in the respective region. No suitable hardwoods are available in the Trenta valley; thus, hardwoods were not utilised. Half of the spruce roof shingles were thermally modified using the Silvapro process at Silvaprodukt at 230°C (Rep et al. Citation2012). A quarter of the modified and non-modified roof shingles were surface treated with a Silvacera wax-water emulsion (Humar et al. Citation2017). The roof shingles had different profiles. The shingles were profiled from the lower part, as shown in . This increased the specific surface area, resulting in faster drying and lower internal stresses (Heshmati et al. Citation2018). All combinations can be seen in . At least 20 shingles are produced from each shingle variant on the roof of the Golobar yarding ().
Figure 2. Golobar cable yarding. The roof covered with wooden tiles made of various wood-based materials is clearly seen.
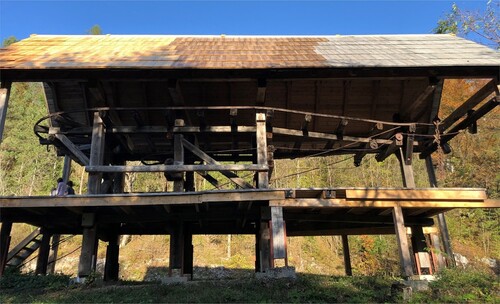
Table 1. Materials and machining used in the production of the planks used for the Golobar cable yarding.
Table 2. Aggregated data from wood moisture monitoring at the Golobar cable yarding. Each value represents 2214 measurements.
2.2. Monitoring
The climatic conditions, temperature (T) and relative humidity (RH) are monitored on the Golobar yarding (). In the covered part of the Golobar yarding, away from any sources of wetting, we installed devices for monitoring relative humidity and temperature from Scanntronik (Germany). A thermo-hygro sensor was installed on a Thermofox data logger (Scanntronik, Germany), which operates in the measuring range from −10°C to +50°C and RH between 0 and 99% RH (repeatability of 1.8% RH and 0.3°C, respectively). Data (measured value, minimum and maximum) were recorded at hourly intervals between 17 October 2019 and 28 October 2022. 80,000 values (temperature and RH) were recorded during this period. Similar measurements are also carried out at other sites (Kržišnik et al. Citation2018, Humar et al. Citation2019). Precipitation data were obtained from the archive of the Slovenian Environment Agency. The data refer to the Trenta rainfall station nearby, while the pH values and concentration of NO3 ad SO4 were obtained from the rainfall station that is located in Rateče 25 km from the Golobar cable yard (ARSO Citation2023). Based on the climate data Scheffer climate index was calculated (Scheffer Citation1971).
Moisture sensors were installed in the roof and structural elements to monitor the moisture content of the wood. The moisture content of the wood is determined using the electrical resistance method (Stamm Citation1927). For this purpose, we use insulated steel electrodes that measure the electrical resistance in the middle of the samples. Two stainless steel screws with a diameter of 3.9 mm (Bauhaus Profi Depot, Germany) were screwed into the middle of the tangential face of the samples at a distance of 32 mm and pre-insulated with a universal shrink polymer – DERAY®-H. The electrodes were fixed to the screws and connected to the measuring instruments. The moisture content was determined in the middle, as most fungi usually develop in the middle of the samples and this is therefore the most representative location. The electrical resistance was measured every 12 h with the Gigamodul (Scanntronik, Germany) and recorded with Thermofox data loggers (Scanntronik, Germany). Parallel to the moisture content, the surface temperature of the samples was also recorded. The moisture content of the wood was calculated from the electrical resistance and temperature data (Brischke and Lampen Citation2014). The moisture measurements have already been described in detail (Kržišnik et al. Citation2018, Humar et al. Citation2020).
