ABSTRACT
Introduction
Persistent and excess IL-6 production often contributes to a variety of immune diseases. IL-6–targeting therapy was first approved for Castleman disease, then rheumatoid arthritis, and now it is broadly used. Furthermore, it has been approved not only for chronic and acute inflammatory diseases but also for autoantibody-induced diseases such as neuromyelitis optica spectrum disorder and interstitial lung disease due to systemic sclerosis.
Areas covered
This review summarizes laboratory changes after IL-6 inhibition and molecular changes in the immune cell. A wide range of diseases is discussed, from those for which the efficacy of IL-6–targeting therapy is approved worldwide to those for which only a small number of clinical cases have been reported. It summarizes the characteristics of the diseases for which IL-6–targeting therapy is expected to be effective.
Expert opinion
Clinical evidence has demonstrated that IL-6–targeting therapy is relatively safe; however, it is necessary to be cognizant of early detection of infectious disease, as inhibition of IL-6 masks inflammation. Although many of the cases for which IL-6–targeting therapy was effective or partially effective still require validation through clinical trials, the therapy is suggested as a candidate treatment option for various refractory immune diseases.
1. Introduction
The cytokine is a humoral factor responsible for signaling between cells to maintain homeostasis. Its function has been studied in vitro through cell stimulation experiments and protein interactions, or in vivo using genetically engineered mice and disease model mice. Accumulating evidence has revealed that cytokines play a central role in the pathophysiology of various diseases, and has led to the development of pharmacotherapy for intractable diseases that targets specific cytokines using biologic drugs [Citation1]. With the spread of cytokine-targeting therapies, the relationship between disease activity and cytokines and the function of cytokines in pathological conditions have been elucidated. IL-6 had originally been studied as a cytokine that was produced by T cells and that induced the differentiation of B cells to antibody production cells [Citation2,Citation3]. Following this, it became clear that IL-6 was involved not only in the regulation of the immune system but also in the regulation of hematopoietic and metabolic systems. IL-6 transgenic mice show splenomegaly, lymph node swelling, polyclonal increases in IgG1, and thrombocytosis similar to that observed in chronic inflammatory diseases [Citation4], whereas IL-6–deficient mice develop normally but show an impaired immune and acute-phase response [Citation5]. Clinically, IL-6 has been shown to correlate with disease activity in a variety of chronic inflammatory diseases and to play a central role in the pathophysiology of chronic inflammation, leading to the development of IL-6 inhibitors [Citation6,Citation7]. Anti–IL-6 receptor (IL-6 R) antibody, tocilizumab (TCZ), was first approved for Castleman disease, which is a lymphoproliferative disease accompanied by IL-6 overproduction and inflammation. Subsequently, TCZ was approved for rheumatoid arthritis (RA) and has been widely used in clinical practice around the world. It was then approved for juvenile idiopathic arthritis (JIA), giant cell arteritis (GCA), Takayasu arteritis, cytokine release syndrome (CRS) associated with chimeric antigen receptor–expressing T (CAR-T) cell therapy, adult-onset Still’s disease (AOSD), neuromyelitis optica spectrum disorder (NMOSD), and systemic sclerosis (SSc)-associated interstitial lung disease, as well as for coronavirus disease (COVID-19) in hospitalized patients [Citation8,Citation9]. In these immune diseases, the symptoms, organs attacked, and type of onset (chronic or acute) are different for each, but many of the changes in the human body caused by inhibiting IL-6 are similar. If IL-6–targeting therapy is administered properly and with appropriate monitoring, there is no serious concern about its safety. Laboratory abnormalities associated with inflammation normalize promptly with inhibition of IL-6. The elevated levels of acute-phase proteins, fibrinogen, and IgG concentrations normalize, and hematological parameters such as platelet counts normalize, inflammatory anemia improves, and neutrophil count is reduced. Furthermore, fever resolves, and swelling and pain associated with the inflamed lesion are relieved [Citation10]. Here, we summarize the changes that occur following IL-6 inhibition in humans and list the diseases for which IL-6–targeting therapy has been approved or shown effective in clinical trials and case reports. We propose disease categories in which the effectiveness of IL-6–targeting therapy can be expected, and point out what should be considered when IL-6–targeting therapy is not sufficiently effective.
2. IL-6 and the IL-6 receptor
2.1. IL-6–producing cells
IL-6 consists of a glycosylated four-helix bundle structure [Citation2]. It is produced by inflammatory lesions and transduces signals throughout the entire human body through the bloodstream by the stimulation of TLRs on immune cells including monocytes, macrophages, neutrophils, dendritic cells [Citation11], activated B cells, and T cells [Citation12,Citation13]. It is also produced by non-immune cells including fibroblasts, keratinocytes, astrocytes, endothelial cells, and neurons after stimulation [Citation14,Citation15]. The cells that produce IL-6 vary from disease to disease. Profiling analysis of fibroblasts, monocytes, T cells, and B cells from synovial tissue of RA patients by scRNA-seq showed that the majority of cells to produce IL-6 were naive B cells and sublining THY1+ fibroblasts with a high level of HLA-DRA (HLA class II DR alpha chain) [Citation16]. In Castleman disease, IL-6 is also known to be produced by B cells in the germinal center of the hyperplastic lymph nodes in the lesion [Citation17].
GCA is a medium- and large-vessel vasculitis that mainly affects the cranial arteries and aorta of patients older than 50 years. IL-6 levels are associated with the disease activity of GCA. In the arterial granulomas of GCA, IL-6–producing cells are distributed in the intima, media, and adventitia, but especially in the media that is in contact with the internal elastic lamina. Most IL-6–producing cells are macrophages in the media, but fibroblasts also produce IL-6 in the intima. Neither endothelial cells nor giant cells express IL-6 [Citation18].
AOSD is a systemic inflammatory disease characterized by a spiking fever, skin rash, hepatosplenomegaly, and arthritis which is thought to be the adult form of systemic JIA due to similarity in symptoms. Neutrophilia and elevated ferritin levels are often observed. Overproduction of pro-inflammatory cytokines including IL-18, IL-1β, TNFα, and IL-6 is thought to be the pathogenesis of AOSD [Citation19]. Previous studies have pointed to the role of monocytes in the production of IL-6; however, recent analyses have shown low-density granulocytes, a subset of pro-inflammatory neutrophils in circulation, to be a primary source of IL-6 [Citation20].
Neuromyelitis optica spectrum disorder (NMOSD) is a rare autoimmune disease that preferentially affects the spinal cord and optic nerve, causing severe flares and leading to permanent neuropathy [Citation21]. In patients with NMOSD, it has been shown that IL-6 is produced in monocytes, especially CD14+CD16++ non-classical monocytes in peripheral blood mononuclear cells [Citation22]. Astrocytes also produce IL-6 by binding AQP4-IgG to their AQP4 channel and stimulating this with inflammatory cytokines [Citation21].
Artificial CAR-T cell therapy is becoming a widely used therapy that interacts with specific targets on cancer cells to kill them. This therapy is always accompanied by inflammatory toxicities, and some of these develop into severe systemic inflammation called cytokine release syndrome (CRS) with high levels of GM-CSF, IL-6, and IL-1β. In this case, IL-6 is not primarily produced by CAR-T cells but is produced by monocytes which are stimulated by GM-CSF from CAR-T cells [Citation23].
A worldwide outbreak of severe acute respiratory syndrome coronavirus 2 (SARS-CoV-2) induced coronavirus disease 2019 (COVID −19). IL-6 levels correlated with disease severity in COVID-19. In peripheral blood, a significantly higher expression of IL-6 was secreted from the pathogenic CD45+CD3+CD4+ T cells and CD14+CD16+ inflammatory monocytes. Particularly in serious cases, these cells can exacerbate COVID-19 and increase mortality through a cytokine storm [Citation24]. IL-6 signaling on endothelial cells forms an inflammatory circuit for further robust production of IL-6 [Citation25].
2.2. IL-6 receptor system
IL-6 signaling involves a cascade of binding events (). IL-6 first binds to its receptor IL-6 R, and subsequently, the IL-6/IL-6 R complex binds to the signal-transducing molecule gp130 forming a gp130-homodimer, resulting in an IL-6(2)/IL-6 R(2)/gp130(2) hexamer structure with two molecules each [Citation26]. The homodimerization of gp130 activates Janus kinases (JAKs) and transduces an intracytoplasmic signal. gp130 is involved in the signal transduction of the IL-6 family of cytokines including oncostatin M (OSM), leukemia inhibitory factor (LIF), ciliary neurotrophic factor (CNTF), cardiotrophin 1 (CT-1), cardiotrophin-like cytokine factor 1 (CLCF1), IL-11, IL-27, IL-35, and IL-39, as well as IL-6 [Citation27,Citation28].
Figure 1. IL-6, its receptor system, and inhibitors at each stage of receptor complex formation. IL-6 has a four-bundle (from A to D) structure, interacting with the IL-6 receptor (IL-6 R) at site I and with gp130 at site II and site III. First, IL-6 binds to IL-6 R, the IL-6/IL-6 R complex binds to gp130, and finally a hexamer structure is formed. IL-6 signaling via membrane IL-6 R or soluble IL-6 R is called classic signaling or trans-signaling, respectively. Red arrows indicate signal transduction. Antibodies that inhibit the binding of IL-6 to IL-6 R, sgp130-Fc that inhibits the binding of IL-6/sIL-6 R complex to gp130, and antibodies that inhibit hexamer formation are surrounded by black squares. The antibodies listed in bold letters are approved for a variety of diseases.
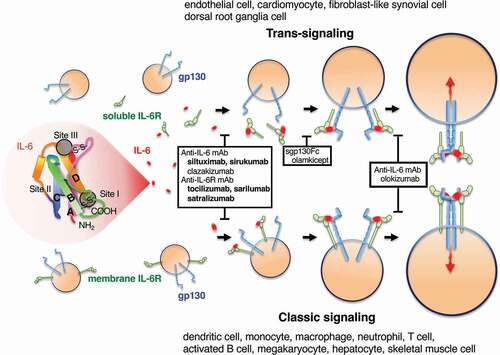
Human IL-6 has three critical sites involved in receptor interaction for IL-6 signaling: site I (on helix D, also including parts of the AB loop) interacts with IL-6 R; site II (on residues from helix A and C) interacts with one gp130; and site III (on the n-terminal end of helix D) is also responsible for interaction with another gpl30 [Citation26]. Two cases of elderly patients with anti–IL-6 autoantibodies reported normal levels of CRP despite bacterial infection [Citation29]. The epitope of anti–IL-6 autoantibodies from these cases was the region containing tryptophan157 of IL-6 that is located at IL-6 site III and important for binding with gp130.
IL-6 R has two forms, membrane IL-6 R (mIL-6 R) and soluble IL-6 R (sIL-6 R), which are shed from the cell’s surface by ADAM metalloprotease 17 (ADAM17) and are also created by alternative mRNA splicing. The IL-6/mIL-6 R or IL-6/sIL-6 R complex–mediated activation of gp130 is called classic signaling or trans-signaling, respectively () [Citation30]. These two signaling modes differ from cell to cell. IL-6 can also be presented from an mIL-6 R–expressing cell to a gp130-expressing cell if both cells are in close proximity. This is called trans-presentation and is observed between dendritic cells and T cells [Citation31]. A soluble form of gp130 (sgp130) also exists in human serum and inhibits IL-6/sIL-6 R–mediated trans-signaling [Citation32]. Increased serum sIL-6 R results in a buffer for IL-6 by IL-6/sIL-6 R/sgp130 complex formation [Citation33].
3. Clinical application of IL-6–targeting therapy
3.1. Background of the first IL-6 inhibitor tocilizumab
After the molecular cloning of human IL-6 and IL-6 R [Citation2,Citation34], murine anti–human IL-6 R mAb PM1 was prepared by immunization with purified human IL-6 R. PM1 inhibited the binding of human IL-6 to human IL-6 R and blocked the IL-6 signal [Citation35]. For the purpose of clinical administration, reshaped human PM1 was designed as a humanized IgG1 class mAb [Citation36]. By that time, the first anti–IL-6 mAb clinical trial case had reported that IL-6 inhibition suppressed the growth of myeloma [Citation37], and reshaped human PM1 was temporarily called myeloma receptor antibody (MRA).
RA is an inflammatory disease in which IL-6 is produced in affected joints with synovitis. When reshaped human PM1 was intravenously injected into patients with severe RA resistant to conventional therapy, it resolved low-grade fever and fatigue within a week, normalized serum CRP, fibrinogen, and ESR levels within 2 weeks, and improved morning stiffness, swollen joint score, pain and tenderness score, as well as anemia, thrombocytosis, hypoalbuminemia, and polyclonal hypergammaglobulinemia [Citation38]. Following this, it was revealed that TCZ (also known as reshaped human PM1, MRA, and atlizumab) exhibits anti-inflammatory activity.
