ABSTRACT
Introduction
Sonidegib and vismodegib are currently the only US Food and Drug Administration and European Medicines Agency-approved small-molecule Hedgehog pathway inhibitors (HHIs)for treating adults with advanced or refractory basal cell carcinoma (BCC) that is not amenable to conventional surgery or radiotherapy. At this time, there are no head-to-head clinical trials comparing these two HHIs for efficacy and safety to assist clinicians with determining which HHI may be best suited for their patients.
Areas covered
This review briefly describes the pathogenesis of BCC, provides a detailed overview of the key pharmacokinetic profile differences between sonidegib and vismodegib, explains their pharmacodynamics, and highlights the therapeutic considerations when either HHI is used to treat special patient populations.
Expert opinion
Although both HHIs act at the same molecular target in the Hedgehog pathway, there are significant differences in their pharmacokinetic profiles that may play a potential role in their efficacy and safety. Evidence-based recommendations serve to inform clinicians until direct comparative clinical trials of sonidegib versus vismodegib are conducted to determine the clinical relevance of the reported differences in their pharmacokinetic properties.
1. Introduction
Basal cell carcinoma (BCC) is the most common keratinocyte tumor and human malignancy worldwide, whose incidence is steadily increasing by approximately 1% annually for an aging Caucasian population [Citation1,Citation2]. Conventional treatment options for low-risk BCC include surgical excision, curettage, topical agents, or radiation therapy [Citation3,Citation4]. According to the 2023 National Comprehensive Cancer Network Clinical Practice GuidelinesⓇ in Oncology: Basal Cell Skin Cancer, the primary goal of treatment is complete removal of the tumor with maximal preservation of function and physical appearance [Citation5]. However, for the subset of advanced high-risk BCCs, which includes locally advanced BCC (laBCC) and metastatic BCC (mBCC), curative surgery, topical therapy, or localized radiation treatment may no longer be feasible or amenable [Citation5–8].
The Hedgehog (HH) pathway is a key regulator of cell growth and differentiation during development and is otherwise mostly silenced in adulthood with only limited activity; however, the aberrant activation of this pathway is central to the pathogenesis and progression of BCC () [Citation9,Citation10]. A major driver of BCC pathogenesis is an inactivation mutation (loss-of-function mutations) of a negative pathway regulator protein, patched homolog 1 (PTCH1) [Citation11–15]. Although less common, activating mutations in a positive regulator of the HH pathway, Smoothened homolog (SMO), can also cause abnormal HH pathway activation [Citation16]. Dysregulation of the HH pathway leads to the loss of glioma-associated oncogene homolog 1 and 2 (GLI1 and GLI2) cytosolic sequestering and overactivation of these transcription factors [Citation17–20]. Unregulated signaling via HH pathway activation facilitates uncontrolled BCC proliferation and neoplastic cell survival [Citation18]. These protein components, which are involved in the regulation of the HH pathway, have therefore become a therapeutic molecular target leading to the development of an entire class of inhibitors (HHIs) for the treatment of BCC () [Citation21].
Figure 1. Schematic of the HH signaling pathway. (a) under physiologic conditions, binding of the Hedgehog (HH) ligand to patched homolog 1 (PTCH1), a negative regulator of smoothened (SMO), triggers SMO activation. SMO initiates an intracellular signaling cascade that causes glioma-associated oncogene homolog 1 and 2 (GLI1 and GLI2) activation and nuclear translocation. GLI1 and GLI2 are transcription factors that facilitate the induction of HH target gene expression. (b) PTCH1 loss-of-function mutations and/or SMO activating mutations cause aberrant HH pathway signaling that drives unregulated transcription and uncontrolled basal cell carcinoma (BCC) proliferation. Sonidegib and vismodegib are SMO antagonists, while other inhibitors are (i) HH/PTCH1 inhibitors (Robotnikinin, 7_3d3, 5E1 monoclonal antibody therapy, and RU-SKI 43) or (ii) GLI inhibitors (GANT-58, GANT-61, and arsenic trioxide). Created with BioRender.com.
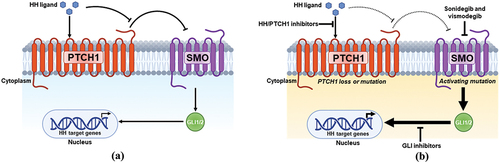
Although the development of therapeutic agents inhibiting HH pathway signaling primarily focuses on targeting SMO, the HHI class consists of a multitude of compounds that interfere at distinct points in the pathway. Robotnikinin, 7_3d3, 5E1 monoclonal antibody therapy, and RU-SKI 43 comprise a class of inhibitors that directly bind to or interfere with modification of HHs ligand to prevent canonical HH/PTCH1 binding and pathway activation [Citation22–26]. GLI antagonists (GANT58 and GANT61) and arsenic trioxide (ATO) are other classes of molecules, which are HHIs that reduce GLI-mediated gene activation and transcription [Citation27–29]. Cyclopamine was the first small molecule of this class that inhibited the HH pathway by binding SMO, which inhibited tumor growth [Citation30–33]. Despite the therapeutic novelty of cyclopamine, limitations such as scarcity, poor aqueous stability, acid lability, and adverse systemic side effects (especially teratogenic effects) consequently led to the development of novel synthetic analogues and mimetics [Citation34–36]. Given the success in preclinical and clinical studies among these three subclasses of HHIs, currently only two systemic SMO antagonists are approved by the US Food and Drug Administration (FDA) and European Medicines Agency (EMA) as treatments for BCC: sonidegib (ODOMZO®; Sun Pharmaceutical Industries, Inc.; Cranbury, NJ) and vismodegib (Erivedge®; Genentech U.S.A., Inc.; San Francisco, CA) [Citation37–42]. Sonidegib is approved for adult patients with laBCC, and vismodegib is approved for adults with mBCC or laBCC. Both drugs are treatments for patients in which BCC has recurred following surgery or radiation therapy or for patients who are not candidates for surgery or radiation therapy [Citation41,Citation42]. This review manuscript provides an overview of the pharmacokinetic and pharmacodynamic profile differences between sonidegib and vismodegib, highlighting the therapeutic considerations for each HHI when used to treat special patient populations.
2. Pharmacokinetics of sonidegib and vismodegib
Sonidegib (molecular weight, 485.5 g/mol) and vismodegib (molecular weight, 421.3 g/mol) are both oral, small-molecule inhibitors of SMO that received FDA/EMA approval based on the pivotal BOLT (NCT01327053) and ERIVANCE (NCT00833417) trials, respectively [Citation37–40,Citation43,Citation44]. Although monotherapy clinical trials for each inhibitor have been conducted, no direct head-to-head comparison clinical studies have been performed to date. Sonidegib and vismodegib possess similar physicochemical and pharmaceutical properties () including similar efficacy and safety profiles, but substantially different pharmacokinetic profiles (). These pharmacokinetic differences could potentially account for the observed differences in frequency and time of onset of treatment-emergent adverse events (TEAEs), which can be a major cause of treatment discontinuation and are a concern for both prescribing clinicians and patients [Citation45,Citation46]. Pharmacokinetic profiles and parameters for each of the HHIs have been determined in healthy participants [Citation47–50] and patients with solid tumors [Citation51–56], and data collected from patients enrolled in the clinical trials conducted for each HHI [Citation43,Citation44,Citation57,Citation58] for population pharmacokinetic (pop PK) modeling.
Table 1. Pharmaceutical properties of sonidegib and vismodegib.
