Abstract
Synthesis of various substituted cyclo[b]indoles has been accomplished by using a combined catalytic system of phosphated zirconia (P-Zr) and Bi(NO3)3.5H2O. However, the use of Bi(NO3)3.5H2O or P-Zr separately as heterogeneous acid catalysts generated 2,2'-diindolylpropanes (DIPs) as a major product. The reactions were carried out under mild reaction conditions and required less time. The catalysts applied for the reaction were reusable. Substituted cyclo[b]indoles and several 2,2'-diindolylpropanes are synthesized by using various indoles and ketones.
Introduction
The synthesis of indole and substituted indoles has received much attention because of their significant biological activities. Indole derivatives possessing various physiological properties are obtained from a variety of natural plants. The diindolylpropanes (DIPs) and substituted cyclopenta[b]indoles (CPIs) constitute an important class of compounds Citation1–3, which have utile pharmacological properties Citation4–12. The applications of DIPs as anticancer are well-known and they are also used for the synthesis of various dyes Citation4–12. There is no dearth in study of reactions between indoles and ketones to produce DIPs, but the study is only limited to acetone and cyclic ketones. Moreover, it lacks a detailed study of synthesis of different DIPs by using various substituted indoles Citation10–12. The applications of bis-indole alkaloid, yuehchukene, which is racemic are well-known Citation13. They possess strong antiimplantation activity in rats Citation14 as well as in mice Citation15 and moderate activity in Guinea pigs Citation16 . The CPIs synthesized by using the above protocol have structures analogous to yuehchukene and thus their synthesis is of immense importance. There is only a cursory knowledge of the reactions between ketones and substituted indoles to synthesize CPIs Citation17–20. The first ever structure elucidation and synthesis of substituted CPIs by reacting acetone and indoles in the presence of ethanolic hydrochloric acid as a reagent were carried out by Bergman et al. in 1989 Citation17–20. Chatterjee et al. used BF3.Ethereate for the synthesis of similar types of compounds in 1980 Citation17–20. Similarly, CPIs were also synthesized by using methanolic HCl as a reagent by Black et al. in 1991 Citation17–20. The reagents used in the above process are hazardous as they are corrosive and cause handling problems in the industry on a larger scale. Moreover, they cause disposal problems and their separation from reaction mixture is difficult. The time required for the completion of reaction is more and the temperature at which the reaction is carried out is difficult to attain. Therefore, none of these methods are environmentally friendly Citation17–23 and a need to synthesize CPIs under greener and benign reaction conditions is necessary. The reactions carried in the above processes are at a lower temperature, requiring lesser reaction time. The synthesis of CPIs is carried out in presence of a solid acid catalyst which could be easily separated from the reaction mixture and does not have any handling problems making it a greener and environmentally friendly process.
Results and discussions
In our quest to synthesize nitroindole, in presence of metal nitrate as a nitrating agent, we barged upon a product which was totally unexpected. A detailed analysis of the new compound synthesized found it to be substituted CPI Citation1 (). The method is highly efficient and free from the aforesaid drawbacks. Phosphated zirconia (P-Zr) Citation21–23 and bismuth nitrate penta hydrate (BNPH) Citation24, Citation25 are tested as a solid acid catalyst for this transformation. A brief account of the work and its main findings as well as the advantages over the extant synthetic routes is discussed in this communication. P-Zr was prepared by precipitation method Citation21–23, whereas BNPH was procured from M/s S.D. Fine Chemicals, India and was used in its pristine form. P-Zr was characterized by FT-IR and XRD techniques Citation21–23. Ketones and indoles used for the reactions were procured from M/s S.D. Fine Chemicals, India and were used without further purification.