TCZ was first clinically approved for Castleman disease in Japan in 2005 [Citation39]. The continuous production of IL-6 from the affected lymph nodes in Castleman disease was the pathogenesis of systemic inflammation; thus, this disease was the first good candidate for IL-6–targeting therapy. Due to its anti-inflammatory properties, TCZ was also trialed as a therapy for RA. The effectiveness of TCZ in the suppression of disease activity and prevention of bone destruction in RA has been confirmed in many randomized clinical trials [Citation40]. In 2008, TCZ was also approved for RA in Japan, in 2009 in the EU, and in 2010 in the United States. The clinical position of TCZ in the recommendation for the management of RA has changed over the years. Based on a larger body of evidence with TNF inhibitors, the 2010 EULAR recommendations stated that TCZ should be used if a TNF inhibitor has failed [Citation41]. Following this, a comparable safety profile of TCZ compared with TNF inhibitors has been shown, and a head-to-head trial revealed TCZ monotherapy to be superior to monotherapy with the TNFα inhibitor adalimumab [Citation42]. Based on this, the updated 2013 EULAR recommendations stated no preference for one biologic agent over the other [Citation43].
3.2. Dynamics of TCZ and its target molecules
The half-life of TCZ increases with repeated and increased doses. The initial study of the half-life of TCZ after the third dose of 8 mg/kg found that the half-life reached 241.8 ± 71.4 h [Citation44]. The elimination rate of mAb depends on various factors and occurs through specific target antigen-mediated nonlinear clearance and non-specific linear clearance through the cells of the reticuloendothelial system by various Fc receptors. Exceptionally, the neonatal Fc receptor protects IgG from clearance as a salvage receptor. The elimination of TCZ occurs mostly in tissues through the reticuloendothelial system as a non-specific clearance mechanism [Citation45]. TCZ is usually administered by infusion at 4-week intervals for RA, but 2-week intervals are often required for Castleman disease that is characterized by marked splenomegaly and lymphadenopathy. Short-interval administration of TCZ in Castleman disease may reflect the disappearance of TCZ mainly in the reticuloendothelial system. The clearance of TCZ was lower in women than in men and appears to be associated with body size [Citation45].
An analysis of the relationship among IL-6, sIL-6 R, and TCZ in patients with RA indicated that IL-6 levels increased from 58 pg/mL at baseline to 90 pg/mL at day 42 and serum sIL-6 R levels also increased from 28 ng/ml at baseline to a steady state 251 ng/ml at day 42 [Citation46]. More than 95% of sIL-6 R was bound to TCZ when TCZ remained at a serum concentration of 1 μg/mL or greater. CRP decreases to normal levels at TCZ concentrations greater than 1 μg/ml, reflecting the high binding rate of TCZ to sIL-6 R. The increase in serum IL-6 and sIL-6 R concentration after TCZ administration is presumed to be due to inhibition of gp130-mediated clearance of IL-6 and sIL-6 R.
3.3. Other IL-6 specific inhibitors
The second anti–IL-6 R mAb approved for RA is sarilumab (SAR), which is a fully human Ab. In a phase 3 study, SAR with MTX produced significant improvements in patients with RA compared to the placebo with MTX group [Citation47]. Furthermore, SAR monotherapy resulted in greater improvement in disease activity scores than adalimumab [Citation48]. SAR was approved for RA in 2017 in Japan, the EU, and the United States. Molecules that specifically inhibit only the IL-6 trans-signal have also been developed. Olamkicept consists of the extracellular region of gp130 fused with the Fc of human IgG1. It inhibits IL-6 trans-signaling effectively, but does not inhibit classical signaling; thus, it is expected to inhibit inflammation other than that of the acute phase response [Citation49]. TCZ biosimilars, HS628, BAT1806, QX003S, and MSB11456, also have been developed and their effects are being investigated in clinical trials [Citation50–53].
The first approved anti–IL-6 mAb is siltuximab (STX). It is a murine–human chimeric anti–human IL-6 mAb and was approved for idiopathic multicentric Castleman disease in the United States in 2014 [Citation54], but has not been approved for other diseases. Olokizumab (OLK) is a humanized anti–IL-6 mAb that selectively blocks the final formation of the hexamer signaling complex and has a long half-life of approximately 31 days [Citation55]. A phase 3 study in patients with RA showed that the subcutaneous administration of OLK 64 mg once every 2 weeks and once every 4 weeks was effective compared with placebo [Citation56]. OLK was approved for RA in Russia in 2020. Sirukumab (SRK) is a human anti–IL-6 mAb and was shown in phase 3 studies to significantly improve the signs and symptoms of RA that was refractory to anti-TNF drugs [Citation57]. The phase 3 study of SRK in Japanese patients showed no new safety signals [Citation58]; however, the FDA declined to approve SRK because of the imbalance of deaths in patients taking SRK compared with a placebo in another study [Citation59]. The other anti–IL-6 mAbs, clazakizumab (BMS945429, ALD518, humanized mAb), gerilimzumab (ARGX-109, human mAb), PF-423691, OP-R003 (human mAb derived from murine anti–IL-6 mAb elsilimomab), and MEDI5117 (human mAb), are also in different phases of clinical trials to examine their efficacy and safety in patients with various diseases [Citation60,Citation61].
3.4. Changes in clinical parameters after IL-6 inhibition
The most characteristic change in blood tests after IL-6 inhibition is the normalization of levels of acute-phase proteins [Citation62]. When IL-6 activity is sufficiently inhibited, concentrations of CRP and serum amyloid A usually decrease to undetectable levels. The erythrocyte sedimentation rate (ESR) is a routine test for overall inflammation and elevated ESR in RA reflects inflammatory anemia, high levels of acute-phase proteins, fibrinogen, and immunoglobulin – ESR drops to normal levels after TCZ treatment. From the post-operative reports of TCZ-treated patients, TCZ strongly suppresses the increase in CRP after surgery, and fever was milder than that in the control group [Citation63].
Hematological changes are also prominent after IL-6 inhibition [Citation62]. A reduction in neutrophil count with TCZ is thought to result not from cytotoxic effect but rather from increased marginalization of circulating neutrophils into the bone marrow [Citation64]. Pooled data of patients showed that more subjects in the TCZ group compared to the placebo group had grade 1/2 or 3/4 neutrophil counts (TCZ: 28.2%/3.1%; placebo: 8.9%/0.2%) [Citation65]. The occurrence of a grade 3/4 neutrophil decrease was associated with female gender and a baseline neutrophil count < 2 × 109/l.
IL-6 indirectly controls erythropoiesis by stimulating the liver to release hepcidin that binds to, internalizes, and degrades the iron exporter ferroportin on duodenal enterocytes, macrophages, and hepatocytes to decrease serum iron levels [Citation66]. Monitoring of iron-related parameters before and after treatment with TCZ in patients with RA showed that significant improvements in anemia and serum iron levels and reductions in hepcidin were observed within 2 weeks. These effects were more pronounced in the TCZ group than in the TNFα inhibitor group [Citation67]. Relief of inflammatory anemia may lead to improvement of fatigue [Citation68]. A major regulator of thrombopoiesis is thrombopoietin that is constitutively produced in hepatocytes. In inflammatory conditions, IL-6 induces thrombopoietin production in the liver and elevates its plasma levels. IL-6–induced thrombocytosis is inhibited by the neutralization of thrombopoietin, suggesting that thrombocytosis is mediated by thrombopoietin [Citation69].
IL-6 trans-signaling directly induces the receptor activator of NF-КB ligand (RANKL) in fibroblast-like synovial cells [Citation70], and IL-6 transgenic mice show osteopenia with decreased osteoblast and increased osteoclast number and activity [Citation71]. These experiments suggest the involvement of IL-6 in osteoporosis. Clinically, inhibition of IL-6 in RA patients increased the bone formation markers osteocalcin and C-terminal type I procollagen propeptide, and decreased the bone resorption markers urinary pyridinoline and deoxypyridinoline, indicating that the balance of bone metabolism moved toward bone formation [Citation62]. Furthermore, according to the data comparison of TCZ and adalimumab with MTX, TCZ monotherapy showed a more pronounced repair of existing bone erosion than adalimumab with MTX in patients with RA [Citation72].
3.5. Molecular changes in immune cells after IL-6 inhibition
Technological advances have made it possible to analyze clinical specimens such as synovial tissue and peripheral blood in detail regarding changes in molecular and gene expression. Microarray analysis of the RA synovial tissue reported that TCZ treatment decreased the expression of cytokine and chemokine genes (e.g. IL-6, IL-22, CCL8, CCL11, CCL13, CCL19, and CCL20) and T cell activation genes (e.g. IL-23A, IL-7, RAG2, and ZAP70) [Citation73]. The genes up-regulated by TCZ were enriched in transcripts associated with healing processes. In comparison with the anti-CD20 Ab rituximab (RTX), methotrexate (MTX), or the TNFα inhibitor adalimumab, the changes by TCZ correlated with the changes with RTX and MTX therapies but differed from the changes induced by adalimumab in which decreased gene expressions are involved in cell proliferation. Although the molecular changes induced by TCZ and RTX treatment are similar, RNA sequence–based stratification of synovial tissue showed that TCZ was more effective than RTX in B cell–poor groups of synovial tissue [Citation74]. As described previously, the major sources of IL-6 were naive B cells and sublining THY1+ fibroblasts in synovial tissue of RA; sublining fibroblasts or other cells may be the main sources of IL-6 in B cell–poor groups. The effect of IL-6 inhibition on synovial T cells has also been shown by immunohistochemical staining of synovial biopsy samples. TCZ significantly decreased the number of CD3 + T cells but did not affect the number of CD68+ macrophages or CD20 + B cells [Citation75].
Analysis of the gene expression profiles of peripheral blood T cells in RA patients showed a decrease in suppression of cytokine-signaling (SOCS) 1 and 3, Bcl3, Batf, Myc, PIM1 and 3, and AT-rich interactive domain-containing protein 5A (Arid5A) in CD4 + T cells [Citation76]. SOCSs are IL-6–induced negative feedback molecules for IL-6 signaling. Batf and Arid5A are molecules related to Th17 differentiation of T cells, Batf is a critical transcription factor for Th17 differentiation [Citation77], and Aird5A is an IL-6 mRNA–binding protein that stabilizes IL-6 mRNA to enhance IL-6 production and the development of Th17 [Citation78]. In fact, Th17 cells (CD4+ IL-17+) increased in the peripheral blood of patients with active RA; however, TCZ significantly decreased the disease activity associated with a decrease in the rate of Th17 cells [Citation79]. Another report of peripheral blood cells showed the proportion of regulatory T (Treg) cells significantly increased after TCZ treatment [Citation80,Citation81]. The most dynamic increase in Treg cells was observed in the clinical disease activity index remission group. These reports suggest that TCZ treatment reduces the elevated Th17/Treg ratio observed in peripheral blood T cell differentiation in RA [Citation82].
The gene expression profile in peripheral blood associated with disease remission of RA was also investigated. Remission was predicted to be more likely in patients with high B cell–related gene expression signatures such as B cell count and B cell function after TCZ treatment [Citation83]. IL-6 was originally investigated as a B cell–stimulating factor and induces immunoglobulin-producing plasma cells [Citation3]. Furthermore, a subset of B cells, especially memory B cells, were reported to decrease after TCZ treatment in patients with RA [Citation84]. These results indicate a relationship between TCZ response and the signature of B cells.
Profiling of peripheral blood in RA patients who show a good response to TCZ reported that most gene transcripts after TCZ treatment were altered in the direction toward the healthy state and the changes were mainly observed in neutrophils, suggesting that the characteristics of RA-related neutrophil changes are more likely to be normalized by TCZ [Citation85]. Peripheral cells in RA patients were characterized by an increase in neutrophils and a decrease in NK cells, but TCZ also influenced these profiles in the direction of the healthy state. In clinical settings, a more than 20% reduction in neutrophil counts 1 month after initiating TCZ predicted clinical remission within 1 year [Citation86], supporting the importance of the change of neutrophil signature for the effect of TCZ on RA. Proteins involved in complement pathways were enriched in the proteins affected by TCZ [Citation85].
In patients with RA with 7.8 years median disease duration who improved to remission with TCZ monotherapy, only 13.4% of patients maintained low disease activity at 52 weeks after the discontinuation of TCZ. The rate of low disease activity reached 38.0% at 52 weeks in patients with low levels of serum IL-6 (less than 12.9 pg/mL) and normal MMP-3 levels [Citation87]. This suggests that TCZ may be discontinued when IL-6 is no longer produced. After the efficacy of IL-6–targeting therapy for RA has been established, it will then be important to determine how to improve the remission rate and increase the rate of drug-free remission.