Table 2. Summary of pharmacokinetic parameters for sonidegib and vismodegib.
The standard oral daily dosing for sonidegib is 200 mg/day and that for vismodegib is 150 mg/day [Citation41,Citation42]. Sonidegib is recommended to be taken on an empty stomach either 1 hour before a meal or 2 hours after, whereas vismodegib can be taken with or without food. Patients are instructed to continue each treatment course until disease progression or unacceptable toxicity. For intolerable adverse reactions with sonidegib, temporary dose interruptions may be required for several weeks (with or without subsequent dose reduction) or until resolution (≤Grade 1), or dose reductions to 200 mg every other day may be required, whereas vismodegib should be discontinued for up to 8 weeks, until improvement or resolution is observed [Citation37,Citation41].
2.1. Absorption
Sonidegib and vismodegib are both Biopharmaceutics Classification System Class II compounds with low solubility and high permeability [Citation37,Citation38]. The maximum plasma concentration (Cmax) following single 200-mg doses of sonidegib was 160 ng/mL in patients with advanced solid tumors versus 104 ng/mL in healthy individuals [Citation54,Citation59]. Cmax for repeated (once daily for 2 weeks) 200-mg doses of sonidegib in cancer patients is 269 ng/mL [Citation54]. Plasma concentrations following sonidegib single-dose administration increased dose proportionally from 100 up to 400 mg, where less than dose-proportional increases were observed for > 400-mg doses [Citation50]. Following a single 150-mg oral dose of vismodegib in healthy volunteers, Cmax was 2506.7–3231.4 ng/mL, whereas in patients with locally advanced or metastatic solid tumors, it was reportedly 1508.3 ng/mL [Citation48,Citation49,Citation52]. Cmax for repeated dosing with vismodegib (1 week of 150 mg once daily) in healthy patients was 6909.32 ng/mL [Citation48]. Similar to sonidegib, dose-proportional exposure increases were observed at lower doses (150- and 270-mg single doses) of vismodegib, but the lack of dose-proportional increase at 540 mg demonstrates absorption was saturable and solubility-limited [Citation38,Citation52,Citation53].
The median time to reach maximum observed concentration (Tmax) following oral dosing of sonidegib (single doses ranging from 100 to 3000 mg) was 2–4 hours [Citation37,Citation50,Citation54]. After repeated dosing (100–3000 mg once daily for 2 weeks), Tmax was 2–13 hours [Citation54]. In contrast, vismodegib (150-mg oral dose) in healthy volunteers had a wide Tmax range (1–48 hours) for single dosing, with a more narrow Tmax range observed (1–10 hours) for repeated dosing (150 mg once daily for 1 week) [Citation48]. The bioavailability of sonidegib (800-mg single oral dose) under prandial fasting conditions in healthy volunteers was approximately 6%–7% [Citation50]. Under fasted prandial conditions in healthy volunteers, the absolute bioavailability of vismodegib (150-mg single oral dose) was 31.8% [Citation48].
In terms of area under the plasma concentration curve from the time of dosing to the last measurable concentration (AUClast), it was relatively similar for 200-mg single oral doses of sonidegib in healthy volunteers versus patients with cancer (3327 vs 3670 ng x h/mL, respectively) [Citation54,Citation59]. The AUClast for single 150-mg oral doses of vismodegib in patients with solid tumors was reportedly 135,658.6 ng x h/mL [Citation52]. Food had a major impact on sonidegib absorption, such that when an 800-mg single oral dose was administered to healthy individuals with a high-fat meal, the AUCinf and Cmax increased by 7.4- and 7.8-fold, respectively, which was substantially increased compared with individuals who were studied in the fasting prandial state [Citation37]. Vismodegib concentrations (150-mg single dose) were mildly impacted by food, in which there was an 84% increase in absorption under fed (high-fat meal) conditions compared with the fasting prandial state, with a notably high degree of interindividual variability (coefficient of variation for Cmax [55% and 45%] and AUC0–168 [56% and 54%] for the fasted versus high-fat fed groups, respectively) [Citation38,Citation55].
2.2. Distribution
Although both HHIs demonstrated high binding affinity (≥97% for sonidegib, >99% for vismodegib) to plasma proteins alpha-1-acid glycoprotein (AAG) and human serum albumin, there were appreciable differences in their binding profiles [Citation37,Citation38,Citation60]. Sonidegib binding of plasma proteins is not concentration dependent within the dose range of 200–800 mg [Citation56]. However, plasma protein binding of ≥150 mg vismodegib was rapid, concentration dependent (nonlinear), saturable, and highly reversible [Citation52,Citation53,Citation61]. The therapeutic implication of this difference is that while the pharmacokinetic properties of sonidegib are dose proportional, higher doses of vismodegib do not increase the free drug concentrations [Citation53,Citation62]. To further understand the distribution parameters of these two HHIs, 14C-sonidegib and 14C-vismodegib were studied in healthy participants [Citation48,Citation50]. The apparent volume of distribution (Vd/F) for sonidegib (Vd/F, >9000 L) was considerably greater than vismodegib (Vd/F, 16–27 L) [Citation37,Citation38,Citation48,Citation50,Citation63]. This Vd/F difference suggests that vismodegib might be largely confined to the plasma with limited tissue penetration, whereas sonidegib, which is a considerably more lipophilic molecule, was extensively distributed into the tissues. Ultimately, over time, the concentration of sonidegib is 6-fold greater in skin than in plasma and has demonstrated effective penetration of the blood–brain barrier in preclinical studies [Citation37,Citation60].
2.3. Metabolism
Extensive in vitro data using human hepatic microsomes and a physiologically based pharmacokinetic (PBPK) model were used to elucidate the contributions of different cytochrome P450 (CYP) enzymes involved in the metabolism of the two HHIs. Sonidegib was primarily metabolized by CYP3A4 in the liver, via oxidation and amide hydrolysis [Citation37,Citation42]. The concomitant administration of strong CYP3A inhibitors or strong/moderate CYP3A inducers with sonidegib should therefore be avoided [Citation42]. However, coadministration of a moderate CYP3A inhibitor is permitted for <14 days, but close monitoring for adverse reactions, particularly musculoskeletal-related adverse reactions, is strongly recommended [Citation42,Citation59]. Although preclinical in vitro findings suggested sonidegib to be a potentially strong inhibitor of CYP2C9 and CYP2B6, drug-drug interaction (DDI) potential was clinically assessed by coadministration of the CYP probe substrates warfarin (CYP2C9 probe substrate) and bupropion (CYP2B6 probe substrate) with sonidegib [Citation64]. This study revealed that sonidegib 800 mg dosed orally once daily (higher than the approved/standard dose) did not impact the pharmacokinetics or pharmacodynamics of warfarin or the pharmacokinetics of bupropion and had an acceptable safety profile consistent with sonidegib monotherapy [Citation64].
