ABSTRACT
Poor condition of roofs in terms of decay of shingles and loss of tar-based surface treatments has been revealed in a number of churches at various geographical locations in mid-Sweden. The condition of tars obtained from shingles of selected churches in mid-Sweden were analysed and obtained data were compared with geographical locations of the churches. According to gas chromatography-mass spectroscopy result, dehydroabietic acid was the dominant compound found in the exposed tars from the churches except in church of Malung where similar amounts of retene was found. Oxidised resin acids were found in exposed tars from the churches but not in commercially available pit burned tar. Tar from church shingles had higher char residues than the pit burned tar which was totally consumed when heated under nitrogen gas up to 750°C using thermogravimetric analysis. Scanning electron microscopy with energy dispersive X-ray spectrometer analysis revealed higher amounts of silicates in some exposed tars; however, these compounds constituted only a minor portion of the organic matter in these specimens. Based on the data obtained in the study other factors rather than simply geographical location of churches seemed to be of importance to explain differences of the investigated properties of the church tars.
KEYWORDS:
Introduction
Wood can be used as a construction material if it is allowed to dry out and the moisture content is kept under certain limits. Traditionally, wood shingles have been used as outer roof material in old buildings and barns, where the replacement of worn-out or broken ones is relatively straightforward. In larger buildings, like church roofs, these shingles are thicker, possess a different shape and are often treated to enhance durability. The treatments are often based on wooden tar alone or with various additives. Wooden tar was one of Sweden’s most important exports for centuries, serving as a preservative for wood exposed to harsh conditions, including ship decking and rigging (Allen Citation2013). It is still used today to treat wooden furniture exposed to the elements, such as a flavouring agent in candies, food, and alcohol, enhancing the grip on the handles of baseball bats, and additive in some paints.
Awareness of the low sustainability and sometimes toxic properties of many synthetic products and preservatives like asbestos and chromated copper arsenate (CCA), has increased interest in reverting to treatments with biobased products. Similar trends have also been seen in other products from heating wood produced under more or less inert conditions, i.e. thermally modified wood timber, torrefaction, and production of biochar along with pyrolysis oil and gases (Sandberg et al. Citation2021).
Wooden tars are traditionally produced along with charcoal in a two-stage process; a limited combustion of often extractive-rich wood in the presence of air followed by closure of air flow and heating under pyrolytic conditions. The resulting tar is collected at the bottom of the chamber, yielding about 5% of pit burned tar. In many countries, Scots pine is used for wood tar production as heartwood in the lower parts of the stem can contain a high concentration of extractives as well as a favourable composition of compounds applicable for various purposes (Ekeberg et al. Citation2006). This composition includes antioxidants such as resin acids and phenols, contributing to the fungicidal effect of the tar (Paghdal and Schwartz Citation2009, Jaouadi et al. Citation2021). It was found that the composition of resin acids was dependent on the production temperature of tar and less abietic acid was found at the higher temperature (Egenberg et al. Citation2002). Pyrolysis at higher temperatures than normally used for the production of wood tars, favours resistance to wood-destroying organisms (Mohan et al. Citation2008, Temiz et al. Citation2010, Oramahi et al. Citation2014).
