Abstract
Silica-supported acid catalysts were synthesized and characterized for composition, morphology, particle size distribution, and crystallinity. The catalysts are used for the esterification of long-chain aliphatic acids with alcohol to produce the corresponding ester. Process parameters such as time, temperature, and solvent ratio influence the conversion of fatty acid to ester. A supported catalyst was found to show higher catalytic activity as compared to the conventional sulfuric acid catalyst.
Introduction
Acids are an important class of catalyst systems for chemical reactions such as alkylation, esterification, sulfonation, nitration, etc. Citation1–3. To overcome drawbacks associated with the effective use of acid catalysts and to move toward more eco-friendly chemical processes, the incorporation of acids such as sulfuric, hydrochloric, and nitric acid over high-surface area supports, is one of the current approaches Citation4–7. For example, sulfuric acid incorporated silica catalysts are used for alkylation of paraffins Citation8–10. Other heterogeneous acid catalyst such as zeolites, clays, and ion exchange resins are also used for organic transformations Citation1, Citation11, Citation12.
Specialty chemicals based on long-chain fatty acid esters find a wide range of end use applications such as cosmetic materials, topical medicine, and polyolefin catalyst component Citation13–19. The esters are synthesized by the reaction of fatty acids with aliphatic alcohols in the presence of acids such as sulfuric acid, para-toluene sulfonic acid, and phosphoric acid as catalyst Citation20 Citation21. Heterogeneous catalyst such as ion exchange resins Citation11 Citation12 and silica-supported catalyst Citation22 are also found to be effective catalysts for esterification.
In view of our interest in polyolefin catalysis Citation23–27, we initiated a program to develop eco-friendly and high yielding processes for aliphatic/aromatic ester formation using acid incorporated supported catalysts. The present study describes the synthesis and physiochemical characteristics of morphological silica-supported acid catalysts. The synthesized catalysts were found to be effective for esterification of long-chain fatty acids.
Results and discussions
Silica-supported sulfuric acid catalysts, SiO2-1-SO4 and SiO2-2-SO4, were prepared by reacting sulfuric acid with different silica materials as represented by reaction in . Commercial silica support, SiO2-1, with spherical morphology and mean particle size of 97 µ ( and ) showed high pore volume (3 cm3/g) and diameter (24 nm) as compared to support, SiO2-2, with irregular morphology and mean particle size of 141 µ (pore volume 0.75 cm3/g, pore diameter 6 nm).
Figure 1. SEM micrographs of catalysts (a) SiO2-1; (b) SiO2-1-SO4; (c) SiO2-1-SO4-400; (d) SiO2-2, (e) SiO2-2-SO4; and (f) SiO2-2-SO4-400.
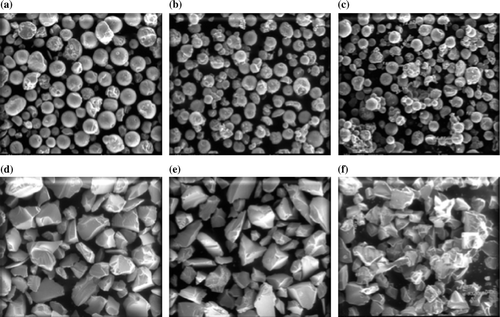
Compositional analyses of the product indicated 60.4 and 40.2 wt% of sulfuric acid for SiO2-1-SO4 and SiO2-2-SO4, respectively (). The FT-IR spectra of the support () indicate a broad signal at 3600–3200 cm−1. These vibrations are assigned to stretching bands of Si[sbnd]OH Citation28 and of mutual linkaging of Si[sbnd]OH and water molecules with silanol groups through hydrogen bonding. These assignments are in addition to bands due to molecular water bonded to each other and to silanol groups through hydrogen bonds. Vibrational absorptions of sulfuric acid are also observed in the supported catalyst Citation29. The vibration band at 1284 cm−1 in the supported catalyst is assigned to Si[sbnd]O[sbnd]S Citation30 indicating bonding of sulfuric acid over the surface of silica. The strong band at 1177 cm−1 in the catalyst is assigned to anti-symmetric S[sbnd](OH)2 bend of H2SO4 with H2O. Vibrations at 886 and 851 cm−1 are due to anti-symmetric and symmetric S[sbnd](OH)2 stretching band of H2SO4, respectively. The sharp band at 577 cm−1 is assigned to the O = S=O rocking band.