3.6. Differences between congenital loss of IL-6 R and anti–IL-6 R Ab treatment
The observation of the congenital loss of the IL-6 receptor is helpful to understand the role of IL-6 in humans. Two patients with homozygous mutations in IL6R gene were reported [Citation88]. The patients lacked an adequate inflammatory response, demonstrated a lack of erythema, and experienced barely elevated fevers even with abscesses. Acute-phase proteins were not elevated despite high elevations in serum IL-6 levels. They suffered from recurrent bacterial infections in the sinuses, lungs, and deep skin, but no significant viral infections were noted. They also suffered from allergic symptoms such as asthma and moderate atopic dermatitis. Serum levels of IgG, IgA, and IgM were mildly reduced, but levels of IgE were high. Infectious episodes were characterized more markedly by eosinophilia than neutrophilia. Immunophenotyping revealed an elevated proportion of CD4 T cells expressing Th2-associated GATA3, an elevated proportion of FOXP3 Treg cells, and a reduced number of class-switched memory B cells. Immunoglobulins included a higher proportion of IgE and IgG4.
Both patients with congenital IL-6 R abnormalities and patients receiving anti–IL-6 R inhibitory mAb TCZ have similar poor acute response and reduced fever response, with some differences (). Allergic symptoms are seen in patients with congenital IL-6 R deficiency but not in adult patients receiving TCZ. Clinical studies and post-marketing surveillance of TCZ have not reported an increase in allergic symptoms [Citation42,Citation89], and TCZ was even reported to improve skin symptoms in cases of intractable atopic dermatitis with high IgE and eosinophilia [Citation90]. Eosinophilia induced by TCZ is a rare complication [Citation91]. A 20-month-old child with anti–IL-6 autoantibodies who presented with sepsis without an increase in CRP was reported to have a mild decrease in IgG and an increase in IgE, but eosinophil counts were normal [Citation92]. The difference between congenital loss of IL-6 R and IL-6 R inhibition therapy after growth might be due to the different timing of IL-6 signal loss – the congenital loss of IL-6 signal from the early stage of life and the inhibition after growing – or might be due to the difference between complete IL-6 signal loss by genetic mutation and incomplete inhibition by pharmacotherapy or autoantibody. Bacterial infections are repeated in congenital IL-6 R disorders, but the incidence of serial infectious adverse events in patients receiving IL-6 R inhibition therapy is 4.7–9.09/100 patient-years [Citation89,Citation93]. The low rate of serious infections in IL-6 R inhibition therapy may be due in part to regular visits to a clinic, early detection, appropriate treatment of infections, and discontinuation of IL-6 R inhibition therapy when infections are suspected.
Table 1. Comparison of congenital loss of IL-6 receptor with IL-6 receptor inhibition therapy.
4. Diseases for which IL-6–targeting therapy has been approved following approval for RA
4.1. Systemic juvenile idiopathic arthritis (sJIA)
After the efficacy of IL-6 inhibitory therapy was established for Castleman disease and RA, IL-6 inhibitory therapy was attempted for some refractory inflammatory diseases. sJIA is a chronic childhood arthritis with symptoms including fever, skin rash, pericarditis, hepatosplenomegaly, and growth retardation. It is often treated with glucocorticoids, but the long-term use of glucocorticoids can potentially cause growth impairment. IL-6 levels are correlated with the severity of disease manifestation of sJIA. A randomized phase 3 trial of TCZ in children with refractory sJIA showed drastic improvement in disease activity [Citation95]. TCZ also restored body growth and improved bone homeostasis [Citation96,Citation97]. TCZ was approved for sJIA treatment in 2008 in Japan and in 2011 in the EU and the United States.
4.2. Giant cell arteritis (GCA) and Takayasu arteritis (TA)
The safety and efficacy of TCZ for RA encouraged clinical trials of TCZ for diseases other than inflammatory joint diseases. A phase 3 GiACTA study of patients with large vessel vasculitis GCA showed that those receiving TCZ plus prednisone led to a reduction in the cumulative dose of prednisone required to regulate GCA compared to those taking prednisone alone [Citation98]. In 2017, TCZ became the first GCA therapy to be approved by the United States, Japan, and the EU.
TA primarily affects women under the age of 40. Arteries and their major branches narrow or develop aneurysms due to chronic inflammation of the vessel walls. In a phase 3 TAKT study, TCZ did not meet the defined primary endpoint for time to relapse of TA; however, the hazard ratio for time to relapse was 0.41 (95.4% CI 0.15–1.10; p = 0.0596) compared to placebo, suggesting a favorable effect of TCZ[Citation99]. Another report of 46 patients with TA also showed significantly better event-free survival of patients who received TCZ[Citation100]. Japan approved TCZ for the treatment of TA in 2017.
4.3. Adult-onset Still’s disease (AOSD)
A meta-analysis of 10 original studies with 147 patients with AOSD showed promising outcomes for TCZ in AOSD [Citation101]. In a phase 3 trial of AOSD refractory to glucocorticoids, although the primary endpoint of ACR50 response at week 4 was not significantly different from the placebo group, the least-squares means for change in systemic feature score were −4.1 in the TCZ group and −2.3 in the placebo group (p = 0.003) at week 12. The dose of glucocorticoid needed also significantly decreased in the TCZ group compared to the placebo group. This study suggests that TCZ is effective in AOSD [Citation102]. Japan approved TCZ for the treatment of AOSD in 2019.
5. Diseases for which IL-6–targeting therapy is newly approved
5.1. Approval for severe acute inflammatory diseases
Clinical trials examining IL-6–targeting therapy in acute inflammatory conditions started after those for chronic inflammatory diseases. IL-6–targeting therapy was first tried in the treatment of severe CRS in CAR-T therapy and bispecific T cell–engaging antibody therapy for B cell leukemia or lymphoma. In CRS, high levels of IL-6 activate the coagulation pathway leading to thrombosis and also activate vascular endothelial cells leading to vascular leakage. Furthermore, IL-6 inhibits myocardial function leading to tissue hypoxia, hypotension, and multiple organ failure [Citation103]. TCZ demonstrated remarkable effects on severe systemic inflammatory response as well as a reduction in elevated serum cytokine levels [Citation104]. TCZ was approved for CRS induced by CAR-T therapy in the United States in 2017, the EU in 2018, and Japan in 2019.
Because of the effects of IL-6 on the immune system, it was thought that IL-6–targeting therapy should not be given when the patient has an infectious disease; however, the historic pandemic of SARS-CoV-2 infection, starting in December 2019, and its clinical syndrome COVID-19 unexpectedly opened a new indication of IL-6–targeting therapy. Severe COVID-19 has a high mortality rate, mainly in the elderly, accompanied by fever, lymphopenia, and pneumonia leading to the most severe complication of acute respiratory distress syndrome (ARDS) with cytokine storm [Citation105]. IL-6 levels correlated with disease severity and contributed to pathological conditions such as highly elevated inflammatory markers, hyper-vascular permeability, and hyper-coagulability observed in COVID-19 [Citation106,Citation107]. IL-6 trans-signaling on endothelial cells induces robust production of IL-6, IL-8, MCP-1, and the inhibitor of fibrinolysis, plasminogen activator inhibitor-1 (PAI-1), to promote further inflammation and coagulation [Citation25]. Randomized trials of TCZ in patients with COVID-19 have led to conflicting results [Citation108]; however, a meta-analysis including 23 studies with a total of 6,279 patients indicated the overall mortality was lower in the TCZ group compared to the standard care group [Citation109]. Subgroup analysis including studies with only severe cases revealed lower mechanical ventilation needs and mortality in the TCZ group compared to the control group [Citation109]. Another meta-analysis with 13,412 patients with COVID-19 also supported a reduction in the mortality rate from COVID-19 by TCZ [Citation110]. TCZ was approved for COVID-19 in the United States and the EU in 2021 and Japan in 2022. Many clinical trials with anti–IL-6 mAbs have also investigated the efficacy and safety in the treatment of COVID-19 [Citation111].
5.2. Approval and recommendations for autoantibody-induced diseases
The effectiveness of TCZ has also been shown in diseases caused by autoantibodies. Neuromyelitis optica spectrum disorder (NMOSD) is an autoimmune disease that is characterized by local inflammation mainly affecting the optic nerve, spinal cord, and possibly the brain stem and cerebrum, presenting with symptoms such as visual impairment, paralysis, sensory loss, bladder dysfunction, nausea, and vomiting [Citation112]. Unlike other systemic inflammatory diseases such as arthritis and vasculitis, auto-Ab–positive NMOSD is thought to be caused by the production of anti–aquaporin-4 (AQP-4) Ab, which attacks astrocytes by activating complement. An in vitro study showed that IL-6 promoted the differentiation of B cells into anti–AQP-4-IgG–producing plasmablasts and enhanced the survival of plasmablasts [Citation113]. A meta-analysis of five clinical trials showed a significant correlation between relapse-free NMOSD and TCZ therapy [Citation114]. Satralizumab (STZ) is an engineered Ab from TCZ and binds to IL-6 R in plasma (pH 7.4), but releases IL-6 R in the endosome (pH 6.0), allowing it to be recycled in plasma and enabling a long half-life compared with TCZ [Citation115]. Treatment with STZ led to a lower risk of relapse than placebo in anti–AQP-4 Ab–positive NMOSD but not in Ab-negative NMOSD [Citation116], suggesting the beneficial effect of IL-6–targeting therapy on autoantibody-mediated diseases. STZ was approved in Japan and the United States in 2020 and the EU in 2021.
Graves’ ophthalmopathy is associated with swelling of the eyelids, exophthalmos, and double vision due to swollen extraocular tissues. It is thought to be caused by anti–thyrotropin-receptor autoantibodies [Citation117]. Orbital fibroblasts are activated to secrete IL-6 by autoantibodies against the thyrotropin receptor [Citation118,Citation119]. In a phase 3 trial, TCZ improved activity and severity in corticosteroid-resistant Graves orbitopathy and has been shown to be effective [Citation119]. The 2021 European Group on Graves’ orbitopathy (EUGOGO) clinical practice guidelines recommended TCZ as second-line treatment for Graves’ orbitopathy [Citation120].
Anti–N-methyl-D-aspartate receptor (NMDAR) encephalitis is an autoimmune encephalitis that predominantly affects children and young adults [Citation121]. Patients progress from psychosis, memory deficits, seizures, and language disintegration into a state of unresponsiveness. Forty percent of the cases occur with tumor association which is usually an ovarian teratoma. Anti-NMDAR Ab is thought to be pathogenic for the clinical features of this encephalitis. A cohort study showed the efficacy of TCZ in refractory patients to RTX [Citation122], and an international consensus group recommends TCZ if the first-line (steroid, intravenous immunoglobulin, and plasma exchange) and second-line treatments (cyclophosphamide or RTX) are not effective [Citation123].
5.3. Approval for systemic sclerosis–induced interstitial lung disease
Systemic sclerosis (SSc) is an intractable connective tissue disease with fibrosis in the skin, lung, or gastrointestinal tract, and life-threatening heart and kidney complications. Interstitial lung disease associated with SSc is the leading cause of death in patients, and there are few effective treatments [Citation124]. The role of osteopontin (OPN) in the progression of interstitial pneumonia has been reported [Citation125]. Immune complexes activate macrophages and produce OPN via IL-6, then OPN promotes fibroblast recruitment and contributes to the progression of fibrosis. Serum OPN levels increased in patients with SSc and predicted disease progression; however, TCZ treatment decreased OPN levels. Two randomized controlled trials showed TCZ to have a significant lung preservation effect in patients with SSc [Citation126,Citation127]. In both trials, there was nonsignificant reduction of skin sclerosis from baseline to week 48 in modified Rodnan skin scores between the TCZ and control groups; however, the decrease of the percentage of predicted forced vital capacity (FVC% predicted) at week 48 was significantly smaller in the TCZ group than the control group. The effects of TCZ differed between interstitial lung disease and skin lesions in SSc, but it is unclear whether the evaluation method of skin sclerosis could not sufficiently detect the effectiveness of TCZ, whether the treatment timing was too late, or whether skin sclerosis and lung lesions are caused by different mechanisms. TCZ was approved for SSc-induced interstitial lung disease in the United States in 2021.
6. Diseases expected to be effective in IL-6–targeting therapy
6.1. Diseases for which IL-6–targeting therapy was effective in randomized controlled trials
6.1.1. Potential for reduction in myocardial infarction
There are several diseases for which a significant effect of IL-6–targeting therapy has been observed by randomized controlled trials. IL-6 inhibition therapy for cardiovascular events raises two questions; whether it prevents cardiovascular events and whether it has a protective effect against cardiac damage after myocardial infarction. Inflammation plays an important role in accelerating atherosclerosis. For example, the risk of cardiovascular disease is increased by 48% in patients with RA [Citation128], and TNFα inhibitor and MTX treatments are associated with a lowered risk of cardiovascular disease in patients with RA [Citation129]. Although TCZ increases levels of total cholesterol and LDL cholesterol, it reduces lipoprotein (a) and oxidized LDL [Citation130], and measurement of intracellular cholesterol content in human macrophages indicated that TCZ reduced cholesterol loading capacity and improved cholesterol efflux capacity [Citation131]. A meta-analysis of 14 observational studies in patients with RA showed that TCZ was associated with a decreased risk of major adverse cardiovascular events (OR 0.59 [95% CI 0.34–1.00]) [Citation132].