Although >98% of vismodegib in circulation was the unmetabolized drug, vismodegib metabolism primarily occurs by CYP2C9 and CYP3A4/5 via oxidation as well as other enzymes via glucuronidation and pyridine ring cleavage [Citation38,Citation41]. The results of a clinical study that assessed concomitant use of fluconazole (CYP2C9 and CYP3A4 probe substrate) and itraconazole (CYP3A4 probe substrate) with vismodegib revealed no clinically significant difference in vismodegib pharmacokinetics [Citation65]. Preclinical DDI assessments using selective probe substrates and human liver microsomes demonstrated vismodegib is not a potent inhibitor of CYP1A2, CYP2B6, CYP3A4/5, or CYP2D6 [Citation66]. Additional in vitro studies using paclitaxel (CYP2C8 probe substrate) and warfarin suggested vismodegib to be a moderate inhibitor of CYP2C8 and CYP2C9 [Citation66]. However, follow-up PBPK simulations modeling coadministration of rosiglitazone (CYP2C8 probe substrate) or warfarin with vismodegib revealed no clinically relevant impact on CYP2C8- or CYP2C9-mediated metabolism, respectively [Citation66]. Furthermore, a follow-up study in patients with cancer revealed rosiglitazone pharmacokinetics were not impacted by vismodegib coadministration [Citation67].
Pop PK modeling for sonidegib based on 200-mg daily doses administered to patients with cancer revealed the steady-state Cmax (Css) was 1030 ng/mL [Citation37]. In contrast, the Css for vismodegib under 150-mg daily dosing was approximately 9605.6 ng/mL, as determined by pop PK [Citation68]. Human clinical data revealed that the time to reach steady-state plasma concentrations (Tss) was approximately 4 months for sonidegib; in comparison, Tss was achieved at 7–21 days with repeated dosing for vismodegib [Citation37,Citation38,Citation48,Citation69]. Notably, the formation of sonidegib and vismodegib metabolites in the circulation is relatively slow with a Tmax of 60 and 24 hours, respectively [Citation50,Citation70]. In vitro assessments determined that the primary metabolite of sonidegib (NVP-LGE899; M48) does not have any off-target impact on the tested array of transporters, ion channels, nuclear receptors, and enzymes [Citation37]. Vismodegib is primarily metabolized to oxidative metabolites (M1, M3, and M14), which are reportedly active, but no further assessments have been conducted to determine their relative activity to vismodegib [Citation38,Citation71].
Additional in vitro studies assessed the inhibitory potential of these HHIs on various drug transporters and found that sonidegib has an inhibitory effect on the breast cancer resistance protein (BRCP) efflux transporter (half-maximal effective concentration [EC50], 1.5 µM), whereas vismodegib does not appear to impact P-glycoprotein, assessed in MDR1-MDCK cells carrying the gene for P-glycoproteins (ABCB1), or BRCP efflux [Citation37,Citation38].
2.4. Elimination
Another substantial pharmacokinetic difference between the two HHIs is evident in their respective elimination half-lives (T1/2) in cancer patients, which were determined by pop PK modeling. The mean T1/2 for a single dose of sonidegib in healthy volunteers was approximately 8.7–13 days versus 28–30 days in cancer patients [Citation37,Citation50,Citation63]. For a single dose of vismodegib, the T1/2 was approximately 9–13 days in healthy volunteers and approximately 4 days in cancer patients after continuous daily dosing [Citation38,Citation41,Citation48,Citation49]. Unabsorbed sonidegib or vismodegib and their metabolites are preferentially excreted from the body in the feces (93% and 82% of the administered parent dose recovered, respectively) rather than the urine (2% and 4%, respectively) [Citation41,Citation49,Citation50].
3. Pharmacodynamics of sonidegib and vismodegib
The efficacy, safety, and tolerability of sonidegib were assessed in the BOLT trial (NCT01327053), and vismodegib outcomes were assessed in the ERIVANCE (NCT00833417), STEVIE (NCT01367665), and MIKIE (NCT01815840) trials [Citation43,Citation46,Citation57,Citation58] and in patients with hepatic impairment (discussed in the next section) [Citation51]. The pharmacodynamic marker for assessing efficacy of each inhibitor was the reduction in GLI1 expression, a downstream component of the HH pathway [Citation43,Citation44,Citation54,Citation60]. Although there are considerable differences in the efficacy assessment criteria for sonidegib and vismodegib in their respective clinical trials, the results from the BOLT and ERIVANCE studies are appropriate for comparative discussion. A recognized expert panel of clinicians, who treat laBCC and are involved in developing national/international treatment guidelines, dissected the results of the BOLT and ERIVANCE trials and concluded both inhibitors share a similar efficacy profile [Citation72]. TEAEs were among the most common reason for treatment discontinuation for both sonidegib and vismodegib in clinical trials (approximately 53% and 57% of patients respectively); among the most common TEAEs were muscle spasms and fatigue [Citation72].
Characterization of the pharmacokinetic-pharmacodynamic (PK/PD) relationship was another critical step in the clinical development of each inhibitor. To determine the risk of prolonging cardiac repolarization, concentration QTc modeling was performed to determine the impact of a drug on the cardiac QTc interval. Neither sonidegib nor vismodegib had a clinically meaningful change in QTc compared with baseline [Citation37,Citation38]. However, this concentration–effect relationship for vismodegib was primarily determined using total concentrations of vismodegib, which may have limitations due to its nonlinear and AAG-dependent pharmacokinetics. In contrast, even though steady-state concentrations of sonidegib are higher than are achieved by a single-dose administration, no dedicated thorough QTc study was conducted. Additional PK/PD modeling revealed increasing sonidegib exposure significantly correlated with an increased risk of common terminology criteria for adverse events Grade 3/4 creatine phosphokinase (CK) elevation, in which dose modifications are recommended [Citation37]. Given musculoskeletal adverse reactions (muscle spasms and arthralgias) are reported to occur with vismodegib treatment, it is recommended to monitor CK levels during treatment and interrupt or discontinue treatment depending on the severity of symptoms [Citation41].
4. HHIs in special patient populations
For sonidegib, pop PK modeling showed that age, body weight, sex, and ethnicity had no clinically meaningful effect on steady-state exposure [Citation42,Citation63]. The effect of hepatic dysfunction on the pharmacokinetics of sonidegib (single 800-mg dose) was assessed in a Phase 1, open-label study [Citation73]. Sonidegib was well tolerated, and no dose adjustments were necessary in patients with mild, moderate, or severe hepatic impairment. Although a formal clinical study has yet to be conducted in patients with chronic kidney disease, because only 2% of the administered sonidegib dose is excreted in the urine, a dose adjustment is unlikely to be necessary in this patient population [Citation50].
Similarly, for vismodegib, neither age, body weight, nor renal impairment had any clinically reported relevant effects on its systemic exposure [Citation41]. Furthermore, a Phase 1b study was conducted that revealed hepatic impairment had no impact on vismodegib pharmacokinetics, and therefore no dose adjustments are warranted in this patient population [Citation51]. However, an increasing number of post-marketing cases have been submitted to the FDA Adverse Events Reporting System regarding vismodegib-associated liver dysfunction and hepatotoxicity [Citation74,Citation75].
4.1. HHI-Resistant BCC patient population
Despite the significant clinical benefit conferred by treatment with either HHI, a subset of patients with BCC who receive sonidegib or vismodegib can exhibit resistance (intrinsic resistance) or develop resistance to treatment following an initial response (developed resistance). The underlying molecular mechanism responsible for this resistance is presumed to primarily be due to SMO mutations, which either disrupt the drug-binding pocket of these small-molecule SMO inhibitors or noncanonically activate SMO [Citation76–79]. Based on the results of an open-label study that assessed the therapeutic utility of sonidegib in vismodegib-resistant patients with BCC, specific residue mutations that account for sonidegib resistance were identified. Mutations that may confer resistance include those in residues Q477 and D473 within the drug-binding site of sonidegib and S533 and W535, which cause conformational changes outside of the binding pocket that render the drug-binding pocket inaccessible to sonidegib [Citation80]. Molecular analyses of patients that reported vismodegib treatment resistance (either intrinsic or acquired) revealed D473 involvement and specific missense SMO mutations (D473G and D473H) [Citation6,Citation76,Citation79]. Based on in silico modeling, intrinsic resistance was associated with a SMO G497W mutation, which interferes with vismodegib entry to the SMO binding site. Acquired resistance was associated with a SMO D473Y mutation that directly interferes with vismodegib binding affinity [Citation79].
