Abstract
It is well known that free fatty acids (FFAs) play a central role in insulin resistance. However, increasing evidence suggests that they are also implicated in the control of pancreatic insulin secretion. Simultaneous exposure of pancreatic β-cells to high levels of glucose and to saturated FFAs results in a substantial increase in insulin release, whereas chronic exposure may lead to a desensitization and suppression of secretion. A source of fatty acid, either exogenous or endogenous, is necessary to support normal insulin secretion. A rapid increase in fatty acids potentiates glucose-stimulated secretion by increasing the concentration of fatty acyl-coenzyme A or complex lipids, which act indirectly by modulating key enzymes, such as protein kinase C, or directly by modulating the exocytotic machinery. Mathematical models including FFAs in controlling insulin secretion are devised. Finally, insulin secretion in a human model of very low-fat diet, the malabsorptive bariatric surgery for morbid obesity, is described.
Introduction
Although there is a large body of literature showing that free fatty acids (FFAs) play a pivotal role in the cellular mechanisms involved in insulin resistance 1–4Citation1Citation2Citation3Citation4, relatively few reports deal with the role of FFAs in the insulin secretion process.
In 1969, Crespin et al. Citation5 reported that a rapid onset of a two- to 12-fold increase in plasma insulin was observed in conscious dogs with acutely rising FFA concentration during infusion of sodium oleate into the circulation. The magnitude of the insulin response correlated with the mean FFA level during infusion. This observation remained isolated for about a decade.
In 1979 Campillo et al. Citation6 showed that in the isolated perfused pancreas of rat, oleic and octanoic acids potentiated glucose-induced insulin release without modification of the pattern of secretion. Thereafter, from time to time, isolated studies showed the effect of FFAs in increasing insulin secretion. Vara and Tamarit-Rodriguez Citation7 found that palmitate increased the insulin response of fed islets to glucose in a dose-related manner. In 1990 Sako and Grill Citation8 observed that in experimental animals hyperlipidaemia was associated with short-term stimulation, but long-term inhibition, of glucose-induced insulin secretion. Wolf et al. Citation9 described that glucose-induced phospholipid hydrolysis and free arachidonate accumulation in pancreatic islets may play a role in the signalling process leading to insulin secretion.
The role of lipotoxicity Citation10 in the derangement of the insulin secretory capacity of β-cells is evident in obese Zucker diabetic fatty (ZDF fa/fa) male rats, which have a leptin receptor mutation and consequent hyperleptinaemia. In these animals, a model of type 2 diabetes mellitus, the inability of leptin to suppress insulin secretion results in chronic hyperinsulinaemia and increases the de novo lipogenesis in pancreatic islets Citation11Citation12. Enhanced activity of fatty acid synthase (FAS), a key insulin-sensitive enzyme in lipogenesis, and overaccumulation of fat in pancreatic islets of obese ZDF rats, induce β-cell failure, resulting in frank diabetes Citation13.
The progression from insulin hypersecretion associated with insulin resistance to insulin secretion failure in male ZDF rats is a function of lipid accumulation in pancreatic β-cells. These rats develop progressive insulin resistance and glucose intolerance between 3 and 8 weeks of age and become overtly diabetic between 8 and 10 weeks; the development of diabetes is associated with a 10–50-fold increase in islet triglyceride content Citation14.
It has been shown that exogenous saturated long-chain fatty acids markedly potentiate glucose-induced insulin release and elevate long-chain acyl-coenzyme A (CoA) esters in the clonal β-cell line Citation15. The secretory action depended on the fatty acid chain length, occurred in the range 3–20 µM free palmitate concentration, and was reversible and inhibitable by the neuromodulator somatostatin.
When the elevated level of circulating FFAs in 18–24 h fasted rats was acutely lowered by infusion of the antilipolytic agent nicotinic acid, subsequent glucose-stimulated insulin secretion was completely ablated, but became supranormal when FFA concentration was maintained at high levels by coinfusion of a lipid emulsion plus heparin Citation16Citation17. Qualitatively similar results have also been obtained in humans Citation17Citation18. The axiom that glucose was the only secretagogue stimulus for pancreatic insulin secretion was, therefore, definitely refuted.