3. Results and discussion
Along with the moisture content of the wood, the temperature is one of the decisive factors influencing fungal decay. Both too-high and too-low temperatures slow down or even stop fungal decomposition. The minimum temperature required for fungal decomposition is around 3°C (Schmidt Citation2006). However, when analysing temperatures, it is important to remember that temperature can vary considerably within site, as monitoring of the hunting cabin on Spitsbergen has shown (Gobakken et al. Citation2008). When analysing the climatic conditions, it is important to remember that the measurements were taken over three years. The temperature varied between −9.0°C (12 January 2021 at 7.00 am) and 38.2°C (22 July 2022 at 3.00 pm). The average temperature was 10.6°C. The temperature was above the threshold required for fungal decomposition for 78% of the period (A). Lower temperatures can slow down the wood-degrading fungi but do not kill them (Unger et al. Citation2001). In addition to temperature, the development of fungi (especially moulds and tertiary moulds) is also influenced by relative humidity (RH). If the RH is high, the wood absorbs water vapour from the air, and if the RH is low, the wood dries out. If the dew point temperature is close to the outside temperature, condensation can occur, which is reflected in a rapid increase in the surface moisture of the wood. Mould on the wood surface occurs when the RH exceeds 80% for a prolonged period of time, while prolonged, cumulative periods of RH above 90% are required for degradation to develop. The RH on the Golobar cableway varied from 9.9% (9 April 2020) to 100% (rainy periods in November and December) (A). The spring period is characterised by weeks of very dry air in the respective regions (Kržišnik et al. Citation2018), particularly evident in the low values in March and April. The average RH during the measurement period was 78.9%.
Figure 3. Distribution of (A) relative humidity (RH) and temperature, (B) precipitation and (C) wood moisture content during the monitoring period between 17 October 2019 and 28 October 2023 at Golobar cable yarding. The wood MC monitoring locations are listed in .
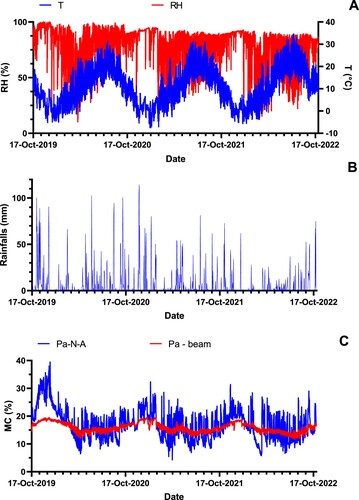
The site in question, the Trenta Valley, received 2396 mm of precipitation in 2020 and 1894 mm of precipitation in 2021. There were five days with more than 100 mm of precipitation per day during the monitoring period, predominately in late autumn. Monitoring lasted 1110 days, of which 431 were rainy days with at least 0.1 mm of precipitation (ARSO Citation2023) (B). This figure shows that the Trenta area is a very humid site, which is also reflected in the moisture content of the wood. On the other hand, the spring period was very dry, which is traditionally typical for March. Chemical analysis of rain was performed on the rain from nearby rainfall station Rateče. As Golobar cable yarding and Rateče rainfall station are located within the area of Julian Alps in the protected area in the region without heavy industry, we presume that the quality of the rain in Rateče and Trenta is comparable. The average pH value of the rain in 2021 was 5.62. Value 5.6 is considered as the threshold for acid rain. The pH of the rains was above 5.6 in the months between February and September 2021. The lowest pH determined was in January 2021 (5.03). High pH is in line with rather low average concentrations of NO3 (0.034 mg/L) and SO4 (0.039 mg/L) (Anonymous Citation2022). As the pH of the rain is within the range of the pH of wood and is within the limits of the pH optimal for fungal decay, we presume that the pH of the rain does not influence fungal decay development (Schmidt Citation2006). In addition, the high buffering capacity of wood, which reduces the importance of rain pH, should be considered as well. Based on the climatological data, the Scheffer climate index (SCI) was calculated only for the monitoring period of three years. The parameter “climate index” was proposed to estimate decay risk at different geographical locations (Scheffer Citation1971, Humar et al. Citation2021). The average SCI index was 65, indicating a relatively high estimated risk of decay compared to other European sites. SCI index in some tropical sites could be much higher.