Albeit to a lesser extent, alterations in components downstream of SMO such as GLI transcription factors or Suppressor of Fused (SUFU), an HH pathway negative regulator that binds and sequesters GLI in the cytoplasm, may also contribute to HHI resistance [Citation78]. As such, the management of patients with HHI resistance can include combinational therapy, on-off treatment schedules, switching HHIs agents, or concomitantly targeting downstream HH effectors [Citation6,Citation81].
4.2. HHIs: Carcinogenic, mutagenic, and teratogenic potential
Although preclinical data found no carcinogenic potential for vismodegib, studies with sonidegib have not yet been performed [Citation41,Citation42]. Neither HHI was found to be mutagenic based on in vitro bacterial reverse mutation assays [Citation41,Citation42]. However, due to the essential role of the HH pathway as a key signaling regulator in embryonic development and the mechanism of action for HHIs, their use possesses a significant teratogenic potential.
Although there is no available data on the use of sonidegib or vismodegib in pregnant women, preclinical studies have been undertaken to determine the extent HHIs may interfere with fertility. When administered to female rats, there was an increased number of resorptions/decreased implantations, a reduction in number of pregnancies, and a decreased number of viable embryos associated with sonidegib and vismodegib (at approximately 1.3- and 1.2-times the human dose, respectively) [Citation41,Citation42]. However, no adverse effects on fertility or reproductive organs were observed in male rats administered either HHI [Citation41,Citation42]. Given the risk of embryofetal death or severe birth defects associated with oncology pharmaceuticals such as HHIs and preclinical findings, it is recommended women taking sonidegib should refrain from getting pregnant during and for ≥20 months following treatment [Citation42]. Since the potential risk of exposure can also be through semen, men are advised to use effective contraception and to refrain from donating semen for ≥8 months after ending treatment [Citation42]. The recommended duration of pregnancy prevention in female patients and female sexual partners of male patients after the last dose of vismodegib is ≥24 months, and since vismodegib is reportedly present in semen, ≥3 months for male patients [Citation41]. These recommendations are based on US FDA guidelines for oncology pharmaceuticals with reproductive toxicity, which takes into account relative genotoxicity and T1/2 of each individual HHI [Citation82].
5. Conclusions
The significant differences among the pharmacokinetic profiles of sonidegib and vismodegib such as volume of distribution, elimination half-life, and time to achieve steady state may have a more significant clinical impact than initially expected. TEAEs were the most common reason for treatment discontinuation for both sonidegib and vismodegib in clinical trials (approximately 53% and 57% of patients respectively) that potentially could be contributed to by their differences of the pharmacokinetic profiles [Citation72]. Although data reported in clinical trials show slightly less severe and less frequent TEAEs for sonidegib versus vismodegib, as well as a slightly delayed onset of TEAEs with sonidegib, without head-to-head comparisons, only evidence-based expert recommendations offer assistance for clinicians whether to treat patients with either sonidegib or vismodegib [Citation8,Citation83].
6. Expert opinion
Sonidegib and vismodegib represent first-in-class drugs for the treatment of advanced or surgery/radiation therapy refractory BCC that have improved outcomes for patients since their respective FDA approvals in 2015 and 2012. Despite having an identical therapeutic molecular target, it is evident based on the literature and peer-reviewed published data that sonidegib and vismodegib have very different pharmacokinetic profiles. Differences such as the lipophilicity, volume of distribution, and terminal half-lives of the two HHIs may play a potentially important role in their efficacy and safety. Notably, the pharmacokinetic properties of sonidegib are dose proportional, whereas vismodegib are non-dose proportional, potentially allowing for sonidegib to be more amenable to alternate dosing regimens that could potentially improve tolerability while maintaining efficacy. From the perspective of a patient, one difference between the two HHIs beyond the pharmacologic differences mentioned is the daily recommended dose and method of administration. Sonidegib 200 mg daily should be taken on an empty stomach (≥1 hour before or 2 hours after a meal), whereas vismodegib 150 mg daily can be taken with or without food [Citation41,Citation42].
In the absence of head-to-head clinical trial comparisons, the extent of the clinical importance of these pharmacokinetic differences is not fully determined. Currently, clinicians are limited to evidence-based recommendations to discern meaningful differences when choosing between the two drugs. Accordingly, clinical trials that directly compare sonidegib and vismodegib are essential to provide conclusive evidence of potential clinically meaningful differences in tolerability and efficacy between these two HHIs, facilitating more categorical evidence-based recommendations for clinicians. In the interim, since sonidegib and vismodegib have been approved for 8 and 11 years, respectively, analyses of real-world clinical data may potentially serve as an additional metric to evidence-based recommendations in the absence of comparative clinical trials. Real-world data sets may also provide critical insight on how intermittent dosing or concomitant therapies affect HHI treatment as well as how various patient populations potentially not represented in clinical trials may respond to treatment [Citation84]. However, interpreting real-world efficacy data can be inherently difficult due to high discontinuation rates, short times to discontinuation, low rates of re-initiation commonly experienced by patients with BCC, and variability among patient populations, which may unpredictably impact the clinical effectiveness of these treatments [Citation85,Citation86].
For the subpopulation of individuals with BCC and resistance (intrinsic or developed) to sonidegib or vismodegib, future studies should explore the use of these inhibitors in combination with other HHIs that target downstream molecular effectors in the HH pathway. Given the limited therapeutic options for patients with advanced refractory BCC and the continuous development of other similar compounds in clinical trials that target various molecular components of the HH pathway, this signaling pathway presents a therapeutic target that has successfully shown durable responses for the treatment of BCC [Citation87]. Among these various compounds under clinical investigation are patidegib (saridegib or IPI-926) [Citation33,Citation88–90] and taladegib (LY2940680) [Citation91,Citation92]; both SMO inhibitors demonstrated antitumor activity with a manageable safety and tolerability profile in patients with BCC. These two HHIs are also being assessed for their pharmacologic potential in other types of cancers [Citation93].
Beyond enhancing the therapeutic index of HHIs currently approved and/or in development, immunotherapy offers an alternative and promising clinical approach that could be used in combination with HHIs. For example, cemiplimab (REGN2810; Libtayo®, Regeneron Pharmaceuticals, Inc.) was the first FDA-approved immunotherapy for patients with BCC [Citation94,Citation95]. Cemiplimab is a monoclonal antibody that binds to and inhibits programmed death receptor-1 (PD) or PD-1 ligand 1 (PD-L1), thereby minimizing its negative modulation of the immune response. As the only approved treatment option for locally advanced and metastatic cutaneous squamous cell carcinoma in patients who are not candidates for curative surgery or radiation [Citation95], cemiplimab clinical trials and real-world data exemplify the utility of this class of inhibitors [Citation96]. With regard to BCC, cemiplimab is currently only approved for patients with laBCC and mBCC who are intolerant to HHI treatment or were previously treated with an HHI and did not respond [Citation95]. The first clinical case of cemiplimab treatment was in a patient with mBCC, which resulted in an overall durable and partial response to treatment [Citation97]. Shortly thereafter, a Phase 2 clinical trial that assessed cemiplimab monotherapy among patients with laBCC after HHI treatment demonstrated clinically meaningful antitumor activity and an acceptable safety profile consistent with cemiplimab in other indications [Citation98].