IL-6 also plays a role in the pathology of acute myocardial infarction. Its level rapidly increases after myocardial infarction and is associated with worse prognosis [Citation133]. In the first phase 2 trial of TCZ for non–ST-elevation myocardial infarction (which characteristically has elevated levels of troponin, ST depression, or T-wave inversion but no ST elevation in ECG), the median area under the curve for troponin T was lower in the intravenous TCZ group than in the placebo group [Citation134]. This difference was observed mainly in patients included within 2 days of onset and those treated with percutaneous coronary intervention. However, in the second trial, no change in major adverse cardiac events was observed among patients who received a single dose of TCZ subcutaneously [Citation135]. It is necessary to consider the method of TCZ administration, evaluation parameters, and selection of patients to confirm the effects of TCZ on myocardial infarction. Patients experiencing out-of-hospital cardiac arrest with comatose status after initial resuscitation are at high risk of mortality from post-cardiac arrest syndrome. Systemic inflammation is a major component of post-cardiac arrest syndrome, and IL-6 levels are associated with this syndrome severity. A phase 2 randomized trial showed that TCZ significantly reduced systemic inflammation and myocardial injury in comatose patients resuscitated from out-of-hospital cardiac arrest [Citation136].
6.1.2. Potential use for polymyalgia rheumatica
Polymyalgia rheumatica is an inflammatory disease associated with stiffness of the shoulder and pelvic girdle. In a phase 2/3 trial in patients with new-onset polymyalgia rheumatica undergoing rapid glucocorticoid tapering, TCZ was superior to placebo in terms of sustained glucocorticoid-free remission and time to relapse [Citation137]. TCZ decreased the cumulative glucocorticoid dose, as was also observed in another clinical trial of large vessel vasculitis in which TCZ reduced the cumulative dose of glucocorticoid required [Citation98].
6.1.3. Potential for reduction in graft inflammation with renal transplantation
In a phase 2 randomized open-label trial, 48 renal transplant recipients with subclinical graft inflammation received either usual immunosuppression or monthly TCZ for 6 months with usual immunosuppression [Citation138]. TCZ treatment was more likely to show improved Banff ti score of kidney biopsy sample (62.5% vs. 21.4%, p = 0.03) and increased Treg frequency. Several clinical trials of TCZ have been conducted for the rejection of kidney transplantation.
6.1.4. Potential for reduction in depression
RA associates with an increased risk of psychiatric disorders, such as depression, anxiety disorder, and bipolar disorder [Citation139]. A meta-analysis of patients with depression showed that the inflammatory markers TNFα, IL-1, IL-6, and CRP are associated with depression [Citation140]. IL-6 inhibition by TCZ significantly decreased anxiety and depression levels based on the Hamilton Depression (HDRS) and Anxiety (HAMA) scores in patients with RA [Citation141]. The phase 3 trial to test the efficacy of olokizumab (OKZ) in RA showed marked improvement in SF-36 mental component scores at week 24 [Citation56]. The data obtained from two phase 2 trials of sirukumab in RA or of siltuximab in Castleman disease were analyzed post hoc[Citation142]. IL-6 inhibition therapies achieved significant improvement in questionnaire items related to depressed mood and anhedonia. These trials evaluated IL-6–targeting therapy in psychiatric disorders associated with inflammatory diseases, and further clinical trials are needed to confirm its efficacy in psychiatric disorders without inflammatory diseases.
6.2. Case series and case reports showing the effectiveness of IL-6–targeting therapy
We searched PubMed from February 2003 to May 2022 to collect case series and case reports on TCZ, the earliest approved IL-6 inhibitor used worldwide. The diseases previously mentioned in this chapter were excluded. Some case series have indicated the efficacy of IL-6–targeting therapy, for example in amyloid A amyloidosis[Citation143], various symptoms of Behçet’s disease [Citation144], relapsing polychondritis [Citation145], steroid-refractory immune-mediated adverse events secondary to immune checkpoint inhibitors [Citation146], pre-engraftment syndrome after unrelated cord blood transplantation [Citation147], pediatric anti-NMDAR Ab encephalitis [Citation123], and myelin oligodendrocyte glycoprotein immunoglobulin G (MOG-IgG) associated disorder (MOGAD) [Citation148]. IL-6 inhibition also has the effect of reducing glucocorticoid doses by suppressing inflammation in refractory inflammatory diseases such as VEXAS syndrome [Citation145]. Furthermore, IL-6 inhibition has been tried as salvage therapy in many intractable diseases that cannot be effectively treated with conventional treatments. Many cases with good results have been reported (); however, these cases may only report selected patients with signs and symptoms that improve with IL-6 inhibition. Although randomized clinical trials are essential to confirm the benefits of IL-6–targeting therapy, this therapy may be considered one of the treatment options for refractory conditions involving the immune system.
Table 2. Indications for IL-6–targeting therapy, approved diseases, phases of clinical trials, and case reports. Case reports excluded approved diseases and diseases for which randomized trials or case series showed the efficacy of IL-6–targeting therapy. TCZ: tocilizumab (anti–IL-6 R mAb); SAR: sarilumab (anti–IL-6 R mAb); STZ: satralizumab (anti–IL-6 R mAb); STX: siltuximab (anti–IL-6 mAb); SRK: sirukumab (anti–IL-6 mAb); CLZ: clazakizumab (anti–IL-6 mAb); OKZ: olokizumab; (anti–IL-6 mAb); OLA: olamkicept (soluble gp130-Fc fusion protein. TJ301); NMDAR; N-methyl-d-aspartate receptor; GVHD: graft-versus-host disease.
6.3. Diseases for which IL-6–targeting therapy was not effective in randomized controlled trials
6.3.1. TCZ or SAR study for ankylosing spondylitis did not achieve response criteria
Ankylosing spondylitis is a gradually progressive inflammatory disease affecting the axial skeleton, peripheral joints, and entheses. Elevated levels of serum IL-6 correlate with disease activity. In randomized controlled trials of TCZ or SAR for ankylosing spondylitis, IL-6 inhibition decreased CRP levels but failed to achieve the primary end point meeting the axial spondyloarthritis international society (ASAS) 20 response criteria at week 12 [Citation149,Citation150]. An assessment of the local inflammatory activity of enthesitis and whether the observation at 12 weeks was appropriate for this slow-progressing disease should be considered.
6.3.2. Clazakizumab study in psoriatic arthritis and TCZ study in acute GVHD
In the phase 2b randomized trial in patients with psoriatic arthritis, anti–IL-6 Ab clazakizumab treatment significantly improved musculoskeletal manifestations (joint symptoms, enthesitis, and dactylitis) compared with placebo but lacked a dose response and minimal improvements in skin disease [Citation151].
The efficacy of TCZ in preventing acute graft-versus-host disease (aGVHD) in patients with acute leukemia or myelodysplasia who are undergoing allogeneic stem cell transplantation has been studied. In a phase 3 trial, TCZ showed nonsignificantly reduced incidence of grade 2–4 aGVHD in recipients from HLA-matched unrelated donors but no improvements in long-term survival [Citation152].
7. Safety profile of IL-6 inhibition therapy
7.1. Risk for infectious disease
There are many reports on safety as well as efficacy of TCZ in patients with RA. The incidence of serious infections with TCZ administration is almost comparable to that of other biologics. In a British study of 19,282 patients’ data, TCZ has a slightly but significantly higher risk of serious infection (HR = 1.22, 95% CI 1.02–1.47) compared with etanercept [Citation153]; however, a study in the United States with 31,801 new biologic treatment episodes showed no difference in the rate of hospitalized infection with TCZ compared with abatacept and slightly higher HRs for hospitalized infection with etanercept, infliximab, and RTX [Citation154].
Risk factors for serious infections included a concurrent or past history of respiratory disorders, current treatment with prednisolone ≥ 5 mg/day, and age ≥ 65 years in a post-marketing surveillance of 3,881 patients with RA in Japan [Citation89]. Risk factors for serious infections in 1,491 patients with RA in the French REGistry included initial high DAS28 score, concomitant leflunomide use, neutrophil count greater than 5.0 × 109/l, and negative anti–citrullinated peptide antibodies [Citation93]. Decreased neutrophils are often observed after TCZ administration; however, rates of serious infections within 30 days of normal, grade 1/2, and 3/4 neutrophil counts were similar, suggesting that decreased neutrophil counts during TCZ treatment in RA did not appear to be associated with serious infections [Citation65].
7.2. Increased risk of intestinal perforation
IL-6 is involved in the protection of intestinal epithelial cells [Citation155,Citation156]; thus, concerns have been pointed out about complications in the intestinal mucosa. According to the German Biologics Register, TCZ led to an increase in the incidence (2.7/1,000 patient-years) of lower intestinal perforation in patients with RA, with a hazard ratio of 4.5 compared to conventional synthetic disease-modifying antirheumatic drugs (DMARDs) [Citation157]. Patients with a history of diverticulitis require careful observation when receiving IL-6 inhibition therapy. When administering IL-6 inhibitors, inflammatory markers such as CRP do not increase or only mildly increase; thus, attention is needed to observe for signs of abdominal inflammation by intestinal perforation. It may be safer to avoid treatment in elderly patients with RA with a history of diverticulitis.
7.3. Pregnancy and lactation
The safety of TCZ in pregnancy has not been established because of limited data. Although the data are small, there have been no reports of safety concerns to date. In Germany, 15 maternal cases recorded 4 spontaneous abortions and 11 live-born infants with no congenital malformations [Citation158]. In Chugai’s TCZ safety database in Japan, 50 pregnancies exposed to TCZ were reported and the spontaneous abortion rate was 18.0%. This rate is comparable to the rate in the general population and no congenital anomalies were identified in 36 births [Citation159]. From the Roche Global Safety Database, 180 pregnancies exposed to TCZ shortly before or during pregnancy were prospectively reported resulting in 109 live births (60.6%), 39 spontaneous abortions (21.7%), and 31 elective terminations (17.2%). Spontaneous abortions appeared to be higher, but the rate of malformations was 4.5% and there was no indication of a substantial increase [Citation160]. In a report of a patient who received TCZ throughout pregnancy, TCZ levels in umbilical cord blood were approximately one-seventh of the concentration in the maternal blood [Citation161]. In two cases that restarted TCZ after delivery, maternal breast milk levels of TCZ were reported to range from 1/500 to 1/1,000 of those in serum, and breastfed infants have no serious infections and no developmental delay [Citation162].
7.4. Children younger than 2 years
FDA and EMA approval of TCZ for the treatment of patients 2 years or older is based on the TENDER study in which 73 patients with sJIA aged 2–17 years received TCZ. TCZ was efficacious in treating JIA, and adverse events in this study were primarily infection [Citation163]. However, sJIA can be diagnosed in even younger patients and patients with very early onset before 18 months of age have been shown to exhibit a more severe course than patients with later onset [Citation164]. The first open-label phase 1 study to investigate TCZ treatment in patients with sJIA younger than 2 years reported that in 11 patients with sJIA who were on average 1.3 years old and received TCZ every 2 weeks, 4 patients withdrew by day 15 due to adverse events, 3 patients withdrew due to serious hypersensitivity, and 1 patient withdrew due to thrombocytopenia. Furthermore, 1 patient withdrew on day 112 with elevated transaminase [Citation165]. No additional safety signals were identified other than the higher incidence of serious hypersensitivity; however, no definitive conclusions can be made regarding the safety of TCZ because of the small sample size.
7.5. Inflammation markers under the IL-6–targeting therapy
The acute phase proteins under IL-6 inhibition are no longer reliable indices for objectively monitoring the inflammatory status in RA and infectious diseases. Thus, it is necessary to carefully observe patients’ signs and symptoms of disease, and utilize image examination including ultrasonography. As an alternative serum marker for activity of arthritis, matrix metalloproteinase-3 (MMP3, also known as stromelysin-1) increases in relation to arthritis and is often monitored in the clinic [Citation166,Citation167]. Neutrophil-to-lymphocyte ratio (NLR) and multi cytokine-dependent inflammatory markers such as leucine-rich α2 glycoprotein (LRG) have been reported to be significantly higher in a flare of RA under IL-6 inhibition therapy [Citation168,Citation169].