Insulin secretion models
When glucose concentration increases, insulin secretion is progressively stimulated. This dose–response relationship is depicted in . If the glucose concentration is briskly increased and maintained at a suprabasal level, insulin secretion shows an initial burst (first phase insulin secretion) followed by a gradually increasing secretion that approaches a nearly constant level after about 60 min (second phase insulin secretion). Prolonged exposure to hyperglycaemia determines a global increase in the insulin response, denoted as potentiation.
Fig. 1. Dose–response curve relating glucose uptake to insulin levels in normal subjects in conditions of euglycaemia.
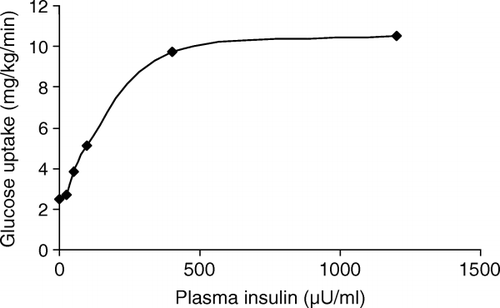
The β-cell response to an oral glucose challenge, such as after an oral glucose tolerance test, is much higher than that observed during an intravenous glucose infusion at matched glucose levels, as a consequence of the intestinal incretin secretion, mainly represented by glucose-dependent insulinotropic peptide (GIP) and glucagon-like peptide-1 (GLP-1).
In insulin resistance states, β-cell function is increased to overcome the impaired insulin action at the level of peripheral tissues, such as skeletal muscle, and at the level of the liver, by increasing the glucose output.
The methods most often used for assessing insulin secretion include the intravenous glucose tolerance test (IVGTT) and the hyperglycaemic clamp, which is also the reference test for first phase insulin secretion. The arginine test is based on the observation that the intravenous injection of arginine produces a large burst in insulin secretion which is also dependent on the current glucose levels. In comparison with the IVGTT, the arginine test releases a larger pool of insulin granules. The physiological significance of this pool is unknown. The response to arginine is also present in diabetic subjects in whom the first phase after the IVGTT is lacking.
β-Cell insulin secretion is generally computed using the C-peptide deconvolution method 19–21Citation19Citation20Citation21, which is based on a standardized model of C-peptide kinetics Citation22. In normal β-cells, the sensitivity–secretion relationship is expressed as a rectangular hyperbola Citation23. The product of insulin sensitivity and insulin secretory response equals a constant, named the “disposition index” (). Changes in insulin sensitivity are accompanied by compensatory modifications in β-cell sensitivity to glucose. Insulin resistance is accompanied by up-regulation of β-cell sensitivity, and thus hyperinsulinaemia, whereas higher insulin sensitivity is associated with a down-regulation of β-cell sensitivity.
Mechanisms of stimulation of insulin secretion
The role of fatty acids in controlling insulin secretion has been recently reviewed by Yaney and Corkey Citation24. These authors underline that a source of fatty acid, either exogenous or endogenous, is necessary to support normal insulin secretion. A rapid increase in fatty acid concentration potentiates glucose-stimulated secretion by increasing the concentration of fatty acyl-CoA or complex lipids that act indirectly by modulating key enzymes, such as protein kinase C, or directly by modulating the exocytotic machinery. The hypothesis is that nutrients stimulate insulin secretion through the simultaneous activation of two pathways, one involving accelerated adenosine triphosphate (ATP) production and increased intracellular calcium cations, and the other depending on the generation and accumulation of excess citrate in the cytosol and on the increase in cytosolic malonyl-CoA, which in turn blocks long-chain acyl-CoA transport into mitochondria. Glucose-stimulated insulin secretion is associated with decreased FFA oxidation Citation24.