Table 1. Physico-chemical characteristics of catalysts.
The supported catalysts were calcined at 400°C for 4 hours to study the thermal effect. The compositional analysis of calcined catalysts, SiO2-1-SO4-400 and SiO2-2-SO4-400, indicate 3.2 and 0.6 wt% of acid, respectively (). The lowering in acid content in the calcined catalysts compared to non-calcined catalysts can be ascribed to the decomposition of sulfuric acid to SO3 and H2O above 340°C Citation31. The IR specrta of the catalysts () show a band at 1284 cm−1 due to Si[sbnd]O[sbnd]S bond confirming the presence of bonded acid species on silica support in calcined catalysts.
The morphological features of the catalysts were investigated by scanning electron microscopy (SEM) and particle size distribution by laser particle size analyzer ( and ). Silica support SiO2-1 particles are spherical in shape with uniform morphology and size as compared to SiO2-2. The morphology of particles is retained after the loading of sulfuric acid and further heating the sample at 400°C for 4 hours (). The XRD diffraction patterns of support and catalyst showed no diffraction peaks indicating an amorphous nature of the supports and its retention after acid loading and calcination. The catalyst based on the SiO2-1 support is found to show high specific surface area and porosity as compared to the SiO2-2 support based catalyst (). Energy dispersive X-ray (EDX) analysis of the catalysts was carried out for determining the distributions of acid sites in terms of sulfur species (). The variation in amount of sulfuric acid over the studied silica supports was observed. The catalyst characterization studies indicate that the spherical shape, narrow particle size distribution, high surface area, and high pore volume resulted in the higher amount of acid in the SiO2-1-based catalyst in comparison to the SiO2-2-based system.
Table 2. Surface elemental distributions of catalysts by EDX in wt%.
The silica-supported catalysts were found to catalyze the esterification of myristic acid with isopropyl alcohol to produce isopropyl myristate (). A time-dependent process study was carried out to determine conversion and selectivity at different time intervals. The data () showed a higher rate of reaction in the initial 1 hour resulting in 60% conversion of myristic acid. The conversion of acid to ester using SiO2-1-SO4 and SiO2-2-SO4-based catalysts were found to be 95 and 64%, respectively, () in 6 hours of reaction time. The selectivity is found to be in the range of 98–99% for both the catalysts. The higher conversion for the SiO2-1-based catalyst is attributed to higher acid content, surface area, and pore volume compared with SiO2-2 system. The catalytic activities of the silica-supported sulfuric acid were compared with other heterogeneous catalysts such as clays and acidic alumina. Results indicate () higher performance of silica-supported catalysts as compared to the clay and acidic alumina systems. The reaction product was characterized by GC and NMR data. 1H and 1H decoupled 13C NMR of the product confirmed the synthesis of isopropyl myristate. 1H NMR spectrum of the product showed additional peaks assignable to –COOC3H9 as compared to myristic acid. Characteristic peaks for CH and CH3 of isopropyl group was observed at 5.0 ppm as multiplate and at 1.2 ppm as doublet, respectively. This is substantiated by 1H decoupled 13C NMR spectrum showing peaks attributed to CH3 of isopropyl at 21.7 ppm and CH of isopropyl at 67.1 ppm. The peak due to >C = O is found to be shifted from 180.4 to 173.2 ppm due to the substitution of the –COOH proton by the more electron donating isopropyl group.
Figure 4. Reaction profile of myristic acid with isopropyl alcohol.
Note: Reaction conditions: myristic acid 2 g, catalyst (SiO2-1-SO4) amount 0.2 g, isopropyl alcohol 20 ml, reaction temperature 100±2°C, reaction time 6 hours.