8. Conclusion
The approved indications for IL-6–targeting therapy have expanded from chronic inflammatory diseases such as Castleman disease, RA, juvenile idiopathic arthritis, large vessel vasculitis, and adult-onset Still’s disease to severe acute inflammatory conditions such as CRS and COVID-19. In addition, IL-6–targeting therapy has been used in NMOSD, suggesting the possibility of beneficial IL-6 inhibition for autoantibody-induced disease. Furthermore, the efficacy of IL-6 inhibition has been confirmed for interstitial lung disease accompanying SSc, and further investigation is required to determine the efficacy of IL-6 inhibition in various diseases associated with fibrosis. It is also expected to be confirmed that IL-6 inhibition prevents myocardial damage after myocardial infarction and also may have a beneficial effect on depression. Analysis of molecular changes in immune cells caused by IL-6 inhibition has also revealed that IL-6 inhibition normalizes neutrophil, B cell, Th17, and Treg parameters. IL-6–targeting therapy may be effective for diseases with these characteristics in immune cells. IL-6 inhibition normalizes serum levels of acute-phase proteins such as CRP that are routine laboratory test items to measure the severity of inflammation; therefore, under IL-6 inhibition, signs of infection should be carefully monitored and diagnosed before the infection becomes serious.
9. Expert opinion
For the diseases for which IL-6–targeting therapy has been approved, efficacy has been shown in randomized controlled trials and case series. Those diseases for which IL-6–targeting therapy is expected to be effective can be divided into the following four categories: ().
Figure 2. Diseases for which IL-6–targeting therapy has been approved, efficacy has been confirmed in randomized clinical trials, or efficacy has been reported in the case series can be divided into four categories: chronic inflammatory disease, acute inflammatory disease, autoantibody-induced disease, and interstitial lung disease.
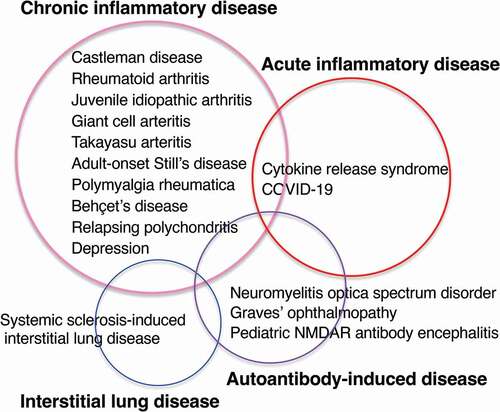
(1) Chronic inflammatory diseases that are characterized by changes that can be explained by the action of IL-6 in peripheral blood and biochemical tests, accompanied by high levels of acute-phase proteins, complement C3, and immunoglobulin, anemia, thrombocytosis, possibly accompanied by fever and pain, and swelling of affected inflammatory lesions as typically observed in Castleman disease and RA. IL-6 induces changes in immune cells resulting in an increase in neutrophils, an increase in Th17 cells, a decrease in Treg cells, and an increase in memory B cells. Inflammation is supported as a result of these activated immune cells. This category includes diseases such as juvenile idiopathic arthritis, giant cell arteritis, Takayasu arteritis, adult-onset Still’s disease, and evidence of the efficacy of IL-6 inhibition has also accumulated in polymyalgia rheumatica, Behçet’s disease, and relapsing polychondritis.
(2) Acute inflammatory diseases in which, in addition to the abnormal laboratory tests seen in chronic inflammatory diseases, IL-6 trans-signaling on vascular endothelial cells increases pro-inflammatory cytokine production, vascular permeability, and coagulation, resulting in a life-threatening condition developing in a short period of time. As the beneficial effects of IL-6–targeting therapy have been observed for severe acute inflammatory diseases such as CRS and COVID-19, it is expected that the therapy will also be effective for other acute inflammatory conditions, for example, after administration of anti-cancer drugs or antibody-based therapies such as RTX and nivolumab, and even in patients with graft-versus-host disease [Citation170]. It may also be effective for septic shock if effective antibiotic treatment is given [Citation103].
(3) The auto-antibody–induced disease. NMOSD, for which IL-6 targeted therapy has been approved, is a disease involving the anti–AQP-4 antibody; many effective cases of IL-6–targeting therapy have been reported for Graves’ ophthalmopathy that is caused by anti–thyrotropin receptor autoantibody, NMDAR Ab encephalitis, and MOG-IgG associated disorder, suggesting IL-6 inhibition may be widely effective for intractable autoantibody-induced chronic and acute diseases. However, the mechanism by which disease activity was improved is not clear. IL-6 inhibition may reduce the inflammation caused by autoantibodies or may reduce autoantibody production. Since autoantibodies are also reduced by a decrease in total IgG, it is important to consider whether the rate of decrease in autoantibodies is greater than the rate of decrease in total IgG. A clinical trial of TCZ in SLE reported that the anti-DNA Ab decreased by 47% more than the 7.8% decrease of IgG [Citation171], suggesting that IL-6 is involved in anti-DNA Ab production; however, autoantibodies often do not disappear completely, suggesting a mechanism of IL-6–dependent and independent autoantibody production. Whether IL-6–targeting therapy is effective for diseases caused by autoantibodies needs to be further established in clinical trials.
(4) Interstitial lung disease associated with SSc; a clinical trial demonstrating that IL-6–targeting therapy has been effective for this indication has expanded the possibility that this therapy will be useful in pathological conditions accompanied by progressive fibrosis. The background disease of SSc often accompanies autoantibodies, and SSc-related interstitial lung disease may also be induced by autoantibodies. Interstitial lung disease is a life-threatening condition associated with fibrosis. It usually progresses slowly and is often refractory. Further research will show whether IL-6–targeting therapy is effective against interstitial lung disease related to other rheumatic diseases.
As mentioned above, IL-6–targeting therapy is approved for many diseases and may be given as an option for inflammatory diseases that are refractory to conventional therapies. If IL-6–targeting therapy is not sufficiently effective, the following three points should be considered:
(1) Whether IL-6 activity is sufficiently suppressed. In order to judge the effectiveness of IL-6 inhibition, it is necessary to sufficiently suppress the biological activity of IL-6. As seen in Castleman disease, the Ab half-life is shortened when the reticuloendothelial system is activated. Alternatively, if the levels of a molecule (IL-6 in the case of anti–IL-6 R Ab) that competes with Ab binding are high, it is necessary to set the concentration of Ab higher. When IL-6 activity is sufficiently suppressed, serum levels of CRP and amyloid A protein usually drop below the measurable levels, and the neutrophils could often decrease. It is necessary to follow these laboratory data in order to confirm whether IL-6 activity is sufficiently suppressed. If IL-6 activity is not sufficiently suppressed, the TCZ dosing interval should be shortened, or the dose should be increased.
(2) Early start or appropriate treatment timing of IL-6–targeting therapy appears to increase its effectiveness. Comparing the DAS28 remission rates at 52 weeks in the LITHE, the SAMURAI, and the U-Act-Early trials for patients with RA whose average age were 53 years in all trials, the rate was 47.2% in patients with a 9.3 years history of RA in the LITHE study [Citation172], 59% in 2.2 years history of RA in the SAMURAI study [Citation173], and 71% in patients who had been diagnosed with RA within 1 year. Median symptom duration was only 25 days in the U-Act-Early study [Citation174], suggesting that inhibition of IL-6 at the early stages of RA is critical to increasing remission rates in patients with RA. Furthermore, in cytokine release syndrome, IL-6 signaling in endothelial cells forms an inflammatory circuit for the robust production of IL-6, IL-8, and MCP-1 and promotes PAI-1 production [Citation25]. Even if the IL-6 signal is suppressed, it may be too late to start therapy at a stage where organ damage has progressed to the point of being irreversible. It may be possible to observe the effect of IL-6–targeting therapy by starting at an early stage even for the diseases for which IL-6 inhibition has not been shown to be effective.
(3) Other factors than IL-6 are involved in the pathology. The pathological condition may be maintained by factors other than IL-6. When TCZ is inadequately effective against RA, the addition of tacrolimus to TCZ is reported to reduce disease activity [Citation166]. The double knock out of IL-6 and TNFR1 shows synergistic effects on arthritis in the collagen-induced arthritis model mice [Citation175]; thus, a treatment that suppresses multiple cytokines will also be an alternative option.
Cellular and molecular changes by IL-6 inhibition have been identified from the analysis of inflammatory lesions or peripheral blood before and after IL-6 inhibition, and characteristics have been extracted for which IL-6 inhibition is expected to be effective. By integrating the disease list for which IL-6–targeting therapy is effective and the molecular analysis of the pathological condition, it will be possible to predict the effectiveness of IL-6–targeting therapy in advance for each disease or individual patient. IL-6–targeting therapy started for patients with RA and now its application has expanded to many diseases worldwide and will benefit patients with a variety of intractable diseases.
Article highlights
IL-6–targeting therapy has been shown to be effective not only in various chronic inflammatory diseases but also in fatal acute inflammatory diseases. Furthermore, it has been found to be effective for pathological conditions caused by autoantibodies or pathological conditions accompanied by fibrosis.
There are many case reports in which IL-6 inhibition was effective, but further confirmation through clinical trials is required.
If the efficacy of IL-6–targeting therapy was inadequate, it is necessary to consider whether IL-6 activity was sufficiently suppressed, the timing of the treatment was appropriate, and whether other inflammatory pathways were involved.
The understanding of the characteristic cellular, molecular, and gene expression changes in the immune system that are significantly changed by IL-6 inhibition is improving.
In the future, it will be possible to predict the effectiveness of IL-6 inhibition in advance by analyzing the molecular profile of the disease in question.
Declaration of interest
M Narazaki has received a grant and payment from the Department of Advanced Clinical and Translational Immunology and Osaka University Graduate School of Medicine, which is collaborating with Chugai Pharmaceutical. T Kishimoto holds a patent for tocilizumab and has received royalties for Actemra. The authors have no other relevant affiliations or financial involvement with any organization or entity with a financial interest in or financial conflict with the subject matter or materials discussed in the manuscript apart from those disclosed.
Reviewer disclosures
Peer reviewers on this manuscript have no relevant financial or other relationships to disclose.
Additional information
Funding
References
- Feldmann M, Brennan FM, Maini RN. Role of cytokines in rheumatoid arthritis. Annu Rev Immunol. 1996;14(1):397–440.
- Hirano T, Yasukawa K, Harada H, et al. Complementary DNA for a novel human interleukin (BSF-2) that induces B lymphocytes to produce immunoglobulin. Nature. 1986 Nov 6-12;324(6092):73–76.
- Kishimoto T. Interleukin-6: from basic science to medicine–40 years in immunology. Annu Rev Immunol. 2005;23(1):1–21.
- Suematsu S, Matsuda T, Aozasa K, et al. IgG1 plasmacytosis in interleukin 6 transgenic mice. Proc Natl Acad Sci U S A. 1989 Oct;86(19):7547–7551.
- Kopf M, Baumann H, Freer G, et al. Impaired immune and acute-phase responses in interleukin-6-deficient mice. Nature. 1994 Mar 24;368(6469):339–342.
- Hunter CA, Jones SA. IL-6 as a keystone cytokine in health and disease. Nat Immunol. 2015 May;16(5):448–457.
- Narazaki M, Kishimoto T. The two-faced cytokine IL-6 in host defense and diseases. Int J Mol Sci. 2018 Nov 9;19(11):3528.
- Kang S, Tanaka T, Narazaki M, et al. Targeting interleukin-6 signaling in clinic. Immunity. 2019 Apr 16;50(4):1007–1023.
- Kishimoto T. IL-6: from arthritis to CAR-T-cell therapy and COVID-19. Int Immunol. 2021 Sep 25;33(10):515–519.
- Tanaka T, Narazaki M, Kishimoto T. IL-6 in inflammation, immunity, and disease. Cold Spring Harb Perspect Biol. 2014 Sep 4;6(10):a016295.
- Akira S, Takeda K. Toll-like receptor signalling. Nat Rev Immunol. 2004 Jul;4(7):499–511.
- Zimmermann M, Aguilera FB, Castellucci M, et al. Chromatin remodelling and autocrine TNFalpha are required for optimal interleukin-6 expression in activated human neutrophils. Nat Commun. 2015 Jan 23;6(1):6061.
- Garbers C, Heink S, Korn T, et al. Interleukin-6: designing specific therapeutics for a complex cytokine. Nat Rev Drug Discov. 2018 Jun;17(6):395–412.
- Ogura H, Murakami M, Okuyama Y, et al. Interleukin-17 promotes autoimmunity by triggering a positive-feedback loop via interleukin-6 induction. Immunity. 2008 Oct 17;29(4):628–636.
- Ma J, Zhang FL, Zhou G, et al. Different characteristics of hepcidin expression in IL-6+/+ and IL-6-/- neurons and astrocytes treated with lipopolysaccharides. Neurochem Res. 2018 Aug;43(8):1624–1630.
- Zhang F, Wei K, Slowikowski K, et al. Defining inflammatory cell states in rheumatoid arthritis joint synovial tissues by integrating single-cell transcriptomics and mass cytometry. Nat Immunol. 2019 Jul;20(7):928–942.