The signal transduction model in nutrient-induced insulin secretion postulates that increased glycolysis leads to accelerated ATP production and, consequently, to an increased ratio of ATP to adenosine diphosphate (ADP) Citation25. The increase in ATP in the β-cells closes the KATP channel and determines the depolarization of the cell membrane, opening the voltage-dependent Ca2+ channels and raising the intracellular Ca2+. The rise in intracellular Ca2+ levels activates the kinase system, which controls insulin secretion, allowing the secretion granules of insulin to fuse with the cellular membrane, thus liberating insulin molecules. However, there is recent evidence that glucose-stimulated insulin secretion (GSIS) can also be independent of the KATP channel 26–28Citation26Citation27Citation28 and can be stimulated by other nutrients, such as FFAs. Further, the action of glucose in the absence of Ca2+ can be mimicked by FFAs Citation29.
According to the view that a dual signalling pathway is involved in the stimulation of insulin secretion by nutrients, a mathematical model in which insulin secretion rate is expressed as a function of both plasma glucose and FFA concentrations has been proposed recently Citation30. Model parameters, obtained by fitting the individual experimental data of plasma C-peptide concentration, give an estimated insulin secretion rate comparable to that obtained by the deconvolution method. This inclusive model of nutrient-stimulated insulin secretion is the first attempt to represent in a simple way a complex molecular mechanism of regulation of insulin secretion in the β-cell, and explains, at least in part, the “potentiation factor” used in previous models Citation30 to account for control factors other than glucose.
Dietary fatty acids and insulin secretion
Dietary supplementation of n-3 fatty acids from marine oils in gold-thioglucose-injected CBA/T6 mice, an animal model of type 2 diabetes, led to an improvement in glycosylated haemoglobin associated with a higher plasma insulin concentration. This suggests that the metabolic effects of n-3 fatty acids were mediated by insulin hypersecretion, rather than by an enhancement of peripheral insulin sensitivity Citation31
Monounsaturated fatty acids (MUFAs) exert a higher stimulatory effect on incretins than saturated fatty acids; however, this graded effect does not result in different insulin secretion. While a mixed meal constituting 80 g olive oil plus 50 g carbohydrates induced lower triacylglycerol and higher GLP-1 and GIP plasma concentrations than a mixed meal containing the same amount of butter, no differences in the concentrations of glucose, insulin and fatty acids were found Citation32.
In healthy volunteers, diets rich in saturated fatty acids (SFAs) or MUFAs exerted different effects on insulin sensitivity, with MUFAs improving and SFAs worsening insulin secretion, while β-cell function resulted unchanged Citation33.
The Finnish Diabetes Prevention Study showed that the incidence of type 2 diabetes mellitus was dramatically reduced by a 3 year intervention study, where the total fat intake was reduced to less than 30%, with a content of SFAs lower than 10% Citation34. In contrast, in the Nurses’ Health Study the total fat intake was not associated with the risk of diabetes mellitus after adjusting for other known risk factors. However, polyunsaturated fatty acid intake was related to a reduction in diabetes incidence, whereas trans fatty acids increased this risk Citation35. Finally, the 30 year dietary survey of Finnish, Italian and Dutch survivors of the Seven Countries Study showed that the incidence of diabetes was independent of dietary fat intake Citation36. Environmental and genetic factors, physical activity and other lifestyle-related factors should be taken in account to explain these apparently discordant results.
Increased levels of FFA may, through their effect on stimulating insulin secretion, have a protective event in reducing the development of diabetes in obese, insulin-resistant individuals. In obese people with normal pancreatic β-cells, FFAs are potent insulin secretagogues and can compensate for the insulin resistance that they produce. When chronically elevated plasma FFA levels were lowered in obese diabetic and non-diabetic subjects, insulin secretion rates decreased by 30–50% Citation37, indicating that the elevated plasma FFAs had supported 30–50% of basal insulin secretion. In contrast, in first degree relatives of patients with type 2 diabetes, FFAs are unable to compensate fully with adequately increased insulin secretion for the insulin resistance that they produce Citation38. This defect in FFA-stimulated insulin secretion can also be demonstrated in patients with impaired glucose tolerance Citation38 or with frank type 2 diabetes Citation38. These observations suggest that the obese individuals who develop type 2 diabetes may have a genetic predisposition to pancreatic β-cell failure.