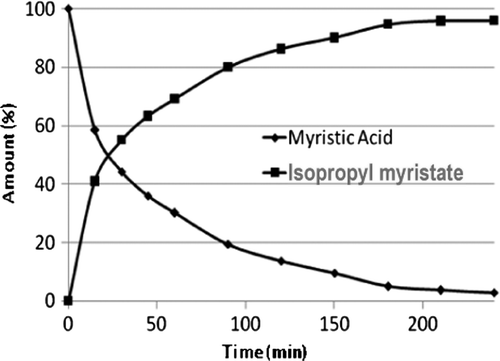
Table 3. Catalytic activities of silica-supported catalysts.
Table 4. Catalytic activities of other heterogeneous catalysts.
Influence of process parameters on catalytic activity
The effects of the process parameters such as solvent to substrate ratio, catalyst concentration, and temperature, were studied for the SiO2-1-SO4 catalyst on conversion and selectivity of the reaction system (). The change in the volume/weight ratio of isopropyl alcohol/myristic acid from 5 to 10 showed an increase in conversion from 88 to 95% with no change in the selectivity. No significant change in conversion and selectivity was observed with further change in ratio to 20. However, the increase in reaction time from 3 to 6 hours at fixed isopropyl alcohol/myristic acid ratio resulted in higher conversions.
Table 5. Effect of process parameters on conversion using SiO4-1-SO4 catalyst.
The effect of reaction temperature on conversion and selectivity is shown in . The conversion is found to be 45% at 60°C. The increase in reaction temperature to 100°C resulted in 95% conversion of myristic acid with selectivity of 99%. The p-toluenesulfonic acid-based esterification is also reported to show higher yield at 130°C Citation32.
The influence of catalyst concentration on the yield of ester was studied at 2.5, 5, and 10 wt% with respect to myristic acid (). The conversion data showed an increasing trend from 69 to 95% with increasing catalyst amount in 3 hours. It is also noted that the conversion can further be increased at same catalyst concentration by increasing the reaction time to 6 hours.
Influence of calcination of catalyst on activity
Catalytic studies were carried out with calcined solid catalyst to understand the performance of the stable acid sites over supported catalyst. Calcined catalysts showed low conversion of 32 and 19% in 6 hours for catalyst having SiO2-1 and SiO2-2 support, respectively (). The increase in reaction time to 24 hours resulted in the increase of conversion to 76 and 42% for SiO2-1-SO4-400 and SiO2-2-SO4-400, respectively. The low performance of calcined catalysts as compared to uncalcined catalysts were due to a smaller numbers of acid sites in calcined catalysts as found by EDX analysis as well as by titration method ( and ). Furthermore, results indicate that the strongly bonded acid sites on the surface of silica show catalytic behavior for esterification reactions.
Influence of recycling of catalyst on activity
The performance of recycled catalyst was studied for the esterification reaction. Conversion of myristic acid to ester with recycled SiO2-1-SO4 catalyst was found to be 23% in 6 hours. An increase in reaction time to 24 hours resulted in a three-fold increase in conversion to 68% (). Results indicate that recycled catalyst showed low activity due to a reduction of acid sites in the recycled catalyst. This was reflected in the compositional analysis of used catalyst showing 3.5 wt% of acid as compared to 60.4% in the fresh catalyst. Similar trends of low activity was also observed from the recycled calcined catalyst system.
Table 6. Catalytic activities of recycled catalysts.
Conversion efficiency of supported catalyst with respect to sulfuric acid
The conversion efficiency of supported catalyst was compared with sulfuric acid (). The conversion data of various catalysts indicates that the 10 wt% amount of supported catalyst SiO2-1-SO4 (where acid contents was 6%) exhibited equivalent activity (98% conversion) to 5 wt% sulfuric acid catalyst. However, the 5 wt% amount of SiO2-1-SO4 (acid content − 3%) also showed 95% conversion. These results indicate the higher activity of the acid in supported catalyst when compared to the homogeneous acid.
Experimental
Materials
SiO2-1 (Silica MS-3050, PQ Corporation, USA), SiO2-2 (Chromatographic grade − 70–230 mesh Silica gel, Labort Chemicals), sulfuric acid (Labort Chemicals), acidic alumina (Sigma-Aldrich), Bentonite clay (Natural resources of Rajasthan, India), myristic acid (CDH, India), and isopropyl alcohol (Labort Chemicals) were used for catalyst synthesis and chemical transformation reactions. All the chemicals were used as such without further purification.