- Yoshizaki K, Matsuda T, Nishimoto N, et al. Pathogenic significance of interleukin-6 (IL-6/BSF-2) in Castleman’s disease. Blood. 1989 Sep;74(4):1360–1367.
- Emilie D, Liozon E, Crevon MC, et al. Production of interleukin 6 by granulomas of giant cell arteritis. Hum Immunol. 1994 Jan;39(1):17–24.
- Feist E, Mitrovic S, Fautrel B. Mechanisms, biomarkers and targets for adult-onset Still’s disease. Nat Rev Rheumatol. 2018 Oct;14(10):603–618.
- Liu Y, Xia C, Chen J, et al. Elevated circulating pro-inflammatory low-density granulocytes in adult-onset Still’s disease. Rheumatology (Oxford). 2021 Jan 5;60(1):297–303.
- Fujihara K, Bennett JL, de Seze J, et al. Interleukin-6 in neuromyelitis optica spectrum disorder pathophysiology. Neurol Neuroimmunol Neuroinflamm. 2020 Sep 3;7(5):e841.
- Kong BS, Kim Y, Kim GY, et al. Increased frequency of IL-6-producing non-classical monocytes in neuromyelitis optica spectrum disorder. J Neuroinflammation. 2017 Sep 25;14(1):191.
- Sachdeva M, Duchateau P, Depil S, et al. Granulocyte-macrophage colony-stimulating factor inactivation in CAR T-cells prevents monocyte-dependent release of key cytokine release syndrome mediators. J Biol Chem. 2019 Apr 5;294(14):5430–5437.
- Zhou Y, Fu B, Zheng X, et al. Pathogenic T-cells and inflammatory monocytes incite inflammatory storms in severe COVID-19 patients. Natl Sci Rev. 2020 Jun;7(6):998–1002.
- Kang S, Tanaka T, Inoue H, et al. IL-6 trans-signaling induces plasminogen activator inhibitor-1 from vascular endothelial cells in cytokine release syndrome. Proc Natl Acad Sci U S A. 2020 Sep 8;117(36):22351–22356.
- Paonessa G, Graziani R, De Serio A, et al. Two distinct and independent sites on IL-6 trigger gp 130 dimer formation and signalling. EMBO J. 1995 May 1;14(9):1942–1951.
- Taga T, Narazaki M, Yasukawa K, et al. Functional inhibition of hematopoietic and neurotrophic cytokines by blocking the interleukin 6 signal transducer gp130. Proc Natl Acad Sci U S A. 1992 Nov 15;89(22):10998–11001.
- Murakami M, Kamimura D, Hirano T. Pleiotropy and specificity: insights from the interleukin 6 family of cytokines. Immunity. 2019 Apr 16;50(4):812–831.
- Nanki T, Onoue I, Nagasaka K, et al. Suppression of elevations in serum C reactive protein levels by anti-IL-6 autoantibodies in two patients with severe bacterial infections. Ann Rheum Dis. 2013 Jun;72(6):1100–1102.
- Rose-John S. IL-6 trans-signaling via the soluble IL-6 receptor: importance for the pro-inflammatory activities of IL-6. Int J Biol Sci. 2012;8(9):1237–1247.
- Heink S, Yogev N, Garbers C, et al. Trans-presentation of IL-6 by dendritic cells is required for the priming of pathogenic TH17 cells. Nat Immunol. 2017 Jan;18(1):74–85.
- Narazaki M, Yasukawa K, Saito T, et al. Soluble forms of the interleukin-6 signal-transducing receptor component gp130 in human serum possessing a potential to inhibit signals through membrane-anchored gp130. Blood. 1993 Aug 15;82(4):1120–1126.
- Muller-Newen G, Kuster A, Hemmann U, et al. Soluble IL-6 receptor potentiates the antagonistic activity of soluble gp130 on IL-6 responses. J Immunol. 1998 Dec 1;161(11):6347–6355.
- Yamasaki K, Taga T, Hirata Y, et al. Cloning and expression of the human interleukin-6 (BSF-2/IFN beta 2) receptor. Science. 1988 Aug 12;241(4867):825–828.
- Hirata Y, Taga T, Hibi M, et al. Characterization of IL-6 receptor expression by monoclonal and polyclonal antibodies. J Immunol. 1989 Nov 1;143(9):2900–2906.
- Sato K, Tsuchiya M, Saldanha J, et al. Reshaping a human antibody to inhibit the interleukin 6-dependent tumor cell growth. Cancer Res. 1993 Feb 15;53(4):851–856.
- Klein B, Wijdenes J, Zhang XG, et al. Murine anti-interleukin-6 monoclonal antibody therapy for a patient with plasma cell leukemia. Blood. 1991 Sep 1;78(5):1198–1204.
- Yoshizaki K, Nishimoto N, Mihara M, et al. Therapy of rheumatoid arthritis by blocking IL-6 signal transduction with a humanized anti-IL-6 receptor antibody. Springer Semin Immunopathol. 1998;20(1–2):247–259.
- Nishimoto N, Kanakura Y, Aozasa K, et al. Humanized anti-interleukin-6 receptor antibody treatment of multicentric Castleman disease. Blood. 2005 Oct 15;106(8):2627–2632.
- Narazaki M, Tanaka T, Kishimoto T. The role and therapeutic targeting of IL-6 in rheumatoid arthritis. Expert Rev Clin Immunol. 2017 Jun;13(6):535–551.
- Smolen JS, Landewe R, Breedveld FC, et al. EULAR recommendations for the management of rheumatoid arthritis with synthetic and biological disease-modifying antirheumatic drugs. Ann Rheum Dis. 2010 Jun;69(6):964–975.
- Gabay C, Emery P, van Vollenhoven R, et al. Tocilizumab monotherapy versus Adalimumab monotherapy for treatment of rheumatoid arthritis (ADACTA): a randomised, double-blind, controlled phase 4 trial. Lancet. 2013 May 4;381(9877):1541–1550.
- Smolen JS, Landewe R, Breedveld FC, et al. EULAR recommendations for the management of rheumatoid arthritis with synthetic and biological disease-modifying antirheumatic drugs: 2013 update. Ann Rheum Dis. 2014 Mar;73(3):492–509.
- Nishimoto N, Yoshizaki K, Maeda K, et al. Toxicity, pharmacokinetics, and dose-finding study of repetitive treatment with the humanized anti-interleukin 6 receptor antibody MRA in rheumatoid arthritis. Phase I/II clinical study. J Rheumatol. 2003 Jul;30(7):1426–1435.
- Frey N, Grange S, Woodworth T. Population pharmacokinetic analysis of tocilizumab in patients with rheumatoid arthritis. J Clin Pharmacol. 2010 Jul;50(7):754–766.
- Nishimoto N, Terao K, Mima T, et al. Mechanisms and pathologic significances in increase in serum interleukin-6 (IL-6) and soluble IL-6 receptor after administration of an anti-IL-6 receptor antibody, tocilizumab, in patients with rheumatoid arthritis and Castleman disease. Blood. 2008 Nov 15;112(10):3959–3964.
- Genovese MC, Fleischmann R, Kivitz AJ, et al. Sarilumab plus methotrexate in patients with active rheumatoid arthritis and inadequate response to methotrexate: results of a phase III study. Arthritis Rheumatol. 2015 Jun;67(6):1424–1437.
- Burmester GR, Lin Y, Patel R, et al. Efficacy and safety of sarilumab monotherapy versus Adalimumab monotherapy for the treatment of patients with active rheumatoid arthritis (MONARCH): a randomised, double-blind, parallel-group phase III trial. Ann Rheum Dis. 2017 May;76(5):840–847.
- Rose-John S. The soluble interleukin 6 receptor: advanced therapeutic options in inflammation. Clin Pharmacol Ther. 2017 Oct;102(4):591–598.
- Miao S, Fan L, Zhao L, et al. Physicochemical and biological characterization of the proposed biosimilar tocilizumab. Biomed Res Int. 2017;2017:4926168.
- Zhang H, Wang H, Wei H, et al. A phase I clinical study comparing the tolerance, immunogenicity, and pharmacokinetics of proposed biosimilar BAT1806 and reference tocilizumab in healthy Chinese men. Front Pharmacol. 2020;11:609522.
- Zhang H, Li X, Liu J, et al. A randomized phase-I pharmacokinetic trial comparing the potential biosimilar tocilizumab (QX003S) with the reference product (Actemra((R))) in Chinese healthy subjects. Ann Med. 2021 Dec;53(1):375–383.
- Schwabe C, Illes A, Ullmann M, et al. Pharmacokinetics and pharmacodynamics of a proposed tocilizumab biosimilar MSB11456 versus both the US-licensed and EU-approved products: a randomized, double-blind trial. Expert Rev Clin Immunol. 2022 Mar 31;18(5):533–543.
- van Rhee F, Wong RS, Munshi N, et al. Siltuximab for multicentric Castleman’s disease: a randomised, double-blind, placebo-controlled trial. Lancet Oncol. 2014 Aug;15(9):966–974.
- Kretsos K, Golor G, Jullion A, et al. Safety and pharmacokinetics of olokizumab, an anti-IL-6 monoclonal antibody, administered to healthy male volunteers: a randomized phase I study. Clin Pharmacol Drug Dev. 2014 Sep;3(5):388–395.
- Nasonov E, Fatenejad S, Feist E, et al. Olokizumab, a monoclonal antibody against interleukin 6, in combination with methotrexate in patients with rheumatoid arthritis inadequately controlled by methotrexate: efficacy and safety results of a randomised controlled phase III study. Ann Rheum Dis. 2021 Aug 3;81(4):469–479.
- Aletaha D, Bingham CO 3rd, Tanaka Y, et al. Efficacy and safety of sirukumab in patients with active rheumatoid arthritis refractory to anti-TNF therapy (SIRROUND-T): a randomised, double-blind, placebo-controlled, parallel-group, multinational, phase 3 study. Lancet. 2017 Mar 25;389(10075):1206–1217.
- Takeuchi T, Thorne C, Karpouzas G, et al. Sirukumab for rheumatoid arthritis: the phase III SIRROUND-D study. Ann Rheum Dis. 2017 Dec;76(12):2001–2008.
- Avci AB, Feist E, Burmester GR. Targeting IL-6 or IL-6 receptor in rheumatoid arthritis: what’s the difference? BioDrugs. 2018 Dec;32(6):531–546.
- Kaur S, Bansal Y, Kumar R, et al. A panoramic review of IL-6: structure, pathophysiological roles and inhibitors. Bioorg Med Chem. 2020 Mar 1;28(5):115327.
- Russell B, Moss C, George G, et al. Associations between immune-suppressive and stimulating drugs and novel COVID-19-a systematic review of current evidence. Ecancermedicalscience. 2020;14:1022.
- Nishimoto N, Yoshizaki K, Miyasaka N, et al. Treatment of rheumatoid arthritis with humanized anti-interleukin-6 receptor antibody: a multicenter, double-blind, placebo-controlled trial. Arthritis Rheum. 2004 Jun;50(6):1761–1769.
- Hiroshima R, Kawakami K, Iwamoto T, et al. Analysis of C-reactive protein levels and febrile tendency after joint surgery in rheumatoid arthritis patients treated with a perioperative 4-week interruption of tocilizumab. Mod Rheumatol. 2011 Feb;21(1):109–111.
- Suwa T, Hogg JC, English D, et al. Interleukin-6 induces demargination of intravascular neutrophils and shortens their transit in marrow. Am J Physiol Heart Circ Physiol. 2000 Dec;279(6):H2954–60.
- Moots RJ, Sebba A, Rigby W, et al. Effect of tocilizumab on neutrophils in adult patients with rheumatoid arthritis: pooled analysis of data from phase 3 and 4 clinical trials. Rheumatology (Oxford). 2017 Apr 1;56(4):541–549.
- Nemeth E, Rivera S, Gabayan V, et al. IL-6 mediates hypoferremia of inflammation by inducing the synthesis of the iron regulatory hormone hepcidin. J Clin Invest. 2004 May;113(9):1271–1276.
- Song SN, Iwahashi M, Tomosugi N, et al. Comparative evaluation of the effects of treatment with tocilizumab and TNF-alpha inhibitors on serum hepcidin, anemia response and disease activity in rheumatoid arthritis patients. Arthritis Res Ther. 2013 Oct 2;15(5):R141.
- Gossec L, Steinberg G, Rouanet S, et al. Fatigue in rheumatoid arthritis: quantitative findings on the efficacy of tocilizumab and on factors associated with fatigue. The French multicentre prospective PEPS study. Clin Exp Rheumatol. 2015 Sep-Oct;33(5):664–670.
- Kaser A, Brandacher G, Steurer W, et al. Interleukin-6 stimulates thrombopoiesis through thrombopoietin: role in inflammatory thrombocytosis. Blood. 2001 Nov 1;98(9):2720–2725.