Malabsorptive bariatric surgery as a model of very-low fat diet
Biliopancreatic diversion (BPD) is a bariatric surgical technique inducing massive lipid malabsorption and, therefore, it represents a fair model to evaluate how dietary lipid deprivation can affect glucose metabolism in humans. After BPD a large depletion of fats in the muscle cells was coupled with the normalization of insulin sensitivity Citation39. The insulin resistance reversion Citation40 probably occurred through a reduced tumour necrosis factor-α Citation41 and acetyl-coenzyme A carboxylase (ACC) Citation42, and an increased GLUT4 mRNA expression Citation41. Pyruvate kinase gene expression was also reduced, resulting in a reduction in the inhibition of pyruvate dehydrogenase activity Citation43.
Modified BPD in two sisters, affected by familiar lipoprotein lipase deficiency, decreased serum triglycerides while improving insulin sensitivity and reversing type 2 diabetes Citation44. The complete reversion of type 2 diabetes after BPD is an early phenomenon appearing in the first few days after the operation Citation45.
Morbidly obese subjects with normal glucose tolerance, impaired glucose tolerance and type 2 diabetes show characteristically an increased fasting insulin secretion rate, while in type 2 diabetics β-cell glucose sensitivity is depressed with lower insulin output after a glucose load. Following BPD, fasting insulin secretion rate and total insulin output decreased, although a tendency towards an improvement in β-cell glucose sensitivity in impaired glucose tolerance and type 2 diabetes was observed Citation46. Furthermore, β-cell glucose sensitivity remained significantly impaired in type 2 diabetic subjects compared with lean controls. In conclusion, malabsorptive bariatric surgery was associated with a rapid improvement in β-cell glucose sensitivity and co-ordinated changes in insulin sensitivity and total insulin output, the latter decreasing as the former increased.
Acronyms | ||
ACC | = | acetyl-coenzyme A carboxylase |
ATP | = | adenosine triphosphate |
BPD | = | biliopancreatic diversion |
CoA | = | coenzyme A |
FAS | = | fatty acid synthase |
FFA | = | free fatty acid |
GIP | = | glucose-dependent insulinotropic peptide |
GLP | = | glucagon-like peptide |
GSIS | = | glucose-stimulated insulin secretion |
IVGTT | = | intravenous glucose tolerance test |
MUFA | = | monounsaturated fatty acid |
SFA | = | saturated fatty acid |
ZDF rat | = | Zucker diabetic fatty (fa/fa) rat. |
References
- ColbergSRSimoneauJAThaeteFLKelleyDE. Skeletal muscle utilization of free fatty acids in women with visceral obesityJ Clin Invest199595184653
- KelleyDEGoodpasterBWingRRSimoneauJA. Skeletal muscle fatty acid metabolism in association with insulin resistance, obesity, and weight lossAm J Physiol1999277E113041
- KelleyDEKullerLHMcKolanisTMHarperPMancinoJKalhanS. Effects of moderate weight loss and orlistat on insulin resistance, regional adiposity, and fatty acids in type 2 diabetesDiabetes Care2004273340
- BajajMSuraamornkulSRomanelliAClineGWMandarinoLJShulmanGI Effect of a sustained reduction in plasma free fatty acid concentration on intramuscular long-chain fatty acyl-CoAs and insulin action in type 2 diabetic patientsDiabetes200554314853
- CrespinSRGreenoughWBSteinbergD. Stimulation of insulin secretion by infusion of free fatty acidsJ Clin Invest19694819343
- CampilloJEValdiviaMMRodriguezEOsorioC. Effect of oleic and octanoic acids on glucose-induced insulin release in vitroDiabete Metab197951837