The most important result of the study was the monitoring of wood moisture in the Golobar yarding. Through moisture monitoring service life of wood can be predicted much faster, than through visual decay assessment. C shows two representative examples of wood moisture trends, a wooden roof shingle (Pa-N-A) exposed to all weather conditions and an old beam not exposed to precipitation. In both cases, the measurement was carried out on Norway spruce wood. Precipitation events primarily influence the moisture content of wooden roof shingles. Therefore, the moisture content of the roof shingle was higher than that of the beam for most of the monitoring period, except for dry and sunny days when direct sunlight led to the faster drying of the shingles. The roof shingles correspond to use class 3.2, while the beams belong to use class 2. This is reflected in the moisture content of the wood (). The moisture content of the construction corresponded to the ten-day average RH. Previous studies have demonstrated that the moisture content of wood is not closely related to the daily RH average (Humar et al. Citation2020). Adsorption, desorption and diffusion are slow and related to long-term RH averages. Relatively high daily oscillations are predominantly observed. The most considerable daily oscillation was recorded on 28 February 2020, when the difference between the highest (89.9%) and lowest RH (19.2%) was 70.7%. The moisture content of the wood in use class 2 is much more influenced by the moisture content of the previous ten days than by the current RH. The relationship between the wood moisture content and the 10-day average RH can be resolved in (r2 = 0.46; P < 0.0001). This result confirms that wood in use class 2 is mainly affected by relative humidity and temperature. The correlation could be even better if there were no offset of the RH sensor, as resolved in A. This offset is probably due to dust deposited on the sensor. The moisture content of the beam was thus relatively constant and ranged between 12.5% and 19.3%, which is considerably less compared to the more exposed shingle, where MC ranged between 6.3% and 36.0%. On the other hand, the moisture content of wood shingles is influenced by RH and reflects precipitation. On rainy days, the MC of wooden shingles is higher than the MC of beams sheltered from rain, while on sunny days, the wood dries out faster, which is reflected in a lower MC than for less exposed wood ().
Figure 4. Correlation between ten-day average relative humidity (RH), rainfall and moisture content of wood during the monitoring period between 17 October 2019 and 28 October 2023 at the Golobar cable yarding. Wood MC monitoring sites are resolved in .
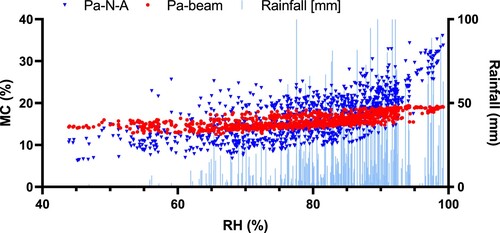
The data of the moisture measurement of wood are summarised in . When analysing the data, it should be taken into account that the Norway spruce shingles were technically dry before installation. Since the resistance measurement of moisture content is less accurate at higher moisture contents above fibre saturation, it is better to use medians to compare the data (Kržišnik et al. Citation2018, Humar et al. Citation2020). Among all shingles made of untreated spruce wood, the highest moisture content was recorded for Pa-N-A shingle with a rectangular cross-section of type A without additional grooves (). The additional grooves positively affected the moisture content of the wood. The grooves contributed to the shingles having a larger surface area, which led to faster drying. Type C roof shingles performed significantly better than type B and type A roof shingles. The median moisture content of type A spruce tiles is 16.8% (Pa-N-A), which is the same as that of type B (Pa-N-B), while type C performs best at 16.3% (Pa-N–C) (). There are also clearer differences in the proportion of measurements where the moisture content of the wood is above 25%. This moisture content is considered the limit above which the probability of fungal decay increases significantly (Brischke and Alfredsen Citation2020). According to recent measurements, the first signs of decay in spruce wood occur when the number of days with a moisture content above 25% exceeds 325 days (Isaksson et al. Citation2013, Meyer-Veltrup et al. Citation2017). For shingles without grooves ((Pa-N-A) 7% of such days were recorded during the monitoring period, while for spruce shingles with C-grooves only 5% of such values were recorded (). As can be seen in , the most notable difference in moisture dynamics is found in the dry periods. Wooden shingles without grooves reach a comparable moisture content when it rains, but drying is faster and more pronounced when the grooves are present.