Catalyst synthesis
-
About 100 ml of 70% concentrated sulfuric acid was added to 5 g of silica. The reaction mixture was stirred at ambient conditions for 4 hours. The mixture was filtered to separate the solid product. The solid product was dried at 80°C for 24 hours under vacuum.
-
Clay catalysts were synthesized by a previously reported method Citation33. A 5 g of Bentonite clay was taken for the reaction with sulfuric acid of varied concentration from 1 to 8N at 80°C for 2 hours. The acid-treated clay was washed thoroughly with distilled water until there was an absence of
as tested by a BaCl2 solution followed by drying in an oven at 110°C.
Catalyst characterization
The acidity of catalysts was determined by volumetric analysis using 0.1 N NaOH solution as the titrant and phenolphthalein as an indicator. FT-IR spectra of solid products in Nujol Mull were recorded with a KBr window using a Spectrum GX Fourier Transform infrared spectrometer (Perkin–Elmer) with a resolution of 2 cm−1 in the range of 4000–400 cm−1. Wide-angle X-ray diffraction (WAXD) were carried out using a Bruker D8-Advance diffractometer in the reflection mode at 40 kV and 40 mA with a Cu Kα1 (λ = 1.5405 Å) as a radiation source in 2κ range from 2 to 70. SEM images were obtained with FEI Inspect S SEM operated at an accelerated voltage of 10–20 kV with a working distance of 10 mm. Surface elemental composition of catalysts were obtained using an Oxford INCA EDX analyzer attached with SEM at 20 kV. Particle size distributions of the catalysts were obtained by dispersing the particles in mineral oil and determined using a Cilas (Model 1180) Laser particle size analyzer (). The surface area and pore volume were determined by Brunauer Emmett Teller (BET) method with nitrogen gas at liquid nitrogen temperature using a Thermoquest – CE Instruments (Model Sorptomatic 1990) surface area analyzer.
Catalytic studies
Myristic acid and isopropyl alcohol were taken in a glass reactor followed by the addition of catalyst with continued stirring at different temperatures. Samples were taken out from the reaction mixture at regular time intervals for quantitative analysis. Quantification of the reactants and products were carried out by gas chromatography (Agilent Model 6980), equipped with HP-1 capillary column (30 m long and 0.32 mm internal diameter). Reaction mixtures were eluted from 50 to 200°C at a rate of 10°C using nitrogen as the carrier gas. The product was confirmed by the retention time of pure compounds prior to quantification.
The conversion was calculated as follows:
The reaction product was also confirmed by 1H NMR at 400 MHz and 1H decoupled 13C NMR at 100 MHz using a Bruker Avance 400 FT-NMR spectrometer with CDCl3 as the solvent in the range of 0–12 ppm and 0–200 ppm, respectively.
Conclusions
Morphological silica-supported acid catalysts were synthesized by the reaction of acid with silica support. The morphology, surface area, and the porosity of the support were found to be critical characteristics for high incorporation of acid. Weak, as well as strongly bonded, acid species were observed over the surface of support. Morphological silica-supported acid catalysts showed better performance for esterification of myristic acid as compared to non-morphological, homogeneous sulfuric acid, and other heterogeneous catalysts. The conversion of myristic acid to the corresponding ester was highly influenced by the change of process parameters as compared to selectivity. These results demonstrated that silica-supported acid catalysts fulfill green synthetic aspects such as minimization of waste, less hazardous chemical synthesis, safer reaction conditions, and higher energy efficiency for the esterification process.
References
- Tanabe , K. and Holderich , W.F. 1999 . Appl. Catal. A Gen. , 181 : 399 – 434 .
- Cornils , B. ; Herrmann , W.A. ; Muhler , M. ; Wong , C.H. Catalysis from A to Z: A Concise Encyclopedia ; Weinheim : Wiley-VCH , 2007 .
- Furniss , B.S. , Hannaford , A.J. , Smith , P.W.G. and Tatchell , A.R. 1989 . Vogel's Textbook of Practical Organic Chemistry , 5th ed , New York : Longman Scientific & Technical .