The presence and composition of tar exposed to various conditions has been studied (Reunanen et al. Citation1990, Hjulström et al. Citation2006). The properties of the tar layer will eventually change due to solidification of the resinous tar material, evaporation of volatiles and leaching when exposed to sun and weather conditions. The presence of abietic acid was found to decrease by using a higher production temperature but not by following heating aiming at higher viscosity of the product (Egenberg et al. Citation2003). A common method to increase the durability and stability of wooden shingles of church roofs in Sweden and other places has been to treat the shingles with wooden tar. When it comes to detailed descriptions of how the roofs have been maintained over the years, including the type of surface treatments often based on pine tar, the frequency of application, and the replacement of degraded and broken roof parts, exist according to the church records. In addition, the knowledge of old methods was not always transferred to the next generations or used by restorers. The introduction of alternative preservative surface treatments became common in the mid-1800s, but a return to using biobased pine tar has been seen more recently. The viscosity of pit-burned tar from pine may vary but could be adjusted by heating or by adding additives and fillers as has been used before i.e. pigments of iron oxides or vitriols, lead sludge, asbestos and so on. In this way, treatments were intended to be more durable as well as easy to apply, achieving a stable tar layer on the shingles. In this context a simple process to promote deeper penetration of tar into disrupted and open-bordered pits of Scots pine wood involving heating the wood before soaking it in tar has been suggested to improve the fixation of the tar to the wood structure (Sheikh et al. Citation2013). The condition of a building’s roof is of large importance and a non-functioning roof will lead to an accelerated deterioration of the building as a whole. Inspections revealed variations in the condition of roof material among churches and positions on the buildings, as observed in various locations in mid-Sweden (Mellgren et al. Citation2023). Examples of decay of shingles and loss of tar-based surface treatments have been observed.
The objective of this study was to determine whether differences in thermal stability and chemical composition exist between tars from roofs and some facades from a series of churches from mid-Sweden and whether the geographical location of churches could be related to the differences in properties obtained. Such information should be of great concern for understanding the stability and life span of such roof wood sheathing. The studies include an investigation of the composition of the tar by a series of analytical methods as well as their thermal stability by heating under inert conditions. A higher thermal stability indicates that the material is more chemically stable with stronger interaction between its constituents and that it is less volatile. Determination of the decay of wooden roofs by density measurement and identification of degrading microbiological species will be presented in the following study.
Material and methods
The geolocation of churches in mid-Sweden used in studies on the chemical composition and properties of tar from exposed shingles is illustrated in .
Figure 1. The map of Sweden with the geolocation of the analysed churches. The dots represent the geolocation of the churches, and the text below is the name of the respective churches.
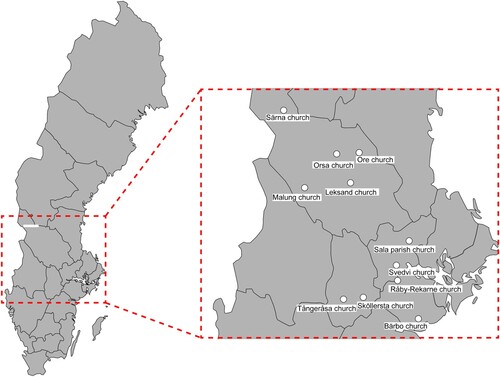
Surface treatments of selected shingles from roofs of churches in mid-Sweden (2020 spring) were isolated by scraping manually with a stainless-steel blade (Leica DB80 LX, Nussloch, Germany) amounting to 0.1 g. Studied tar samples from shingles of eleven selected churches are listed in . One commercially available tar from burning Scots pine (Dalbränd tjära, Claessons Trätjära AB, Gothenburg, Sweden) in a pit was used as a comparative material.
Table 1. Churches with the respective location of the specimens collected for analysis.
Fourier transform infrared spectroscopy (FTIR) was recorded using a PerkinElmer FTIR Frontier spectrometer (Waltham, MA, USA) equipped with UATR Diamond/ZnSe ATR (Single Reflection) over the wavenumber range of 4000–600 cm−1 with 8 scans at a resolution of 4 cm−1.
Thermal gravimetric analysis (TGA) was determined using a PerkinElmer TGA 4000 (Waltham, MA, USA), whereby 4 ± 1 mg of each specimen was loaded in an alumina crucible and heated at a rate of 20°C min−1 from 30 to 800°C under an N2 flow rate of 20 ml min−1. At least, three replicates were performed for each specimen.