Figure 5. Influence of shingle design on the moisture content of Norway spruce wood shingle during the monitoring period between 17 October 2019 and 28 October 2023 at Golobar cable yarding. Wood MC monitoring sites are resolved in .
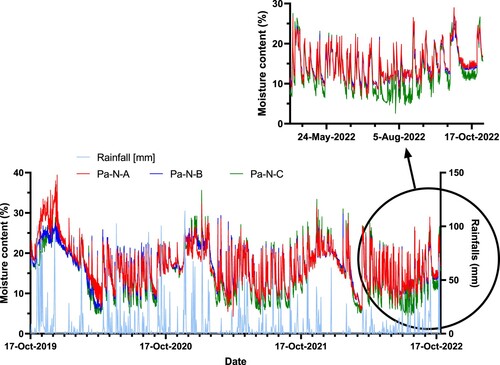
Treating the wood with Silvacera wax had an even more pronounced effect on the moisture content of the shingles than the grooves. The wax forms a water-repellent film on the surface, allowing water vapour to diffuse and dry the wood (Lesar et al. Citation2011, Lesar and Humar Citation2011). The combination of waxes and profiles on the roof shingles had a synergistic effect. For example, the mean moisture content of the wax-treated B-profile spruce roof shingle was consistently below the 25% MC threshold (). Furthermore, despite intense rainfall during the measurement period, the moisture content never exceeded 20% MC ().
On the other hand, thermally modified wood is characterised by increased permeability due to the modification. As a result, it often absorbs more water during rainfall than unmodified wood. However, it must be considered that it also dries out more quickly. Therefore, the number of days on which the moisture content of thermally modified wood exceeded the specified limits was comparable to that of spruce wood. The median of the thermally modified wood (10.8%; Pa-TM-A) was lower than that of the unmodified wood (16.8%; Pa-N-A) (). The sorption properties of the modified wood were the main reason for the low MC. During dry periods the equilibrium moisture content of modified wood is significantly lower than that of unmodified spruce under the same climatic conditions (Esteves and Pereira Citation2009). As with unmodified spruce wood, grooving and wax treatment also positively affected the moisture content of modified wood. The wax treatment slowed down the wetting of the thermally modified wood, and the grooves increased the specific surface area of the wood, resulting in lower moisture content than modified spruce wood. Surprisingly, thermally modified wood did not show high moisture performance. The excellent moisture performance of freshly thermally modified wood has been reported many times (Olek et al. Citation2013, Hill et al. Citation2021). However, as shown in the data presented in , exposure touse class 3.2 conditions apparently resulted in increased water uptake. The decrease in moisture performance can be attributed to microcrack formation, bacterial degradation of the pit membranes and blue staining (Žlahtič-Zupanc et al. Citation2018, Keržič et al. Citation2021).
The moisture content of Norway spruce and thermally modified spruce were compared to see the difference in the moisture content of the wood between dry and wet periods (). The distinction between wet and dry days was made based on rainfall events. Days with rainfall and a subsequent day after the rain event were considered wet days. As can be seen from , the moisture content of thermally modified shingles is lower on both wet and dry days than the MC of untreated spruce wood, but the deviation for thermally modified wood is much higher. The analysis of moisture dynamics shows that thermally modified wood absorbs more water during rain events but releases it more quickly.
Figure 6. Moisture content of wooden shingles in wet and dry periods during the monitoring period between 17 October 2019 and 28 October 2023 at the Golobar cable yarding. Wood MC monitoring locations are resolved in .