For the time being, HHIs still represent the first-line pharmacologic approach while immune checkpoint inhibitors are a second-line treatment for circumstances in which HHIs are found to be ineffective or in cases of persisting toxicity [Citation99]. Emerging studies are elucidating the modulatory role of HH pathway signaling and HHIs on tumor immunity in a variety of cancers [Citation100–102]. Consistent with these findings, clinical evidence demonstrates HHI-induced BCC tumor regression is accompanied by activation of the adaptive immune system [Citation103]. As the scientific basis of the molecular mechanisms that underlie BCC HHI resistance are more fully elucidated, treatment recommendations in the near future may include HHI/immunotherapy combination approaches as the new standard-of-care treatment option for patients with advanced refractory BCC. However, preclinical and clinical studies are needed to fully characterize the clinical efficacy and safety of combining immune checkpoint inhibitors and HHIs in patients with BCC.
To circumvent resistance to SMO antagonists, a number of novel pharmacologic agents that target terminal effectors of the HH pathway are being developed and tested in clinical trials [Citation81]. Novel combinatorial strategies that complement the demonstrated clinical benefit conferred by sonidegib and vismodegib may well lead to significant outcome improvements in patients with advanced refractory BCC, as well as current HHI treatment-resistant subpopulations. An alternative and emerging approach to overcoming treatment resistance lies with second-generation HHIs. Compounds that retain antitumor activity in the presence of resistance-conferring mutations are currently being screened and assessed in preclinical studies [Citation33]. Despite the considerable therapeutic potential of combinational treatment approaches, it must be emphasized that only direct head-to-head comparative clinical trials will provide the ultimate ‘holy grail’ of clinical evidence-based guidance.
Article highlights
The small-molecule inhibitors sonidegib and vismodegib are currently the only approved Hedgehog pathway inhibitors (HHIs) for the treatment of advanced basal cell carcinoma (BCC) that is not amenable to conventional surgery or radiotherapy.
However, currently there are no head-to-head clinical trials comparing these two HHIs that would provide evidence-based guidance for treating clinicians.
Despite similar indications, the pharmacokinetic profiles of sonidegib and vismodegib are notably different, including their volumes of distribution and elimination half-lives.
Until comparative studies are conducted to determine the clinical relevance of the reported differences in their pharmacokinetic properties, evidence-based recommendations using available preclinical and clinical data must be utilized to bridge this gap.
Declaration of interest
JT Lear has received personal fees from Sun Pharma. LD Lewis has received funding support via his institution for clinical trials involving sonidegib and vismodegib from Sun Pharma and Genentech, respectively, and is a paid consultant to G1 Therapeutics and 7 Hills Pharm LLC. The authors have no other relevant affiliations or financial involvement with any organization or entity with a financial interest in or financial conflict with the subject matter or materials discussed in the manuscript apart from those disclosed.
Reviewer disclosures
Peer reviewers on this manuscript have no relevant financial relationships or otherwise to disclose.
Acknowledgments
The authors thank Alexander S. Milliken, AlphaBioCom, a Red Nucleus company, for their medical writing and editorial support.
Additional information
Funding
References
- Asgari MM, Moffet HH, Ray GT, et al. Trends in basal cell carcinoma incidence and identification of high-risk subgroups, 1998-2012. JAMA Dermatol. 2015;151(9):976–981. doi: 10.1001/jamadermatol.2015.1188
- Leiter U, Eigentler T, Garbe C. Epidemiology of skin cancer. Adv Exp Med Biol. 2014;810:120–140.
- Bichakjian C, Armstrong A, Baum C, et al. Guidelines of care for the management of basal cell carcinoma. J Am Acad Dermatol. 2018;78(3):540–559. doi: 10.1016/j.jaad.2017.10.006
- Peris K, Fargnoli MC, Garbe C, et al. Diagnosis and treatment of basal cell carcinoma: European consensus-based interdisciplinary guidelines. Eur J Cancer. 2019;118:10–34. doi: 10.1016/j.ejca.2019.06.003
- NCCN Clinical Practice Guidelines® in oncology: basal cell skin cancer. Version. 2023;1(2023). Available at: https://www.nccn.org/guidelines/guidelines-detail?category=1&id=1416
- Doan HQ, Chen L, Nawas Z, et al. Switching Hedgehog inhibitors and other strategies to address resistance when treating advanced basal cell carcinoma. Oncotarget. 2021;12(20):2089–2100. doi: 10.18632/oncotarget.28080
- Lear JT, Corner C, Dziewulski P, et al. Challenges and new horizons in the management of advanced basal cell carcinoma: a UK perspective. Br J Cancer. 2014;111(8):1476–1481. doi: 10.1038/bjc.2014.270
- Lear JT, Dummer R, Guminski A. Using drug scheduling to manage adverse events associated with hedgehog pathway inhibitors for basal cell carcinoma. Oncotarget. 2021;12(26):2531–2540. doi: 10.18632/oncotarget.28145
- Epstein EH. Basal cell carcinomas: attack of the hedgehog. Nat Rev Cancer. 2008;8(10):743–754. doi: 10.1038/nrc2503
- Varjosalo M, Taipale J. Hedgehog: functions and mechanisms. Genes Dev. 2008;22(18):2454–2472. doi: 10.1101/gad.1693608
- Hahn H, Wicking C, Zaphiropoulous PG, et al. Mutations of the human homolog of drosophila patched in the nevoid basal cell carcinoma syndrome. Cell. 1996;85(6):841–851. doi: 10.1016/S0092-8674(00)81268-4
- Johnson RL, Rothman AL, Xie J, et al. Human homolog of patched, a candidate gene for the basal cell nevus syndrome. Science. 1996;272(5268):1668–1671. doi: 10.1126/science.272.5268.1668
- Gailani MR, Stahle-Backdahl M, Leffell DJ, et al. The role of the human homologue of drosophila patched in sporadic basal cell carcinomas. Nat Genet. 1996;14(1):78–81. doi: 10.1038/ng0996-78
- Trieu KG, Tsai SY, Eberl M, et al. Basal cell carcinomas acquire secondary mutations to overcome dormancy and progress from microscopic to macroscopic disease. Cell Rep. 2022;39(5):110779. doi: 10.1016/j.celrep.2022.110779