Scanning electron microscopy (SEM) Jeol JSM-IT300LV (Tokyo, Japan) equipped with an energy-dispersive X-ray spectrometer (EDX) was used to analyse the morphology and elemental composition of the specimens. The spectrometer was controlled through the software Oxford Instrument ZAtec V3.1 (Buckinghamshire, UK). The specimens without coating were examined by secondary electrons, with the electron beam acceleration voltage set at 15 kV for SEM and EDX, under low-vacuum mode at 100 Pa, with an approximately 10 mm working distance. Approximately 160 s of the scanning time was used for the EDX mapping. Each specimen had three replicates of the analysis at different spots.
Gas chromatography equipped with a mass spectrometer (GC-MS) of tars in the study was performed as their trimethylsilylates. Samples from church tars (ca. 10 mg) were mixed in 1 ml chloroform in a small test tube and ultra-sonicated for 15 min. The tars were trimethylsilylated with 0.1 ml BSTFA + 10% trimethylsilyl chloride (TMSCl) at 67°C for 40 min, transferred to 1 ml vial and after sedimentation the supernatant was analysed by GC-MS. Pit-burned tar was solubilised by the treatment. Corresponding signals from the chromatogram were identified by MS and NIST library in which a hit rate of >50% was used. The area under absorption peaks in the chromatogram was used to estimate the relative amounts of compounds in the tars. GC-MS: Agilent 6890, carrier gas: He (split flow), column: 30 m long and 0.25 mm thick Zebron, ZB-5MS, film thickness of 0.25 µm. GC temperature programme: equilibrating period of 0.2 min, 50°C for 2 min, 10°C min−1, final time at 325°C for 10.5 min. The MS-interface temperature was 230°C and the MS-quadrupole temperature was 150°C.
Results and discussion
Infrared spectroscopy
The analysis of the solid tar specimens done by FTIR showed characteristic absorptions, including a band of absorptions at 2950–2850 cm−1 representing C–H stretching, as well as C-H bending at 1455 and 1379 cm−1, especially noticeable for Orsa-N specimen (). These absorptions were significantly more pronounced than those observed in corresponding unmodified wood spectra in Figure S1. Additionally, a band at 1696 cm−1 representing unconjugated C=O stretching, and a smaller broad band at 3600–3100 cm−1 related to O–H stretching were also observed. These absorptions could be related to corresponding structures in resin acids, as has been reported for the absorption of abietic acids (Ren et al. Citation2015).
Figure 2. ATR-FTIR spectra of tars collected from roof and façade shingles from churches in mid-Sweden.
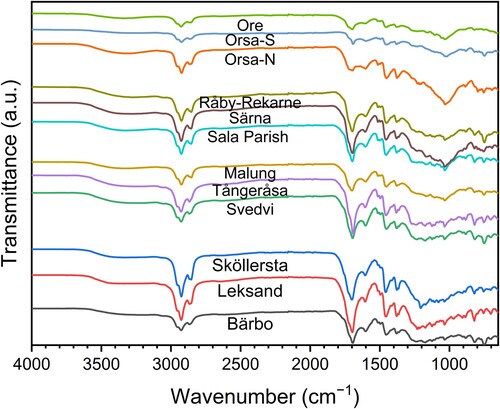
The FTIR spectra of pit-burned tar from Scots pine (Figure S1) and abietic acid (Ren et al. Citation2015) showed large similarities. This resemblance is also true for the tar obtained from the shingles; however, absorptions at 1031 cm−1 were found in the tar specimens from Orsa and Ore churches, whereas they were minimal in the specimens of Bärbo, Leksand, Sköllersta, Svedvi, and Tångeråsa (). This absorption could be related to a material different from that found in pit-burned tar, such as Si–O stretching in alumina silicate (Puligilla and Mondal Citation2015) and C–O stretching absorption in wood (Figure S1). Despite the presence of an absorption band at 16030 cm−1, representing aromatic and unsaturated C–H stretching in all church specimens, it seemed to be more pronounced (relative to 2925 cm−1) in the specimens from the church of Svedvi.