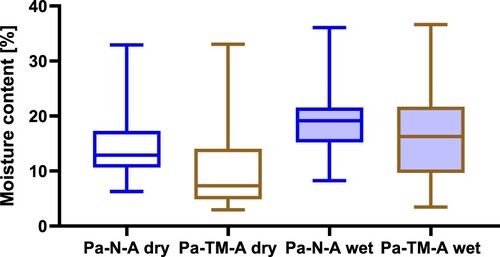
The question might arise of how the presence of the shingles’ profiles influences the roofing elements’ mechanical properties. It should be considered that the shingles are not exposed to high loads. In addition, shingles are rather thick. In some of the locations in Slovenia and the Alps, even thinner shingles are used, and mechanical failure was newer reported as the prime reason for failure. Thus we presume that the presence of the profiles will not reduce the technical service life. Despite some heavy storms in the area, all shingles remain in place.
For comparison, the moisture content on some elements of the Golobar cable yard structure was also analysed. These measurements allow us to assess structural risk and predict future decay. The first sensor was installed on the batten under the roof covering. This batten is not directly exposed to precipitation but is in contact with potentially wet shingles. The moisture content of this batten has never exceeded 25% MC, indicating that the roof shingles are doing their job, preventing the rain from entering the building. However, the batten has an MC above 20% for 8% of the monitoring time. This value is a result of the high humidity under the shingles. Part of the reason for the high moisture content of these battens could be that these battens were not dry when installed.
In addition, other older parts of the construction were monitored as well. The beam and the upper part of the column are in use class 2 according to EN 335 (CEN, 2013). Respective use class is usually not threatened by wood decay fungi. This is reflected in the moisture content of the wood, which never exceeds 20%. The low moisture content shows that the Golobar yarding does not become moisturised and that rain does not soak vital parts of the structure, even when windy with wind-driven precipitation. The moisture content of the column near the ground was significantly higher. This location also recorded the highest humidity (104%) throughout the monitoring period. The average value at this site was 19.0%, and the median 15.7%. The moisture content of the wood was above 25% for the quarter of the monitoring period (), as precipitation runs off the roof near the column, so this part of the cableway is highly exposed to moisture due to the reflection of water.
It should be considered that the moisture performance of wood changes during weathering. Exposure to sunlight, fluctuating temperature and rain leads to superficial deterioration and reduced surface hydrophobicity. Therefore it is important to perform long-term moisture monitoring, which can serve as input for future moisture modelling (Niklewski et al. Citation2022).
4. Conclusions
The Golobar yarding is an important wooden monument of Slovenia’s technical heritage. The building is made entirely of wood and is located in a humid environment above the Soča River in an area of high rainfall. This is reflected in the high potential for wood degradation. Therefore, it is necessary to pay more attention to the protection by-design details to protect the construction.
The building is covered with wooden shingles. Traditionally, wooden shingles are used for roofing in Trenta. From three years of moisture content measurements, it can be concluded that among shingles made of untreated spruce wood, the highest moisture content was recorded for shingles with a rectangular cross-section without additional grooves. The additional grooves positively affected the moisture content of the wood. The grooves contributed to the shingles having a larger surface area, which led to faster drying. Treating the wood with Silvacera wax had an even more pronounced effect on the moisture content of the shingles than the grooves. The wax forms a water-repellent film on the surface, allowing water vapour to diffuse and dry the wood. The positive effect of wax treatment and grooves was determined at thermally modified wood as well. Based on the moisture monitoring, it can be presumed that the service life of wax-treated spruce wood shingles with grooves is up to three times longer than of untreated shingles without grooves.
We will continue to monitor the facility. We expect to come to more reliable conclusions if we monitor the moisture content of the wood over a more extended period of time. Based on the respective monitoring, the optimal solution will be formulated and guidelines for the local populations will be prepared in order to offer a sustainable and economically feasible solution.
The experiment will be validated on additional objects in the same valley and on the experimental objects on the field test site of the University of Ljubljana in Ljubljana. Based on the three years of monitoring the design of the shingles will be optimized in addition.