- Pellegrini C, Maturo MG, Di Nardo L, et al. Understanding the molecular genetics of basal cell carcinoma. Int J Mol Sci. 2017;18(11):2485. doi: 10.3390/ijms18112485
- Xie J, Murone M, Luoh SM, et al. Activating smoothened mutations in sporadic basal-cell carcinoma. Nature. 1998;391(6662):90–92. doi: 10.1038/34201
- Dahmane N, Lee J, Robins P, et al. Activation of the transcription factor Gli1 and the Sonic hedgehog signalling pathway in skin tumours. Nature. 1997;389(6653):876–881. doi: 10.1038/39918
- Hutchin ME, Kariapper MS, Grachtchouk M, et al. Sustained Hedgehog signaling is required for basal cell carcinoma proliferation and survival: conditional skin tumorigenesis recapitulates the hair growth cycle. Genes Dev. 2005;19(2):214–223. doi: 10.1101/gad.1258705
- Niewiadomski P, Niedziolka SM, Markiewicz L, et al. Gli proteins: regulation in development and cancer. Cells. 2019;8(2):147. doi: 10.3390/cells8020147
- Kogerman P, Grimm T, Kogerman L, et al. Mammalian suppressor-of-fused modulates nuclear-cytoplasmic shuttling of gli-1. Nat Cell Biol. 1999;1(5):312–319. doi: 10.1038/13031
- Gutzmer R, Solomon JA. Hedgehog pathway inhibition for the treatment of basal cell carcinoma. Target Oncol. 2019;14(3):253–267. doi: 10.1007/s11523-019-00648-2
- Maun HR, Wen X, Lingel A, et al. Hedgehog pathway antagonist 5E1 binds hedgehog at the pseudo-active site. J Biol Chem. 2010;285(34):26570–26580. doi: 10.1074/jbc.M110.112284
- Stanton BZ, Peng LF, Maloof N, et al. A small molecule that binds Hedgehog and blocks its signaling in human cells. Nat Chem Biol. 2009;5(3):154–156. doi: 10.1038/nchembio.142
- Bosanac I, Maun HR, Scales SJ, et al. The structure of SHH in complex with HHIP reveals a recognition role for the shh pseudo active site in signaling. Nat Struct Mol Biol. 2009;16(7):691–697. doi: 10.1038/nsmb.1632
- Yun T, Wang J, Yang J, et al. Discovery of small molecule inhibitors targeting the sonic Hedgehog. Front Chem. 2020;8:498. doi: 10.3389/fchem.2020.00498
- Petrova E, Rios-Esteves J, Ouerfelli O, et al. Inhibitors of Hedgehog acyltransferase block sonic Hedgehog signaling. Nat Chem Biol. 2013;9(4):247–249. doi: 10.1038/nchembio.1184
- Lauth M, Bergstrom A, Shimokawa T, et al. Inhibition of GLI-mediated transcription and tumor cell growth by small-molecule antagonists. Proc Natl Acad Sci U S A. 2007;104(20):8455–8460. doi: 10.1073/pnas.0609699104
- Beauchamp EM, Ringer L, Bulut G, et al. Arsenic trioxide inhibits human cancer cell growth and tumor development in mice by blocking Hedgehog/GLI pathway. J Clin Invest. 2011;121(1):148–160. doi: 10.1172/JCI42874
- Kim J, Lee JJ, Kim J, et al. Arsenic antagonizes the Hedgehog pathway by preventing ciliary accumulation and reducing stability of the Gli2 transcriptional effector. Proc Natl Acad Sci U S A. 2010;107(30):13432–13437. doi: 10.1073/pnas.1006822107
- Berman DM, Karhadkar SS, Hallahan AR, et al. Medulloblastoma growth inhibition by hedgehog pathway blockade. Science. 2002;297(5586):1559–1561. doi: 10.1126/science.1073733
- Chen JK, Taipale J, Cooper MK, et al. Inhibition of Hedgehog signaling by direct binding of cyclopamine to smoothened. Genes Dev. 2002;16(21):2743–2748. doi: 10.1101/gad.1025302
- Sanchez P, Ruiz i Altaba A. In vivo inhibition of endogenous brain tumors through systemic interference of Hedgehog signaling in mice. Mech Dev. 2005;122(2):223–230. doi: 10.1016/j.mod.2004.10.002
- Nguyen NM, Cho J. Hedgehog pathway inhibitors as targeted cancer therapy and strategies to overcome drug resistance. Int J Mol Sci. 2022;23(3):1733. doi: 10.3390/ijms23031733
- Winkler JD, Isaacs A, Holderbaum L, et al. Design and synthesis of inhibitors of Hedgehog signaling based on the alkaloid cyclopamine. Org Lett. 2009;11(13):2824–2827. doi: 10.1021/ol900974u
- Gould SE, Low JA, Marsters JC Jr., et al. Discovery and preclinical development of vismodegib. Expert Opin Drug Discov. 2014;9(8):969–984. doi: 10.1517/17460441.2014.920816
- Cooper MK, Porter JA, Young KE, et al. Teratogen-mediated inhibition of target tissue response to Shh signaling. Science. 1998;280(5369):1603–1607. doi: 10.1126/science.280.5369.1603
- European Medicines Agency. Assessment report for ODOMZO. London (UK): European Medicines Agency; 2015.
- European Medicines Agency. Assessment report for Erivedge. London (UK): European Medicines Agency; 2016.
- Axelson M, Liu K, Jiang X, et al. US Food and Drug Administration approval: vismodegib for recurrent, locally advanced, or metastatic basal cell carcinoma. Clin Cancer Res. 2013;19(9):2289–2293. doi: 10.1158/1078-0432.CCR-12-1956
- Casey D, Demko S, Shord S, et al. FDA approval summary: sonidegib for locally advanced basal cell carcinoma. Clin Cancer Res. 2017;23(10):2377–2381. doi: 10.1158/1078-0432.CCR-16-2051
- Erivedge® (vismodegib capsules) Prescribing Information (2023). Available at: https://www.gene.com/download/pdf/erivedge_prescribing.pdf
- ODOMZO® (sonidegib capsules) Prescribing Information (2022). Available at: https://www.odomzo.com/themes/custom/odomzo/global/pdfs/pi.pdf
- Migden MR, Guminski A, Gutzmer R, et al. Treatment with two different doses of sonidegib in patients with locally advanced or metastatic basal cell carcinoma (BOLT): a multicentre, randomised, double-blind phase 2 trial. Lancet Oncol. 2015;16(6):716–728. doi: 10.1016/S1470-2045(15)70100-2
- Sekulic A, Migden MR, Oro AE, et al. Efficacy and safety of vismodegib in advanced basal-cell carcinoma. N Engl J Med. 2012;366(23):2171–2179. doi: 10.1056/NEJMoa1113713
- Dummer R, Guminksi A, Gutzmer R, et al. Long-term efficacy and safety of sonidegib in patients with advanced basal cell carcinoma: 42-month analysis of the phase II randomized, double-blind BOLT study. Br J Dermatol. 2020;182(6):1369–1378. doi: 10.1111/bjd.18552
- Sekulic A, Migden MR, Basset-Seguin N, et al. Long-term safety and efficacy of vismodegib in patients with advanced basal cell carcinoma: final update of the pivotal ERIVANCE BCC study. BMC Cancer. 2017;17(1):332. doi: 10.1186/s12885-017-3286-5
- Ding X, Chou B, Graham RA, et al. Determination of GDC-0449, a small-molecule inhibitor of the Hedgehog signaling pathway, in human plasma by solid phase extraction-liquid chromatographic-tandem mass spectrometry. J Chromatogr B Analyt Technol Biomed Life Sci. 2010;878(9–10):785–790. doi: 10.1016/j.jchromb.2010.01.039