- VaraETamarit-RodriguezJ. Glucose stimulation of insulin secretion in islets of fed and starved rats and its dependence on lipid metabolismMetabolism19863526671
- SakoYGrillVE. A 48-hour lipid infusion in the rat time-dependently inhibits glucose-induced insulin secretion and B cell oxidation through a process likely coupled to fatty acid oxidationEndocrinology199012715809
- WolfBAPasqualeSMTurkJ. Free fatty acid accumulation in secretagogue-stimulated pancreatic islets and effects of arachidonate on depolarization-induced insulin secretionBiochemistry19913063729
- LeeYHiroseHOhnedaMJohnsonJHMcGarryJDUngerRH. B-cell lipotoxicity in the pathogenesis of non-insulin-dependent diabetes mellitus of obese rats: impairment in adipocyte–β-cell relationshipsProc Natl Acad Sci USA1994911087882
- KiefferTJHellerRSLeechCAHolzGGHabenerJF. Leptin suppression of insulin secretion by the activation of ATP-sensitive K+ channels in pancreatic β-cellsDiabetes199746108793
- ZhouY-TShimabukuroMLeeYKoyamaKHigaMFergusonT Enhanced de novo lipogenesis in the leptin-unresponsive pancreatic islets of prediabetic Zucker diabetic fatty rats: role in the pathogenesis of lipotoxic diabetesDiabetes19984719048
- ShimabukuroMHigaMZhouY-TWangM-YNewgardCBUngerRH. Lipoapoptosis in the β-cells of obese prediabetic fa/fa rats: role of serine palmitoyltransferase overexpressionJ Biol Chem1998493248790
- PrentkiMVischerSGlennonMCRegazziRDeeneyJTCorkeyBE. Malonyl-CoA and long chain acyl-CoA esters as metabolic coupling factors in nutrient-induced insulin secretionJ Biol Chem1992267580210
- UngerRH. Lipotoxicity in the pathogenesis of obesity-dependent NIDDM. Genetic and clinical implicationsDiabetes19954486370
- SteinDTEsserVStevensonBLaneKEWhitesideJHDanielsMB Essentiality of circulating fatty acids for glucose-stimulated insulin secretion in the fasted ratJ Clin Invest199697272835
- SteinDTStevensonBChesterMWBasitMDanielsMBTurleySD The insulinotropic potency of fatty acids is influenced profoundly by their chain length and degree of saturationJ Clin Invest1997100398403
- DobbinsRMcGarryJDSteinDT. Normal glucose stimulated insulin secretion is dependent on free fatty acids in the 24-°to 48-hour fasted humanDiabetes199746504
- HovorkaRJonesRH. How to measure insulin secretionDiabetes Metab Rev19941091117
- EatonRPAllenRCSchadeDSEricksonKMStandeferJ. Prehepatic insulin production in man: kinetic analysis using peripheral connecting peptide behaviorJ Clin Endocrinol Metab1980515208
- PolonskyKSRubensteinAH. Current approaches to measurement of insulin secretionDiabetes Metab Rev1986231529
- Van CauterEMestrezFSturisJPolonskyKS. Estimation of insulin secretion rates from C-peptide levels. Comparison of individual and standard kinetic parameters for C-peptide clearanceDiabetes19924136877
- BergmanRNPhillipsLSCobelliC. Physiologic evaluation of factors controlling glucose tolerance in man: measurement of insulin sensitivity and B-cell glucose sensitivity from the response to intravenous glucoseJ Clin Invest198168145667
- YaneyGCCorkeyBE. Fatty acid metabolism and insulin secretion in pancreatic beta cellsDiabetologia2003461297312
- PrentkiMTornheimKCorkeyBE. Signal transduction mechanisms in nutrient-induced insulin secretionDiabetologia199740S3241
- AizawaTSatoYIshiharaFTaguchiNKomatsuMSuzukiN ATP-sensitive K+ channel-independent glucose action in rat pancreatic beta-cellAm J Physiol1994266C6227
- GembalMDetimaryPGilonPGaoZYHenquinJC. Mechanisms by which glucose can control insulin release independently from its action on adenosine triphosphate-sensitive K+ channels in mouse B cellsJ Clin Invest19939187180