- Yadav , M.K. and Jasra , R.V. 2006 . Catal. Commun. , 7 : 889 – 895 .
- Price , P.M. , Clark , J.H. and Macquarrie , D.J. 2000 . J. Chem. Soc., Dalton Trans. , 2 : 101 – 110 .
- Clark , J.H. , Butterworth , A.J. , Tavener , S.J. , Teasdale , A.J. , Barlow , S.J. , Bastock , T.W. and Martin , K. 1997 . J. Chem. Technol. Biotechnol. , 68 : 367 – 376 .
- Barlow , S.J. , Bastock , T.W. , Clark , J.H. and Cullen , S.R. 1995 . Tetra. Lett. , 34 : 3339 – 3342 .
- Ciapetta , F.G. US Patent 2430803, November 11 , 1947 .
- Joly , J.F. ; Marcilly , C. ; Benazzi , E. US Patent 5420093, May 30 , 1969 .
- Joly , J.F. ; Marcilly , C. ; Benazzi , E. US Patent 5336833, August 9 , 1994 .
- Yadav , G.D. and Goel , P.K. 2000 . Green Chem. , 2 : 71 – 72 .
- Steinigeweg , S. and Gmehling , J. 2003 . Ind. Eng. Chem. Res. , 42 : 3612 – 3619 .
- Chaussee , J.G. US Patent 4478853, October 23 , 1984 .
- Parab , P.V. US Patent 5326566, July 5 , 1994 .
- Nakagawa , A. ; Miyata , S. ; Kubota , Y. US Patent 5322685, June 21 , 1994 .
- Kimura , C. EP Patent 1852106, November 7 , 2007 .
- Jayaraman , S.C. , Ramachandran , C. and Weiner , N. 1996 . J. Pharma. Sci. , 85 ( 10 ) : 1082 – 1084 .
- Agapiou , A.K. ; Kuo , C.I. ; Glowczwski , D.M. Ackerman , S.K. US Patent 7354880, April 8 , 2008 .
- Chen , L. ; Campbell , R.E. Jr. US Patent Appl. 20070027275, February 1 , 2007 .
- Erguen , N. Panning , P. US patent 7256301, August 14 , 2007 .
- Wilson , K. and Clark , J.H. 2000 . Pure Appl. Chem. , 72 : 1313 – 1319 .
- Juan , J.C. , Zhang , J. and Yarmo , M.A. 2007 . Appl. Catal. A Gen. , 332 : 209 – 215 .
- Gupta , V.K. , Satish , S. and Bhardwaj , I.S. 1993 . Die Angew Makro Chem. , 213 : 113 – 125 .
- Gupta , V.K. , Satish , S. and Bhardwaj , I.S. 1994 . Die Angew Makro Chem. , 220 : 39 – 47 .
- Gupta , V.K. , Satish , S. and Bhardwaj , I.S. 1994 . JMS Pure App. Chem. , A31 ( 4 ) : 451 – 463 .
- Gupta , V.K. , Satish , S. and Bhardwaj , I.S. 1995 . Euro. Poly. J. , 31 ( 2 ) : 145 – 148 .
- Kaushik , V.K. , Gupta , V.K. , Patil , H. and Naik , D.G. 2008 . Cat. Lett. , 121 : 58 – 62 .
- Lenza , R.F.S. and Vasconcelos , W.L. 2001 . Mat. Res. , 4 ( 3 ) : 189 – 194 .
- Givan , A. , Loewenschuss , A. and Nielsen , C.L. 1999 . Phys. Chem. Chem. Phy. , 1 : 37 – 43 .
- Budavari , S. The Merck Index , 11th ed. New Jersey : Merck & Co. Inc , 1989 , 1417 – 1418 .
- Wang , R. , Zhang , D. and Liu , C. 2005 . Chem. Phys. Lett. , 1404 : 237 – 243 .
- Blanco , M. , Castillo , M. and Beneyto , R. 2007 . Talanta , 72 : 519 – 525 .
- Yadav , M.K. , Chudasama , C.D. and Jasra , R.V. 2004 . J. Mol. Catal. A Chem. , 216 : 51 – 59 .