- Graham RA, Hop CE, Borin MT, et al. Single and multiple dose intravenous and oral pharmacokinetics of the hedgehog pathway inhibitor vismodegib in healthy female subjects. Br J Clin Pharmacol. 2012;74(5):788–796. doi: 10.1111/j.1365-2125.2012.04281.x
- Graham RA, Lum BL, Morrison G, et al. A single dose mass balance study of the Hedgehog pathway inhibitor vismodegib (GDC-0449) in humans using accelerator mass spectrometry. Drug Metab Dispos. 2011;39(8):1460–1467. doi: 10.1124/dmd.111.039339
- Zollinger M, Lozac’h F, Hurh E, et al. Absorption, distribution, metabolism, and excretion (ADME) of (1)(4)C-sonidegib (LDE225) in healthy volunteers. Cancer Chemother Pharmacol. 2014;74(1):63–75. doi: 10.1007/s00280-014-2468-y
- Abou-Alfa GK, Lewis LD, LoRusso P, et al. Pharmacokinetics and safety of vismodegib in patients with advanced solid malignancies and hepatic impairment. Cancer Chemother Pharmacol. 2017;80(1):29–36. doi: 10.1007/s00280-017-3315-8
- Graham RA, Lum BL, Cheeti S, et al. Pharmacokinetics of hedgehog pathway inhibitor vismodegib (GDC-0449) in patients with locally advanced or metastatic solid tumors: the role of alpha-1-acid glycoprotein binding. Clin Cancer Res. 2011;17(8):2512–2520. doi: 10.1158/1078-0432.CCR-10-2736
- Lorusso PM, Jimeno A, Dy G, et al. Pharmacokinetic dose-scheduling study of hedgehog pathway inhibitor vismodegib (GDC-0449) in patients with locally advanced or metastatic solid tumors. Clin Cancer Res. 2011;17(17):5774–5782. doi: 10.1158/1078-0432.CCR-11-0972
- Rodon J, Tawbi HA, Thomas AL, et al. A phase I, multicenter, open-label, first-in-human, dose-escalation study of the oral smoothened inhibitor sonidegib (LDE225) in patients with advanced solid tumors. Clin Cancer Res. 2014;20(7):1900–1909. doi: 10.1158/1078-0432.CCR-13-1710
- Sharma MR, Karrison TG, Kell B, et al. Evaluation of food effect on pharmacokinetics of vismodegib in advanced solid tumor patients. Clin Cancer Res. 2013;19(11):3059–3067. doi: 10.1158/1078-0432.CCR-12-3829
- Zhou J, Quinlan M, Hurh E, et al. Exposure-response analysis of sonidegib (LDE225), an oral inhibitor of the Hedgehog signaling pathway, for effectiveness and safety in patients with advanced solid tumors. J Clin Pharmacol. 2016;56(11):1406–1415. doi: 10.1002/jcph.749
- Basset-Seguin N, Hauschild A, Kunstfeld R, et al. Vismodegib in patients with advanced basal cell carcinoma: primary analysis of STEVIE, an international, open-label trial. Eur J Cancer. 2017;86:334–348. doi: 10.1016/j.ejca.2017.08.022
- Dreno B, Kunstfeld R, Hauschild A, et al. Two intermittent vismodegib dosing regimens in patients with multiple basal-cell carcinomas (MIKIE): a randomised, regimen-controlled, double-blind, phase 2 trial. Lancet Oncol. 2017;18(3):404–412. doi: 10.1016/S1470-2045(17)30072-4
- Einolf HJ, Zhou J, Won C, et al. A physiologically-based pharmacokinetic modeling approach to predict drug-drug interactions of sonidegib (LDE225) with perpetrators of CYP3A in cancer patients. Drug Metab Dispos. 2017;45(4):361–374. doi: 10.1124/dmd.116.073585
- Pan S, Wu X, Jiang J, et al. Discovery of NVP-LDE225, a potent and selective smoothened antagonist. ACS Med Chem Lett. 2010;1(3):130–134. doi: 10.1021/ml1000307
- Giannetti AM, Wong H, Dijkgraaf GJ, et al. Identification, characterization, and implications of species-dependent plasma protein binding for the oral Hedgehog pathway inhibitor vismodegib (GDC-0449). J Med Chem. 2011;54(8):2592–2601. doi: 10.1021/jm1008924
- Campione E, Di Prete M, Lozzi F, et al. High-risk recurrence basal cell carcinoma: focus on Hedgehog pathway inhibitors and review of the literature. Chemotherapy. 2020;65(1–2):2–10. doi: 10.1159/000509156
- Goel V, Hurh E, Stein A, et al. Population pharmacokinetics of sonidegib (LDE225), an oral inhibitor of hedgehog pathway signaling, in healthy subjects and in patients with advanced solid tumors. Cancer Chemother Pharmacol. 2016;77(4):745–755. doi: 10.1007/s00280-016-2982-1
- Pooler DB, Ness DB, Sarantopoulos J, et al. The effect of sonidegib (LDE225) on the pharmacokinetics of bupropion and warfarin in patients with advanced solid tumours. Br J Clin Pharmacol. 2021;87(3):1291–1302. doi: 10.1111/bcp.14508
- Malhi V, Colburn D, Williams SJ, et al. A clinical drug-drug interaction study to evaluate the effect of a proton-pump inhibitor, a combined P-glycoprotein/cytochrome 450 enzyme (CYP)3A4 inhibitor, and a CYP2C9 inhibitor on the pharmacokinetics of vismodegib. Cancer Chemother Pharmacol. 2016;78(1):41–49. doi: 10.1007/s00280-016-3020-z
- Wong H, Chen JZ, Chou B, et al. Preclinical assessment of the absorption, distribution, metabolism and excretion of GDC-0449 (2-chloro-N-(4-chloro-3-(pyridin-2-yl)phenyl)-4-(methylsulfonyl)benzamide), an orally bioavailable systemic Hedgehog signalling pathway inhibitor. Xenobiotica. 2009;39(11):850–861. doi: 10.3109/00498250903180289
- LoRusso PM, Piha-Paul SA, Mita M, et al. Co-administration of vismodegib with rosiglitazone or combined oral contraceptive in patients with locally advanced or metastatic solid tumors: a pharmacokinetic assessment of drug-drug interaction potential. Cancer Chemother Pharmacol. 2013;71(1):193–202. doi: 10.1007/s00280-012-1996-6
- Lu T, Wang B, Gao Y, et al. Semi-mechanism-based population pharmacokinetic modeling of the Hedgehog pathway inhibitor vismodegib. CPT Pharmacometrics Syst Pharmacol. 2015;4(11):680–689. doi: 10.1002/psp4.12039
- Villani A, Fabbrocini G, Costa C, et al. Sonidegib: safety and efficacy in treatment of advanced basal cell carcinoma. Dermatol Ther. 2020;10(3):401–412. doi: 10.1007/s13555-020-00378-8
- Jain S, Song R, Xie J. Sonidegib: mechanism of action, pharmacology, and clinical utility for advanced basal cell carcinomas. Onco Targets Ther. 2017;10:1645–1653. doi: 10.2147/OTT.S130910
- Cancer Care Ontario. Drug monograph: Vismodegib (2019). Available at: https://www.cancercareontario.ca/en/drugformulary/drugs/vismodegib
- Dummer R, Ascierto PA, Basset-Seguin N, et al. Sonidegib and vismodegib in the treatment of patients with locally advanced basal cell carcinoma: a joint expert opinion. J Eur Acad Dermatol Venereol. 2020;34(9):1944–1956. doi: 10.1111/jdv.16230
- Horsmans Y, Zhou J, Liudmila M, et al. Effects of mild to severe hepatic impairment on the pharmacokinetics of sonidegib: a multicenter, open-label, parallel-group study. Clin Pharmacokinet. 2018;57(3):345–354. doi: 10.1007/s40262-017-0560-2