Gas chromatography-mass spectroscopy (GC-MS)
Dehydroabietic acid (7) was found to be the dominant identified compound in all church samples except tar from Malung church where retene (17) was found in similar quantities (). The polyaromatic compound (17) was found in samples from all churches and in pit-burned tar (Tables S1 and S2). Phenols were found in pit-burned and church tars; however, their distribution varied. Vanillic acid but not guaiacol was found in the church tars, while the composition of these phenols showed an opposite trend for the pit-burned tar. Other compounds that were found in significant amounts in church tars were mostly composed of fatty acids and similar types of compounds.
Figure 3. Distributions of the six most common compounds in tar from churches and pit-burned tar performed by GC-MS: 3 (Methyl 7-Oxodehydroabietate), 7 (Dehydroabietic acid), 8 (10H-Benzo[b][1,8]naphthyridin-5-one, 7-ethyl-2,4-dimethyl-), 9 (Methyl-dehydroabietate), 10 (Methyl-levopimarate), 13 (Methyl abiet-7-en-18-oate), 14 (Cryptomaric acid), 15 (Stearic acid), 17 (Retene), 21 (Palmitic acid), 22 (Pyrodruvic acid), 23 (18-Norabieta-8,11,13-triene (4b,8-Dimethyl-2-isopropylphenanthrene, 4b,5,6,7,8,8a,9,10-octahydro-)), 24 (Azelaic acid), 25 (Dibenzofulvene), 26 (Vanillic acid), and 43 (monoterpene).
![Figure 3. Distributions of the six most common compounds in tar from churches and pit-burned tar performed by GC-MS: 3 (Methyl 7-Oxodehydroabietate), 7 (Dehydroabietic acid), 8 (10H-Benzo[b][1,8]naphthyridin-5-one, 7-ethyl-2,4-dimethyl-), 9 (Methyl-dehydroabietate), 10 (Methyl-levopimarate), 13 (Methyl abiet-7-en-18-oate), 14 (Cryptomaric acid), 15 (Stearic acid), 17 (Retene), 21 (Palmitic acid), 22 (Pyrodruvic acid), 23 (18-Norabieta-8,11,13-triene (4b,8-Dimethyl-2-isopropylphenanthrene, 4b,5,6,7,8,8a,9,10-octahydro-)), 24 (Azelaic acid), 25 (Dibenzofulvene), 26 (Vanillic acid), and 43 (monoterpene).](/cms/asset/84f7d865-0b3f-4cef-afc5-edd6b4a85420/swoo_a_2311169_f0003_oc.jpg)
Abietic acid (6) was one of the major resin acids in pit-burned tar but was low in the exposed tar from the churches ( and S2, S3). Studies on the decrease in the content of 6 and other resin acids during weathering conditions of tars applied on glass plates have been undertaken (Egenberg et al. Citation2003). Degradation of 6 was proposed to involve oxidation and thermal dehydration processes (Egenberg et al. Citation2003, Ren et al. Citation2015, Li et al. Citation2019). Oxidised resin acid (3) was found in church tars but was not detected in the pit-burned tar. Methylated 7-oxo-dehydroabietic acid (4) was more evident in tar from churches of Särna and Tångeråsa than in other churches in the study indicating a more seasoned tar in these old churches. Also, the methyl ester of a reduced form of abietic acid (13) was found in these and a few other churches. Methylated dehydroabietic acid (9) has been proposed as a biomarker of distilled tar in soil from ancient tar production sites (Hjulström et al. Citation2006). Compound (9) was found in pit-burned tar but only in tar from shingles of Bärbo church. On the other hand, methylated resin acid (10) was found in the tar of the church shingles but not in Bärbo and a few others in the study (Tables S2 and S3).