Acknowledgement
The authors acknowledge the financial support of the Slovenian-Italian project DURASOFT, funded under the Interreg Italia-Slovenia 2014–2020 programme, and the Slovenian Research Agency (ARRS) within research programme P4-0015 (Wood and lignocellulosic composites), and the Infrastructure Centre (IC LES PST 0481-09). The Ministry of Agriculture, Forestry and Food, under the V4-2017 project, also supported part of the published research.
Disclosure statement
No potential conflict of interest was reported by the author(s).
Additional information
Funding
References
- Anonymous, 2022. Onesnaženost zraka v Sloveniji v letu 2021 (Pollution of air in Slovenia in 2021). Ministrstvo za okolje in prostor, Hidrometeorološki zavod Republike Slovenije, Ljubljana, Slovenia.
- Anonymous, 2023. Roof definition & meaning – Merriam-Webster. Available from: https://www.merriam-webster.com/dictionary/roof [Accessed 17 January 2023].
- ARSO, 2023. Agencija Republike Slovenije za okolje / Slovenian environmental agency. Available from: http://meteo.arso.gov.si/met/sl/archive/ [Accessed 20 January 2023].
- Brate, T., 1988. Pantzova zicnica v Soteski : tehniska dediscina Slovenije. Pionir, 43, 15–17.
- Brischke, C., et al., 2008. Monitoring the “material climate” of wood to predict the potential for decay: results from in situ measurements on buildings. Building and Environment, 43, 1575–1582. doi:10.1016/j.buildenv.2007.10.001.
- Brischke, C. and Alfredsen, G., 2020. Wood-water relationships and their role for wood susceptibility to fungal decay. Applied Microbiology and Biotechnology, 104, 3781–3795. doi:10.1007/s00253-020-10479-1.
- Brischke, C. and Lampen, S.C., 2014. Resistance based moisture content measurements on native, modified and preservative treated wood. European Journal of Wood and Wood Products, 72, 289–292. doi:10.1007/s00107-013-0775-3.
- Brischke, C. and Thelandersson, S., 2014. Modelling the outdoor performance of wood products – A review on existing approaches. Construction and Building Materials, 66, 384–397. doi:10.1016/j.conbuildmat.2014.05.087.
- Bucşa, L. and Bucşa, C., 2010. Deterioration of wooden heritage in outdoor exposure in Romania. Document no.: IRG/WP 10-40535. Proceedings IRG Annual Meeting, Stockholm, Sweden.
- Cevc, T., Kopač, V., and Suhadolc, J., 1993. Velika planina : življenje, delo in izročilo pastirjev. [samozal.] T. Cevc : Institut za slovensko narodopisje Znanstvenoraziskovalnega centra Slovenske akademije znanosti in umetnosti, Ljubljana.
- DIN, 2019. DIN 68119:2019-04, Holzschindeln. Beuth Verlag GmbH, Berlin.
- Eaton, R.A. and Hale, M.D.C., 1993. Wood : decay, pests, and protection. London; New York: Chapman & Hall.
- Esteves, B.M. and Pereira, H.M., 2009. Wood modification by heat treatment: a review. Bioresources, 4, 370–404.
- Fredriksson, M., et al., 2016. Microclimate and moisture content profile measurements in rain exposed Norway spruce (Picea abies (L.) Karst.) joints. Wood Material Science & Engineering, 11, 189–200. doi:10.1080/17480272.2014.965742.
- Gobakken, L.R., Mattsson, J., and Alfredsen, G., 2008. In-service performance of wood depends upon the critical in-situ conditions. case studies. In: Proceedings IRG annual meeting. Stockholm, Sweden.
- Heshmati, S., Mazloomi, M., and Evans, P., 2018. Optimizing surface micro grooving to reduce the checking and cupping of Douglas fir, western hemlock and white spruce decking exposed to natural weathering. Journal of Manufacturing and Materials Processing, 2, 67. doi:10.3390/jmmp2040067.
- Hill, C., Altgen, M., and Rautkari, L., 2021. Thermal modification of wood—a review: chemical changes and hygroscopicity. Journal of Materials Science, 56, 6581–6614.