- Ventarola DJ, Silverstein DI. Vismodegib-associated hepatotoxicity: a potential side effect detected in postmarketing surveillance. J Am Acad Dermatol. 2014;71(2):397–398. doi: 10.1016/j.jaad.2014.04.023
- Edwards BJ, Raisch DW, Saraykar SS, et al. Hepatotoxicity with vismodegib: an MD Anderson Cancer center and research on adverse drug events and reports project. Drugs R D. 2017;17(1):211–218. doi: 10.1007/s40268-016-0168-2
- Atwood SX, Sarin KY, Whitson RJ, et al. Smoothened variants explain the majority of drug resistance in basal cell carcinoma. Cancer Cell. 2015;27(3):342–353. doi: 10.1016/j.ccell.2015.02.002
- Shimizu Y, Ishii T, Ogawa K, et al. Biochemical characterization of smoothened receptor antagonists by binding kinetics against drug-resistant mutant. Eur J Pharmacol. 2015;764:220–227. doi: 10.1016/j.ejphar.2015.05.062
- Sharpe HJ, Pau G, Dijkgraaf GJ, et al. Genomic analysis of smoothened inhibitor resistance in basal cell carcinoma. Cancer Cell. 2015;27(3):327–341. doi: 10.1016/j.ccell.2015.02.001
- Pricl S, Cortelazzi B, Dal Col V, et al. Smoothened (SMO) receptor mutations dictate resistance to vismodegib in basal cell carcinoma. Mol Oncol. 2015;9(2):389–397. doi: 10.1016/j.molonc.2014.09.003
- Danial C, Sarin KY, Oro AE, et al. An investigator-initiated open-label trial of sonidegib in advanced basal cell carcinoma patients resistant to vismodegib. Clin Cancer Res. 2016;22(6):1325–1329. doi: 10.1158/1078-0432.CCR-15-1588
- Heppt MV, Gebhardt C, Hassel JC, et al. Long-term management of advanced basal cell carcinoma: current challenges and future perspectives. Cancers (Basel). 2022;14(19):4547. doi: 10.3390/cancers14194547
- US Food and Drug Administration. Oncology pharmaceuticals: reproductive toxicity testing and labeling recommendations guidance for industry (2019). Available at: https://www.fda.gov/regulatory-information/search-fda-guidance-documents/oncology-pharmaceuticals-reproductive-toxicity-testing-and-labeling-recommendations-guidance
- Gutzmer R, Loquai C, Robert C, et al. Key clinical adverse events in patients with advanced basal cell carcinoma treated with sonidegib or vismodegib: a Post Hoc analysis. Dermatol Ther. 2021;11(5):1839–1849. doi: 10.1007/s13555-021-00588-8
- Grossmann LE, Ramelyte E, Nageli MC, et al. Eight years of real-life experience with smoothened inhibitors in a Swiss Tertiary skin Referral center. Cancers (Basel). 2022;14(10):2496. doi: 10.3390/cancers14102496
- Ge W, Chen CI, Wu N, et al. Hedgehog pathway inhibitor real-world treatment patterns in patients with basal cell carcinoma: a claims-based analysis. Future Oncol. 2022;18(23):2561–2572. doi: 10.2217/fon-2022-0373
- Mannino M, Piccerillo A, Fabbrocini G, et al. Clinical characteristics of an Italian patient population with advanced BCC and real-life evaluation of HHI safety and effectiveness. Dermatology. 2023;1–9. doi: 10.1159/000531280
- Villani A, Potestio L, Fabbrocini G, et al. New emerging treatment options for advanced basal cell carcinoma and squamous cell carcinoma. Adv Ther. 2022;39(3):1164–1178. doi: 10.1007/s12325-022-02044-1
- Jimeno A, Weiss GJ, Miller WH Jr., et al. Phase I study of the Hedgehog pathway inhibitor IPI-926 in adult patients with solid tumors. Clin Cancer Res. 2013;19(10):2766–2774. doi: 10.1158/1078-0432.CCR-12-3654
- Trial of patidegib gel 2%, 4%, and vehicle to decrease the number of surgically eligible basal cell carcinomas in gorlin syndrome patients. Available at: https://clinicaltrials.gov/study/NCT02762084
- Clinical trial of patidegib gel 2%, 4%, and vehicle applied once or twice daily to decrease the GLI1 biomarker in sporadic nodular basal cell carcinomas (BCC). Available at: https://clinicaltrials.gov/study/NCT02828111
- Bendell J, Andre V, Ho A, et al. Phase I study of LY2940680, a Smo antagonist, in patients with advanced cancer including treatment-naive and previously treated basal cell carcinoma. Clin Cancer Res. 2018;24(9):2082–2091. doi: 10.1158/1078-0432.CCR-17-0723
- Ueno H, Kondo S, Yoshikawa S, et al. A phase I and pharmacokinetic study of taladegib, a Smoothened inhibitor, in Japanese patients with advanced solid tumors. Invest New Drugs. 2018;36(4):647–656. doi: 10.1007/s10637-017-0544-y
- Xie H, Paradise BD, Ma WW, et al. Recent advances in the clinical targeting of Hedgehog/GLI signaling in cancer. Cells. 2019;8(5):394. doi: 10.3390/cells8050394
- US Food and Drug Administration. FDA approves first treatment for advanced form of the second most common skin cancer (2018). Available at: https://www.fda.gov/news-events/press-announcements/fda-approves-first-treatment-advanced-form-second-most-common-skin-cancer
- LIBTAYO® (cemiplimab-rwlc) highlights of Prescribing information (2022). Available at: https://www.accessdata.fda.gov/drugsatfda_docs/label/2022/761097s014lbl.pdf
- Villani A, Ocampo-Garza SS, Potestio L, et al. Cemiplimab for the treatment of advanced cutaneous squamous cell carcinoma. Expert Opin Drug Saf. 2022;21(1):21–29. doi: 10.1080/14740338.2022.1993819
- Falchook GS, Leidner R, Stankevich E, et al. Responses of metastatic basal cell and cutaneous squamous cell carcinomas to anti-PD1 monoclonal antibody REGN2810. J Immunother Cancer. 2016;4(1):70. doi: 10.1186/s40425-016-0176-3
- Stratigos AJ, Sekulic A, Peris K, et al. Cemiplimab in locally advanced basal cell carcinoma after hedgehog inhibitor therapy: an open-label, multi-centre, single-arm, phase 2 trial. Lancet Oncol. 2021;22(6):848–857. doi: 10.1016/S1470-2045(21)00126-1
- Bossi P, Ascierto PA, Basset-Seguin N, et al. Long-term strategies for management of advanced basal cell carcinoma with hedgehog inhibitors. Crit Rev Oncol Hematol. 2023;189:104066. doi: 10.1016/j.critrevonc.2023.104066
- Wang J, Cui B, Li X, et al. The emerging roles of Hedgehog signaling in tumor immune microenvironment. Front Oncol. 2023;13:1171418. doi: 10.3389/fonc.2023.1171418
- Giammona A, Crivaro E, Stecca B. Emerging roles of Hedgehog signaling in cancer immunity. Int J Mol Sci. 2023;24(2):1321. doi: 10.3390/ijms24021321
- Gambini D, Passoni E, Nazzaro G, et al. Basal cell carcinoma and Hedgehog pathway inhibitors: focus on immune response. Front Med. 2022;9:893063. doi: 10.3389/fmed.2022.893063
- Otsuka A, Dreier J, Cheng PF, et al. Hedgehog pathway inhibitors promote adaptive immune responses in basal cell carcinoma. Clin Cancer Res. 2015;21(6):1289–1297. doi: 10.1158/1078-0432.CCR-14-2110