Thermogravimetric analysis
The thermal behaviour, investigated through thermal gravimetric analysis (TGA), is presented in , while the corresponding onset temperature, offset temperature, and residue at 800°C are presented in . The TGA curve of pit-burned tar showed a rapid weight loss, with less than 10 wt.% remaining after 350°C, and complete consumption after 700°C. This observation indicated that the original compounds are highly volatile and readily decomposes during heating.
Table 2. Onset and offset temperature of the main decomposition based on the TGA results in , and the weight percentage of the char residue from tars at 800°C.
The residue at 800°C from the tar specimens obtained from churches exhibits variability, ranges from 11 wt.% of the Tångeråsa specimen to 45 wt.% of the Orsa-N specimen. Specimens of Orsa-S, Malung, and Leksand had a notable amount of residues at 800°C, account for approximately 30 wt.% of their initial weight. In contrast, specimens of Råby-Rekarne, Särna, Svedvi, Ore, Sköllersta, Sala, and Bärbo were distributed in the lower ranges, between 12 and 20 wt.%. The higher weight percentage at elevated temperatures of the tar specimens from churches, when compared to pit-burn tar, indicates a high content of materials that withstand thermal decomposition, such as the presence of inorganic incombustible material (see SEM-EDX).
Scanning electron microscopy-energy-dispersive X-ray spectrometry
SEM-EDX was used to evaluate the elemental composition of the tar specimens. Table S3 indicates that the elemental carbon (C) and oxygen (O) constitute most elements across all specimens, primarily attributed to the constituents of tar. In (a,b), the SEM images of Orsa and Särna specimens reveal white-colour spots, indicative of heavier elements such as elemental silicon (Si), as supported by the elemental composition in Table S3. The presence of elemental Si was likely sourced from silicate-based compounds, such as potassium alumina silicate mineral, which were more distinctly observed in the specimens from Särna and Orsa churches. Additionally, other tar specimens, like from Svedvi church as shown in Table S3, had lower content of elemental Si and other metallic elements. Notably, tar from Svedvi church has a higher content of elemental aluminium (Al), probably existing in a silicate form, as indicated by its elemental mapping in (c).
Conclusions
The poor condition of roofs in terms of decay of shingles and loss of tar-based surface treatments has been revealed in many churches at various geographical locations in mid-Sweden. The condition of tars obtained from the shingles of selected churches in mid-Sweden were analysed using infrared spectroscopy (FTIR), gas chromatography-mass spectroscopy (GC-MS) as their trimethylsilylates, thermogravimetric analysis (TGA), and scanning electron microscopy (SEM) equipped with an energy-dispersive X-ray spectrometer (EDX) and obtained analysis data were compared with geographical locations of the churches.
Variations in composition between the tars from shingles of the churches were observed. The outdoor exposure of the tars was suggested to be the reason that vanillic acid was found in tar from all churches but not in the pit-burned tar. Relatively high content of resin acids was found in most of the churches like Tångeråsa except the church of Malung which had a lower content of resin acids but more retene. As the composition of roof tar from the northern churches of Orsa and Leksand showed more similarity with tar from other churches rather than from the church of Malung the importance of the geographical location of churches was suggested to be minor and probably more related to other factors such as individual application differences.
Moreover, the residue obtained after TGA was higher for tar from Orsa-N than for Tångeråsa in accordance with results obtained from SEM-EDX; a tar with a more stabilised carbon structure containing higher amounts of inorganic compounds in the former than in the latter one. More detailed analysis of the strength parameters of the exposed tar as well as studies performed under more controlled exposure conditions will contribute to a more full understanding of the stability of exposed church tars.