- KomatsuMSchermerhornTAizawaTSharpGW. Glucose stimulation of insulin release in the absence of extracellular Ca2+ and in the absence of any increase in intracellular Ca2+ in rat pancreatic isletsProc Natl Acad Sci USA1995921072832
- KomatsuMSharpGW. Palmitate and myristate selectively mimic the effect of glucose in augmenting insulin release in the absence of extracellular Ca2+Diabetes1998473527
- MariASchmitzOGastaldelliAOestergaardTNyholmBFerranniniE. Meal and oral glucose tests for assessment of beta β-cell function: modelling analysis in normal subjectsAm J Physiol Endocrinol Metab2002283E115966
- SullivanDRYueDKCapogrecoCMcLennanSNicksJCooneyG The effects of dietary n-3 fatty acid in animal models of type 1 and type 2 diabetesDiabetes Res Clin Pract1990922530
- ThomsenCRasmussenOLousenTHolstJJFenselauSSchrezenmeirJ Differential effects of saturated and monounsaturated fatty acids on postprandial lipemia and incretin responses in healthy subjectsAm J Clin Nutr199969113543
- VessbyBUnsitupaMHermansenKRiccardiGRivelleseAATapsellLC Substituting dietary saturated for monounsaturated fat impairs insulin sensitivity in healthy men and women: The KANWU StudyDiabetologia.2001443129
- TuomilehtoJLindstromJErikssonJGValleTTHamalainenHIlanne-ParikkaP Finnish Diabetes Prevention Study Group. Prevention of type 2 diabetes mellitus by changes in lifestyle among subjects with impaired glucose toleranceN Engl J Med2001344134350
- SchulzeMBHoffmannKMansonJEWillettWCMeigsJBWeikertC Dietary pattern, inflammation, and incidence of type 2 diabetes in womenAm J Clin Nutr20058267584
- VirtanenSMFeskensEJRasanenLFidanzaFTuomilehtoJGiampaoliS Comparison of diets of diabetic and non-diabetic elderly men in Finland, The Netherlands and ItalyEur J Clin Nutr2000541816
- BodenG. Free fatty acids – the link between obesity and insulin resistanceEndocr Pract200174451
- PerseghinGGhoshSGerowKShulmanGI. Metabolic defects in lean nondiabetic offspring of NIDDM parents: a cross-sectional studyDiabetes19974610019
- GrecoAVMingroneGGiancateriniAMancoMMorroniMCintiS Insulin resistance in morbid obesity: reversal with intramyocellular fat depletionDiabetes20025114451
- MingroneGDeGaetanoAGrecoAVCapristoEBenedettiGCastagnetoM Reversibility of insulin resistance in obese diabetic patients: role of plasma lipidsDiabetologia199740599605
- MingroneGRosaGDi RoccoPMancoMCapristoECastagnetoM Skeletal muscle triglycerides lowering is associated with net improvement of insulin sensitivity, TNF-alpha reduction and GLUT4 expression enhancementInt J Obes Relat Metab Disord200226116572
- MingroneGMancoMGranatoLCalvaniMScarfoneAMoraEV Leptin pulsatility in formerly obese womenFASEB J20051913802
- RosaGDi RoccoPMancoMGrecoAVCastagnetoMVidalH Reduced PDK4 expression associates with increased insulin sensitivity in postobese patientsObes Res20031117682
- MingroneGHenriksenFLGrecoAVKroghLNCapristoEGastaldelliA Triglyceride-induced diabetes associated with familial lipoprotein lipase deficiencyDiabetes199948125863
- Guidone C, Manco M, Valera-Mora E, Iaconelli A, Gniuli D, Mari A, . Mechanisms of recovery from type 2 diabetes mellitus after malabsorptive bariatric surgery. Diabetes 2006; 55: 2025–31.
- Mari A., Manco M, Guidone C, Nanni G., Castagneto M, Mingrone G, . Restoration of normal glucose tolerance in severely obese patients after bilio-pancreatic diversion: role of insulin sensitivity and beta cell function. Diabetologia 2006; 49: 2136–43.