- Humar, M., et al., 2017. Thermal modification of wax-impregnated wood to enhance its physical, mechanical, and biological properties. Holzforschung, 71, 57–64. doi:10.1515/hf-2016-0063.
- Humar, M., et al., 2019. The performance of wood decking after five years of exposure: verification of the combined effect of wetting ability and durability. Forests, 10, 903. doi:10.3390/f10100903.
- Humar, M., et al., 2020. Monitoring a building made of CLT in Ljubljana. Wood Material Science & Engineering, 15, 335–342. doi:10.1080/17480272.2020.1712740.
- Humar, M., Lesar, B., and Kržišnik, D., 2021. Vpliv podnebnih sprememb na dinamiko glivnega razkroja lesa v Sloveniji. Acta Silvae et Ligni, 125, 53–59. doi:10.20315/asetl.125.5.
- Isaksson, T., Brischke, C., and Thelandersson, S., 2013. Development of decay performance models for outdoor timber structures. Materials and Structures, 46, 1209–1225. doi:10.1617/s11527-012-9965-4.
- Isaksson, T. and Thelandersson, S., 2013. Experimental investigation on the effect of detail design on wood moisture content in outdoor above ground applications. Building and Environment, 59, 239–249. doi:10.1016/j.buildenv.2012.08.023.
- Jerin, A. and Povse, M., 2017. Skodlarstvo. http://www.mk.gov.si/fileadmin/mk.gov.si/pageuploads/Ministrstvo/Razvidi/RKD_Ziva/Rzd-02_00059.pdf.
- Jones, D. and Brischke, C., 2017. Performance of bio-based building materials. Duxford; Cambridge; Kidlington: Woodhead Publishing.
- Kain, G., et al., 2020. Suitability of wooden shingles for ventilated roofs: an evaluation of ventilation efficiency. Applied Sciences, 10, 6499. doi:10.3390/APP10186499.
- Kaplan, J.O., Krumhardt, K.M., and Zimmermann, N., 2009. The prehistoric and preindustrial deforestation of Europe. Quaternary Science Reviews, 28, 3016–3034. doi:10.1016/j.quascirev.2009.09.028.
- Keržič, E., Lesar, B., and Humar, M., 2021. Influence of weathering on surface roughness of thermally modified wood. Bioresources, 16, 4675–4692. doi:10.15376/biores.16.3.4675-4692.
- Koželj, B., 2020. Skodlarstvo Bojan Koželj s.p. Available from: http://www.skodlarstvo.si/ [Accessed 27 April 2020].
- Kozlov, V. and Kisternaya, M., 2014. Biodeterioration of historic timber structures: a comparative analysis. Wood Material Science & Engineering, 9, 156–161. doi:10.1080/17480272.2014.894573.
- Kozorog, E., 1992. Žičnica Golobar – gozdarski muzej na prostem. Planinski vestnik, 93, 395.
- Kržišnik, D., et al., 2018. Micro and material climate monitoring in wooden buildings in sub-Alpine environments. Construction and Building Materials, 166, 188–195. doi:10.1016/j.conbuildmat.2018.01.118.
- Kurek, J., 2019. Wooden orthodox church architecture in a country landscape after World War II – In the area of the former Eastern Galicia. In: IOP conference Series: Materials Science and Engineering.
- Lesar, B., et al., 2011. Wax treatment of wood slows photodegradation. Polymer Degradation and Stability, 96, 1271–1278. doi:10.1016/j.polymdegradstab.2011.04.006.
- Lesar, B. and Humar, M., 2011. Use of wax emulsions for improvement of wood durability and sorption properties. European Journal of Wood and Wood Products, 69, 231–238. doi:10.1007/s00107-010-0425-y.
- MacKenzie, C.E., 2007. Timber service life design guide. Forest and Wood Products Australia, World Trade Centre, Vic.
- Marais, B.N., Brischke, C., and Militz, H., 2022. Wood durability in terrestrial and aquatic environments–a review of biotic and abiotic influence factors. Wood Material Science & Engineering, 17, 82–105, doi:10.1080/17480272.2020.1779810.