- Hashizume M, Hayakawa N, Mihara M. IL-6 trans-signalling directly induces RANKL on fibroblast-like synovial cells and is involved in RANKL induction by TNF-alpha and IL-17. Rheumatology (Oxford). 2008 Nov;47(11):1635–1640.
- De Benedetti F, Rucci N, Del Fattore A, et al. Impaired skeletal development in interleukin-6-transgenic mice: a model for the impact of chronic inflammation on the growing skeletal system. Arthritis Rheum. 2006 Nov;54(11):3551–3563.
- Finzel S, Kraus S, Figueiredo CP, et al. Comparison of the effects of tocilizumab monotherapy and Adalimumab in combination with methotrexate on bone erosion repair in rheumatoid arthritis. Ann Rheum Dis. 2019 Sep;78(9):1186–1191.
- Ducreux J, Durez P, Galant C, et al. Global molecular effects of tocilizumab therapy in rheumatoid arthritis synovium. Arthritis Rheumatol. 2014 Jan;66(1):15–23.
- Humby F, Durez P, Buch MH, et al. Rituximab versus tocilizumab in anti-TNF inadequate responder patients with rheumatoid arthritis (R4RA): 16-week outcomes of a stratified, biopsy-driven, multicentre, open-label, phase 4 randomised controlled trial. Lancet. 2021 Jan 23;397(10271):305–317.
- Chatzidionysiou K, Circiumaru A, Rethi B, et al. Tocilizumab decreases T cells but not macrophages in the synovium of patients with rheumatoid arthritis while it increases the levels of serum interleukin-6 and RANKL. RMD Open. 2021 Jun;7(2):e001662.
- Saito Y, Kagami S, Sanayama Y, et al. AT-rich-interactive domain-containing protein 5A functions as a negative regulator of retinoic acid receptor-related orphan nuclear receptor gammat-induced Th17 cell differentiation. Arthritis Rheumatol. 2014 May;66(5):1185–1194.
- Schraml BU, Hildner K, Ise W, et al. The AP-1 transcription factor Batf controls T(H)17 differentiation. Nature. 2009 Jul 16;460(7253):405–409.
- Masuda K, Ripley B, Nishimura R, et al. Arid5a controls IL-6 mRNA stability, which contributes to elevation of IL-6 level in vivo. Proc Natl Acad Sci U S A. 2013 Jun 4;110(23):9409–9414.
- Samson M, Audia S, Janikashvili N, et al. Brief report: inhibition of interleukin-6 function corrects Th17/Treg cell imbalance in patients with rheumatoid arthritis. Arthritis Rheum. 2012 Aug;64(8):2499–2503.
- Pesce B, Soto L, Sabugo F, et al. Effect of interleukin-6 receptor blockade on the balance between regulatory T cells and T helper type 17 cells in rheumatoid arthritis patients. Clin Exp Immunol. 2013 Mar;171(3):237–242.
- Kikuchi J, Hashizume M, Kaneko Y, et al. Peripheral blood CD4(+)CD25(+)CD127(low) regulatory T cells are significantly increased by tocilizumab treatment in patients with rheumatoid arthritis: increase in regulatory T cells correlates with clinical response. Arthritis Res Ther. 2015 Jan 21;17(1):10.
- Kimura A, Kishimoto T. IL-6: regulator of Treg/Th17 balance. Eur J Immunol. 2010 Jul;40(7):1830–1835.
- Nakamura S, Suzuki K, Iijima H, et al. Identification of baseline gene expression signatures predicting therapeutic responses to three biologic agents in rheumatoid arthritis: a retrospective observational study. Arthritis Res Ther. 2016 Jul 19;18(1):159.
- Roll P, Muhammad K, Schumann M, et al. In vivo effects of the anti-interleukin-6 receptor inhibitor tocilizumab on the B cell compartment. Arthritis Rheum. 2011 May;63(5):1255–1264.
- Tasaki S, Suzuki K, Kassai Y, et al. Multi-omics monitoring of drug response in rheumatoid arthritis in pursuit of molecular remission. Nat Commun. 2018 Jul 16;9(1):2755.
- Nakajima T, Watanabe R, Hashimoto M, et al. Neutrophil count reduction 1 month after initiating tocilizumab can predict clinical remission within 1 year in rheumatoid arthritis patients. Rheumatol Int. 2021 Jul 9. DOI:10.1007/s00296-021-04944-x.
- Nishimoto N, Amano K, Hirabayashi Y, et al. Drug free REmission/low disease activity after cessation of tocilizumab (Actemra) Monotherapy (DREAM) study. Mod Rheumatol. 2014 Jan;24(1):17–25.
- Spencer S, Kostel Bal S, Egner W, et al. Loss of the interleukin-6 receptor causes immunodeficiency, atopy, and abnormal inflammatory responses. J Exp Med. 2019 Sep 2;216(9):1986–1998.
- Koike T, Harigai M, Inokuma S, et al. Postmarketing surveillance of tocilizumab for rheumatoid arthritis in Japan: interim analysis of 3881 patients. Ann Rheum Dis. 2011 Dec;70(12):2148–2151.
- Navarini AA, French LE, Hofbauer GF. Interrupting IL-6-receptor signaling improves atopic dermatitis but associates with bacterial superinfection. J Allergy Clin Immunol. 2011 Nov;128(5):1128–1130.
- Massolino RI, Hissaria P, Lee A, et al. Tocilizumab-induced drug reaction with eosinophilia and systemic symptoms (DRESS) in a patient with rheumatoid arthritis. Rheumatol Adv Pract. 2018;2(2):rky029.
- Bloomfield M, Parackova Z, Cabelova T, et al. Anti-IL6 autoantibodies in an infant with CRP-less septic shock. Front Immunol. 2019;10:2629.
- Morel J, Constantin A, Baron G, et al. Risk factors of serious infections in patients with rheumatoid arthritis treated with tocilizumab in the French registry REGATE. Rheumatology (Oxford). 2017 Oct 1;56(10):1746–1754.
- Daien CI, Gailhac S, Audo R, et al. High levels of natural killer cells are associated with response to tocilizumab in patients with severe rheumatoid arthritis. Rheumatology (Oxford). 2015 Apr;54(4):601–608.
- Yokota S, Imagawa T, Mori M, et al. Efficacy and safety of tocilizumab in patients with systemic-onset juvenile idiopathic arthritis: a randomised, double-blind, placebo-controlled, withdrawal phase III trial. Lancet. 2008 Mar 22;371(9617):998–1006.
- Miyamae T, Yokoya S, Yamanaka H, et al. Effect of tocilizumab on growth impairment in systemic juvenile idiopathic arthritis with long-term corticosteroid therapy. Mod Rheumatol. 2014 Jul;24(4):567–571.
- De Benedetti F, Brunner H, Ruperto N, et al. Catch-up growth during tocilizumab therapy for systemic juvenile idiopathic arthritis: results from a phase III trial. Arthritis Rheumatol. 2015 Mar;67(3):840–848.
- Stone JH, Tuckwell K, Dimonaco S, et al. Trial of tocilizumab in giant-cell arteritis. N Engl J Med. 2017 Jul 27;377(4):317–328.
- Nakaoka Y, Isobe M, Takei S, et al. Efficacy and safety of tocilizumab in patients with refractory Takayasu arteritis: results from a randomised, double-blind, placebo-controlled, phase 3 trial in Japan (the TAKT study). Ann Rheum Dis. 2018 Mar;77(3):348–354.
- Mekinian A, Resche-Rigon M, Comarmond C, et al. Efficacy of tocilizumab in Takayasu arteritis: multicenter retrospective study of 46 patients. J Autoimmun. 2018 Jul;91:55–60.
- Ma Y, Wu M, Zhang X, et al. Efficacy and safety of tocilizumab with inhibition of interleukin-6 in adult-onset Still’s disease: a meta-analysis. Mod Rheumatol. 2018 Sep;28(5):849–857.
- Kaneko Y, Kameda H, Ikeda K, et al. Tocilizumab in patients with adult-onset still’s disease refractory to glucocorticoid treatment: a randomised, double-blind, placebo-controlled phase III trial. Ann Rheum Dis. 2018 Dec;77(12):1720–1729.
- Tanaka T, Narazaki M, Kishimoto T. Immunotherapeutic implications of IL-6 blockade for cytokine storm. Immunotherapy. 2016 Jul;8(8):959–970.
- Fitzgerald JC, Weiss SL, Maude SL, et al. Cytokine release syndrome after chimeric antigen receptor T cell therapy for acute lymphoblastic leukemia. Crit Care Med. 2017 Feb;45(2):e124–e131.
- Zhou F, Yu T, Du R, et al. Clinical course and risk factors for mortality of adult inpatients with COVID-19 in Wuhan, China: a retrospective cohort study. Lancet. 2020 Mar 28;395(10229):1054–1062.
- Mazzoni A, Salvati L, Maggi L, et al. Impaired immune cell cytotoxicity in severe COVID-19 is IL-6 dependent. J Clin Invest. 2020 Sep 1;130(9):4694–4703.
- Labo N, Ohnuki H, Tosato G. Vasculopathy and coagulopathy associated with SARS-CoV-2 infection. Cells. 2020 Jun 30;9(7):1583.
- Kenny G, Mallon PWG. Tocilizumab for the treatment of non-critical COVID-19 pneumonia: an overview of the rationale and clinical evidence to date. Expert Rev Clin Pharmacol. 2021 Oct;14(10):1279–1287.
- Aziz M, Haghbin H, Abu Sitta E, et al. Efficacy of tocilizumab in COVID-19: a systematic review and meta-analysis. J Med Virol. 2021 Mar;93(3):1620–1630.
- Hariyanto TI, Hardyson W, Kurniawan A. Efficacy and safety of tocilizumab for coronavirus disease 2019 (Covid-19) patients: a systematic review and meta-analysis. Drug Res (Stuttg). 2021 May;71(5):265–274.
- Patel S, Saxena B, Mehta P. Recent updates in the clinical trials of therapeutic monoclonal antibodies targeting cytokine storm for the management of COVID-19. Heliyon. 2021 Feb;7(2):e06158.
- Wingerchuk DM, Banwell B, Bennett JL, et al. International consensus diagnostic criteria for neuromyelitis optica spectrum disorders. Neurology. 2015 Jul 14;85(2):177–189.
- Chihara N, Aranami T, Sato W, et al. Interleukin 6 signaling promotes anti-aquaporin 4 autoantibody production from plasmablasts in neuromyelitis optica. Proc Natl Acad Sci U S A. 2011 Mar 1;108(9):3701–3706.
- Xie Q, Zheng T, Sun M, et al. A meta-analysis to determine the efficacy and safety of tocilizumab in neuromyelitis optica spectrum disorders. Mult Scler Relat Disord. 2020 Oct;45:102421.
- Igawa T, Ishii S, Tachibana T, et al. Antibody recycling by engineered pH-dependent antigen binding improves the duration of antigen neutralization. Nat Biotechnol. 2010 Nov;28(11):1203–1207.
- Yamamura T, Kleiter I, Fujihara K, et al. Trial of satralizumab in neuromyelitis optica spectrum disorder. N Engl J Med. 2019 Nov 28;381(22):2114–2124.
- Bahn RS. Graves’ ophthalmopathy. N Engl J Med. 2010 Feb 25;362(8):726–738.
- Khong JJ, McNab AA, Ebeling PR, et al. Pathogenesis of thyroid eye disease: review and update on molecular mechanisms. Br J Ophthalmol. 2016 Jan;100(1):142–150.
- Perez-Moreiras JV, Gomez-Reino JJ, Maneiro JR, et al. Efficacy of tocilizumab in patients with moderate-to-severe corticosteroid-resistant graves orbitopathy: a randomized clinical trial. Am J Ophthalmol. 2018 Nov;195:181–190.
- Bartalena L, Kahaly GJ, Baldeschi L, et al. The 2021 European group on Graves’ orbitopathy (EUGOGO) clinical practice guidelines for the medical management of Graves’ orbitopathy. Eur J Endocrinol. 2021 Aug 27;185(4):G43–G67.
- Dalmau J, Lancaster E, Martinez-Hernandez E, et al. Clinical experience and laboratory investigations in patients with anti-NMDAR encephalitis. Lancet Neurol. 2011 Jan;10(1):63–74.
- Lee WJ, Lee ST, Moon J, et al. Tocilizumab in autoimmune encephalitis refractory to rituximab: an institutional Cohort study. Neurotherapeutics. 2016 Oct;13(4):824–832.
- Nosadini M, Thomas T, and Eyre M, et al. International consensus recommendations for the treatment of pediatric NMDAR antibody encephalitis. Neurol Neuroimmunol Neuroinflamm. 2021 Jul;8(5). DOI:10.1212/NXI.0000000000001052.