Supporting_information
Download MS Word (439.1 KB)Acknowledgements
We thank Ylva Blank and co-workers at Stiftelsen Kulturmiljövård (KM) and Per Liljelind at the Department of Chemistry, Umeå University for fruitful collaboration. Support through the Swedish National Heritage Board, CT WOOD a centre of excellence at Luleå University of Technology supported by the Swedish wood industry, and from the Swedish Research Council for Environment, Agricultural Sciences, and Spatial Planning (FORMAS), project “Biobased fire protection of wood panel for exterior conditions by using phosphorylated lignin from wheat straw” 2021-00818, is gratefully acknowledged. We also acknowledge LUMIA (Luleå Material Imaging and Analysis) for providing the SEM–EDX instrument.
Disclosure statement
No potential conflict of interest was reported by the author(s).
Data availability statement
Basic data used to present findings in the manuscript will be available from the corresponding author on request.
Additional information
Funding
References
- Allen, L.V., Jr., 2013. Basics of compounding with tars. International Journal of Pharmaceutical Compounding, 17, 400–410.
- Egenberg, I.M., et al., 2002. Characterisation of traditionally kiln produced pine tar by gas chromatography-mass spectrometry. Journal of Analytical and Applied Pyrolysis, 62, 143–155.
- Egenberg, I.M., Holtekjolen, A.K., and Lundanes, E., 2003. Characterisation of naturally and artificially weathered pine tar coatings by visual assessment and gas chromatography-mass spectrometry. Journal of Cultural Heritage, 4, 221–241.
- Ekeberg, D., et al., 2006. Qualitative and quantitative determination of extractives in heartwood of Scots pine (Pinus sylvestris L.) by gas chromatography. Journal of Chromatography A, 1109, 267–272.
- Hjulström, B., Isaksson, S., and Hennius, A., 2006. Organic geochemical evidence for pine tar production in middle Eastern Sweden during the Roman Iron Age. Journal of Archaeological Science, 33, 283–294.
- Jaouadi, I., et al., 2021. Chemical variability and antioxidant activity of Cedrus atlantica Manetti essential oils isolated from wood tar and sawdust. Arabian Journal of Chemistry, 14 (12), 103441.
- Li, Y., et al., 2019. Thermal stability of abietic acid and its oxidation products. Energy & Fuels, 33, 11200–11209.
- Mellgren, M., et al., 2023. Stavspån i Strängnäs stift och Västerås stift: Fördjupade undersökningar etapp 2. Stiftelsen Kulturmiljövård 2022:27.
- Mohan, D., et al., 2008. Fungicidal values of bio-oils and their lignin-rich fractions obtained from wood/bark fast pyrolysis. Chemosphere, 71, 456–465.
- Oramahi, H.A., Diba, F., and Nurhaida, 2014. New bio preservatives from lignocelluloses biomass bio-oil for anti termites Coptotermes curvignathus Holmgren. Procedia Environmental Sciences, 20, 778–784.
- Paghdal, K.V. and Schwartz, R.A., 2009. Topical tar: back to the future. Journal of the American Academy of Dermatology, 61 (2), 294–302.
- Puligilla, S. and Mondal, P., 2015. Co-existence of aluminosilicate and calcium silicate gel characterized through selective dissolution and FTIR spectral subtraction. Cement and Concrete Research, 70, 39–49.
- Ren, F., et al., 2015. An investigation of the oxidation mechanism of abietic acid using two-dimensional infrared correlation spectroscopy. Journal of Molecular Structure, 1084, 236–243.
- Reunanen, M., Ekman, R., and Heinonen, M., 1990. Long-term alteration of pine tar in a marine environment. Holzforschung, 44, 277–278.
- Sandberg, D., et al., 2021. Wood modification technologies: principles, sustainability, and the need for innovation. Baton Rouge, FL: CRC Press. ISBN: 978-1-138-49177-9.
- Sheikh, A.A., Hansson, L., and Morén, T., 2013. Distribution of preservatives in thermally modified Scots pine and Norway spruce sapwood. Wood Science and Technology, 47, 499–513.
- Temiz, A., et al., 2010. Efficiency of bio-oil against wood destroying organisms. Journal of Biobased Materials and Bioenergy, 4 (3), 1–7.