- Meyer-Veltrup, L., et al., 2017. The combined effect of wetting ability and durability on outdoor performance of wood: development and verification of a new prediction approach. Wood Science and Technology, 51, 615–637. doi:10.1007/s00226-017-0893-x.
- Meyer, L., et al., 2016. Critical moisture conditions for fungal decay of modified wood by basidiomycetes as detected by pile tests. Holzforschung, 70, 331–339. doi:10.1515/hf-2015-0046.
- Niklewski, J., van Niekerk, P.B., and Marais, B.N., 2022. The effect of weathering on the surface moisture conditions of Norway spruce under outdoor exposure. Wood Material Science & Engineering. doi:10.1080/17480272.2022.2144444.
- Olek, W., Majka, J., and Czajkowski, Ł., 2013. Sorption isotherms of thermally modified wood. hfsg, 67, 183–191. doi:10.1515/hf-2011-0260.
- Palanti, S., et al., 2014. A case study: The evaluation of biological decay of a historical hayloft in Rendena Valley, Trento, Italy. International Biodeterioration & Biodegradation, 86, 179–187. doi:10.1016/j.ibiod.2013.06.026.
- Plackett, D., Chittenden, C.M., and Preston, A.F., 1984. Exterior weathering trials on Pinus radiata roofing shingles. New Zealand Journal of Forestry Science, 14, 368–381.
- Pouska, V., Macek, P., and Zíbarová, L., 2016. The relation of fungal communities to wood microclimate in a mountain spruce forest. Fungal Ecology, 21, 1–9. doi:10.1016/j.funeco.2016.01.006.
- Rep, G., Pohleven, F., and Kosmerl, S., 2012. Development of the industrial kiln for thermal wood modification by a procedure with an initial vacuum and commercialisation of modified Silvapro wood. In: D. Jones, H. Militz, M. Petrič, F. Pohleven, H. Humar, and M. Pavlič, eds. Proceedings of the 6th European conference on wood modification. Ljubljana: University of Ljubljana, 11–17.
- Rüther, P. and Time, B., 2015. External wood claddings – performance criteria, driving rain and large-scale water penetration methods. Wood Material Science & Engineering, 10, 287–299. doi:10.1080/17480272.2015.1063688.
- Šarf, F., 1976. Lesene strehe v Sloveniji. Slovenski etnograf, 29, 53–74.
- Scheffer, C.T., 1971. Climate index for estimating potential for decay in wood structures above ground. Forest Products Journal 21, 25–31.
- Schmidt, O., 2006. Wood and tree fungi: biology, damage, protection, and use. Berlin Heidelberg: Springer-Verlag.
- Stamm, A.J., 1927. The electrical resistance of wood as a measure of its moisture content. Industrial & Engineering Chemistry, 19, 1021–1025. doi:10.1021/ie50213a022.
- Stienen, T., Schmidt, O., and Huckfeldt, T., 2014. Wood decay by indoor basidiomycetes at different moisture and temperature. hfsg, 68, 9–15. doi:10.1515/hf-2013-0065.
- Teodorescu, I., et al., 2017. Influence of the climatic changes on wood structures behaviour. Energy Procedia, 112, 450–459.
- Unger, A., Schniewind, A.P., and Unger, W., 2001. Conservation of wood artifacts : a handbook. Berlin; London: Springer.
- Valvasor, J., et al., 1969. Valvasorjevo berilo. Ljubljana: Mladinska knjiga.
- Wagenführ, R., 2014. Holzatlas. 4th ed. Leipzig, Germany: Fachbuchverlag.
- Zabel, R.A. and Morrell, J.J., 2020. Wood microbiology : decay and its prevention. 2nd ed. Amsterdam: Academic Press.
- Žlahtič-Zupanc, M., Lesar, B., and Humar, M., 2018. Changes in moisture performance of wood after weathering. Construction and Building Materials, 193, 529–538. doi:10.1016/j.conbuildmat.2018.10.196.