- Steen VD, Medsger TA. Changes in causes of death in systemic sclerosis, 1972-2002. Ann Rheum Dis. 2007 Jul;66(7):940–944.
- Gao X, Jia G, Guttman A, et al. Osteopontin links myeloid activation and disease progression in systemic sclerosis. ell Rep Med. 2020 Nov 17;1(8):100140.
- Khanna D, Denton CP, Jahreis A, et al. Safety and efficacy of subcutaneous tocilizumab in adults with systemic sclerosis (faSScinate): a phase 2, randomised, controlled trial. Lancet. 2016 Jun 25;387(10038):2630–2640.
- Khanna D, Lin CJF, Furst DE, et al. Tocilizumab in systemic sclerosis: a randomised, double-blind, placebo-controlled, phase 3 trial. Lancet Respir Med. 2020 Oct;8(10):963–974.
- Avina-Zubieta JA, Thomas J, Sadatsafavi M, et al. Risk of incident cardiovascular events in patients with rheumatoid arthritis: a meta-analysis of observational studies. Ann Rheum Dis. 2012 Sep;71(9):1524–1529.
- Peters MJ, Symmons DP, McCarey D, et al. EULAR evidence-based recommendations for cardiovascular risk management in patients with rheumatoid arthritis and other forms of inflammatory arthritis. Ann Rheum Dis. 2010 Feb;69(2):325–331.
- Pierini FS, Botta E, Soriano ER, et al. Effect of tocilizumab on LDL and HDL characteristics in patients with rheumatoid arthritis. An Observational Study. Rheumatol Ther. 2021 Jun; 8(2):803–815
- Greco D, Gualtierotti R, Agosti P, et al. Anti-atherogenic modification of serum lipoprotein function in patients with rheumatoid arthritis after tocilizumab treatment, a pilot study. J Clin Med. 2020 Jul 8;9(7):2157.
- Singh S, Fumery M, Singh AG, et al. Comparative risk of cardiovascular events with biologic and synthetic disease-modifying antirheumatic drugs in patients with rheumatoid arthritis: a systematic review and meta-analysis. Arthritis Care Res (Hoboken). 2020 Apr;72(4):561–576.
- Zamani P, Schwartz GG, Olsson AG, et al. Inflammatory biomarkers, death, and recurrent nonfatal coronary events after an acute coronary syndrome in the MIRACL study. J Am Heart Assoc. 2013 Jan 28;2(1):e003103.
- Kleveland O, Kunszt G, Bratlie M, et al. Effect of a single dose of the interleukin-6 receptor antagonist tocilizumab on inflammation and troponin T release in patients with non-ST-elevation myocardial infarction: a double-blind, randomized, placebo-controlled phase 2 trial. Eur Heart J. 2016 Aug 7;37(30):2406–2413.
- Carroll MB, Haller C, Smith C. Short-term application of tocilizumab during myocardial infarction (STAT-MI). Rheumatol Int. 2018 Jan;38(1):59–66.
- Meyer MAS, Wiberg S, Grand J, et al. Treatment effects of interleukin-6 receptor antibodies for modulating the systemic inflammatory response after out-of-hospital cardiac arrest (The IMICA Trial): a double-blinded, placebo-controlled, single-center, randomized, clinical trial. Circulation. 2021 May 11;143(19):1841–1851.
- Bonelli M, Radner H, Kerschbaumer A, et al. Tocilizumab in patients with new onset polymyalgia rheumatica (PMR-SPARE): a phase 2/3 randomised controlled trial. Ann Rheum Dis. 2022 Feb 24;81(6):838–844.
- Chandran S, Leung J, Hu C, et al. Interleukin-6 blockade with tocilizumab increases Tregs and reduces T effector cytokines in renal graft inflammation: a randomized controlled trial. Am J Transplant. 2021 Jul;21(7):2543–2554.
- Marrie RA, Hitchon CA, Walld R, et al. Increased burden of psychiatric disorders in rheumatoid arthritis. Arthritis Care Res (Hoboken). 2018 Jul;70(7):970–978.
- Dowlati Y, Herrmann N, Swardfager W, et al. A meta-analysis of cytokines in major depression. Biol Psychiatry. 2010 Mar 1;67(5):446–457.
- Tiosano S, Yavne Y, Watad A, et al. The impact of tocilizumab on anxiety and depression in patients with rheumatoid arthritis. Eur J Clin Invest. 2020 Sep;50(9):e13268.
- Sun Y, Wang D, Salvadore G, et al. The effects of interleukin-6 neutralizing antibodies on symptoms of depressed mood and anhedonia in patients with rheumatoid arthritis and multicentric Castleman’s disease. Brain Behav Immun. 2017 Nov;66:156–164.
- Okuda Y, Ohnishi M, Matoba K, et al. Comparison of the clinical utility of tocilizumab and anti-TNF therapy in AA amyloidosis complicating rheumatic diseases. Mod Rheumatol. 2014 Jan;24(1):137–143.
- Akiyama M, Kaneko Y, Takeuchi T. Effectiveness of tocilizumab in Behcet’s disease: a systematic literature review. Semin Arthritis Rheum. 2020 Aug;50(4):797–804.
- Kirino Y, Takase-Minegishi K, Tsuchida N, et al. Tocilizumab in VEXAS relapsing polychondritis: a single-center pilot study in Japan. Ann Rheum Dis. 2021 Nov;80(11):1501–1502.
- Stroud CR, Hegde A, Cherry C, et al. Tocilizumab for the management of immune mediated adverse events secondary to PD-1 blockade. J Oncol Pharm Pract. 2019 Apr;25(3):551–557.
- Jin L, Sun Z, Liu H, et al. Inflammatory monocytes promote pre-engraftment syndrome and tocilizumab can therapeutically limit pathology in patients. Nat Commun. 2021 Jul 6;12(1):4137.
- Elsbernd PM, Hoffman WR, Carter JL, et al. Interleukin-6 inhibition with tocilizumab for relapsing MOG-IgG associated disorder (MOGAD): a case-series and review. Mult Scler Relat Disord. 2021 Feb;48:102696.
- Sieper J, Porter-Brown B, Thompson L, et al. Assessment of short-term symptomatic efficacy of tocilizumab in ankylosing spondylitis: results of randomised, placebo-controlled trials. Ann Rheum Dis. 2014 Jan;73(1):95–100.
- Sieper J, Braun J, Kay J, et al. Sarilumab for the treatment of ankylosing spondylitis: results of a Phase II, randomised, double-blind, placebo-controlled study (ALIGN). Ann Rheum Dis. 2015 Jun;74(6):1051–1057.
- Mease PJ, Gottlieb AB, Berman A, et al. The efficacy and safety of clazakizumab, an anti-interleukin-6 monoclonal antibody, in a phase IIb study of adults with active psoriatic arthritis. Arthritis Rheumatol. 2016 Sep;68(9):2163–2173.
- Kennedy GA, Tey SK, Buizen L, et al. A phase 3 double-blind study of the addition of tocilizumab vs placebo to cyclosporin/methotrexate GVHD prophylaxis. Blood. 2021 Apr 8;137(14):1970–1979.
- Rutherford AI, Subesinghe S, Hyrich KL, et al. Serious infection across biologic-treated patients with rheumatoid arthritis: results from the British society for rheumatology biologics register for rheumatoid arthritis. Ann Rheum Dis. 2018 Jun;77(6):905–910.
- Yun H, Xie F, Delzell E, et al. Comparative risk of hospitalized infection associated with biologic agents in rheumatoid arthritis patients enrolled in medicare. Arthritis Rheumatol. 2016 Jan;68(1):56–66.
- Grivennikov S, Karin E, Terzic J, et al. IL-6 and Stat3 are required for survival of intestinal epithelial cells and development of colitis-associated cancer. Cancer Cell. 2009 Feb 3;15(2):103–113.
- Taniguchi K, Wu LW, Grivennikov SI, et al. A gp130-Src-YAP module links inflammation to epithelial regeneration. Nature. 2015 Mar 5;519(7541):57–62.
- Strangfeld A, Richter A, Siegmund B, et al. Risk for lower intestinal perforations in patients with rheumatoid arthritis treated with tocilizumab in comparison to treatment with other biologic or conventional synthetic DMARDs. Ann Rheum Dis. 2017 Mar;76(3):504–510.
- Weber-Schoendorfer C, Schaefer C. Pregnancy outcome after tocilizumab therapy in early pregnancy-a case series from the German embryotox pharmacovigilance center. Reprod Toxicol. 2016 Apr;60:29–32.
- Nakajima K, Watanabe O, Mochizuki M, et al. Pregnancy outcomes after exposure to tocilizumab: a retrospective analysis of 61 patients in Japan. Mod Rheumatol. 2016 Sep;26(5):667–671.
- Hoeltzenbein M, Beck E, Rajwanshi R, et al. Tocilizumab use in pregnancy: analysis of a global safety database including data from clinical trials and post-marketing data. Semin Arthritis Rheum. 2016 Oct;46(2):238–245.
- Saito J, Yakuwa N, Kaneko K, et al. Tocilizumab during pregnancy and lactation: drug levels in maternal serum, cord blood, breast milk and infant serum. Rheumatology (Oxford). 2019 Aug 1;58(8):1505–1507.
- Saito J, Yakuwa N, Takai C, et al. Tocilizumab concentrations in maternal serum and breast milk during breastfeeding and a safety assessment in infants: a case study. Rheumatology (Oxford). 2018 Aug 1;57(8):1499–1501.
- De Benedetti F, Brunner HI, Ruperto N, et al. Randomized trial of tocilizumab in systemic juvenile idiopathic arthritis. N Engl J Med. 2012 Dec 20;367(25):2385–2395.
- Russo RA, Katsicas MM. Patients with very early-onset systemic juvenile idiopathic arthritis exhibit more inflammatory features and a worse outcome. J Rheumatol. 2013 Mar;40(3):329–334.
- Mallalieu NL, Wimalasundera S, Hsu JC, et al. Intravenous dosing of tocilizumab in patients younger than two years of age with systemic juvenile idiopathic arthritis: results from an open-label phase 1 clinical trial. Pediatr Rheumatol Online J. 2019 Aug 22;17(1):57.
- Kaneshiro S, Ebina K, Hirao M, et al. The efficacy and safety of additional administration of tacrolimus in patients with rheumatoid arthritis who showed an inadequate response to tocilizumab. Mod Rheumatol. 2017 Jan;27(1):42–49.
- Lerner A, Neidhofer S, Reuter S, et al. MMP3 is a reliable marker for disease activity, radiological monitoring, disease outcome predictability, and therapeutic response in rheumatoid arthritis. Best Pract Res Clin Rheumatol. 2018 Aug;32(4):550–562.
- Ghang B, Kwon O, Hong S, et al. Neutrophil-to-lymphocyte ratio is a reliable marker of treatment response in rheumatoid arthritis patients during tocilizumab therapy. Mod Rheumatol. 2017 May;27(3):405–410.
- Naka T, Fujimoto M. LRG is a novel inflammatory marker clinically useful for the evaluation of disease activity in rheumatoid arthritis and inflammatory bowel disease. Immunol Med. 2018 Jun;41(2):62–67.
- Shimabukuro-Vornhagen A, Godel P, Subklewe M, et al. Cytokine release syndrome. J Immunother Cancer. 2018 Jun 15;6(1):56.
- Illei GG, Shirota Y, Yarboro CH, et al. Tocilizumab in systemic lupus erythematosus: data on safety, preliminary efficacy, and impact on circulating plasma cells from an open-label phase I dosage-escalation study. Arthritis Rheum. 2010 Feb;62(2):542–552.
- Kremer JM, Blanco R, Brzosko M, et al. Tocilizumab inhibits structural joint damage in rheumatoid arthritis patients with inadequate responses to methotrexate: results from the double-blind treatment phase of a randomized placebo-controlled trial of tocilizumab safety and prevention of structural joint damage at one year. Arthritis Rheum. 2011 Mar;63(3):609–621.
- Nishimoto N, Hashimoto J, Miyasaka N, et al. Study of active controlled monotherapy used for rheumatoid arthritis, an IL-6 inhibitor (SAMURAI): evidence of clinical and radiographic benefit from an x ray reader-blinded randomised controlled trial of tocilizumab. Ann Rheum Dis. 2007 Sep;66(9):1162–1167.
- Bijlsma JWJ, Welsing PMJ, Woodworth TG, et al. Early rheumatoid arthritis treated with tocilizumab, methotrexate, or their combination (U-Act-Early): a multicentre, randomised, double-blind, double-dummy, strategy trial. Lancet. 2016 Jul 23;388(10042):343–355.
- Yamaguchi N, Ohshima S, Umeshita-Sasai M, et al. Synergistic effect on the attenuation of collagen induced arthritis in tumor necrosis factor receptor I (TNFRI) and interleukin 6 double knockout mice. J Rheumatol. 2003 Jan;30(1):22–27.