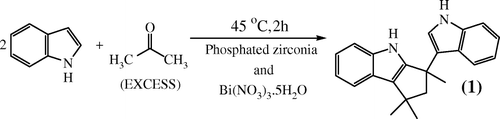
The synthesis, 3-(1H-indol-3-yl)-1,1,3-trimethyl-1,2,3,4-tettrahydro-cyclopenta[b]indole (), suggests that the role of the acetone in the reaction is much more than that of a solvent. Instead, acetone interferes in the reaction and completely alters the final product. Acetone acts as an electrophile which is attacked by electron-rich indole acting as a nucleophile. BNPH was originally meant to act as a nitrate donor, but the synthesis of CPI discarded the role of BNPH as reactant. It is assumed that BNPH interacts with P-Zr in situ and thereby increasing the acidic characteristic of the combined catalytic system BNPH/P-Zr catalyst. It could be hypothesized that the increase in acidity favors the formation of alkene substituted indole Citation2 () and results in the synthesis of cyclic[b]indoles.
Figure 1. Mechanism for the synthesis of 3-(1H-indol-3-yl)-1,1,3-trimethyl-1,2,3,4-tettrahydro-cyclopenta[b]indole.
![Figure 1. Mechanism for the synthesis of 3-(1H-indol-3-yl)-1,1,3-trimethyl-1,2,3,4-tettrahydro-cyclopenta[b]indole.](/cms/asset/238c96f9-e2e3-4dd5-a060-5b8460e10c1c/tgcl_a_515619_o_f0001g.gif)
In absence of either P-Zr or BNPH, the net acidity of the catalyst decreases, which results in the decrease in the synthesis of Citation2 (). This could result in the increase in concentration of the unreacted indole as compared to the alkene substituted indole and favor the synthesis of DIPs. The exact ratio of the amount of BNPH and P-Zr was studied and the optimum ratio of P-Zr/BNPH for a batch of 1 mmol of indole was finalized at 0.022 g of P-Zr to 0.4 mmol of BNPH with respect to indole. A series of nitrates of Fe, Cu, Al, and Co were tried instead of BNPH, but BNPH was retained for the reaction as it gave best results. The reaction temperature of 45°C was enough to give a good yield of product in a span of 2 h. Reusability of catalyst was also explored. The catalyst was separated from the reaction mixture by filtration, washed with ethanol, and dried at 60°C for 1 h. The product yield was 85% the procedure is repeated for another cycle and gave more or less same product yield. The synthesis, 3-(1H-indol-3-yl)-1,1,3-trimethyl-1,2,3,4-tettrahydro-cyclopenta[b]indole, involves the addition of an acetone molecule to the indole moiety by a simple acid catalyzed condensation reaction to gives an unsaturated alkene substituted indole (A). The two isomeric forms of the unsaturated alkene substituted indole (A) moieties undergo addition reaction, which further undergo an intra molecular cyclization reaction to produce the desired compound (B) Citation13 ().
Reactions when carried out by using 2-methylindole under similar conditions to that of indole, produce a slightly different moiety than that of indole, 2-methylindoles produce 4,4,9a-trimethyl-2-(2-methyl-1H-indol-3-yl)-4,4a,9,9a-tettrahydro-3H-carbazole instead of CPIs Citation13 ().
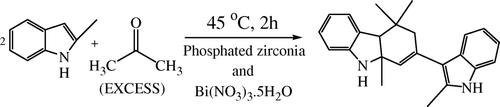
The mechanism followed for the synthesis of substituted carbazoles is similar to that of the synthesis of substituted CPIs. 2-Methylindole reacts with acetone to produce alkene substituted 2-methylindole (A’). Two molecules of (A’) react with each other to form an adduct that further cyclizes to form substituted carbazoles. But due to the presence of a methyl group in case of (B), the cyclization process is hindered. Due to this hindrance, the terminal carbon atom gets involved in the ring formation process instead of the quaternary carbon atom thereby giving rise to a six member ring rather than a five member ring Citation13 (, ).
Figure 2. Mechanism for the synthesis of 4,4,9a-trimethyl-2-(2-methyl-1H-indol-3-yl)-4,4a,9,9a-tettrahydro-3H-carbazole.
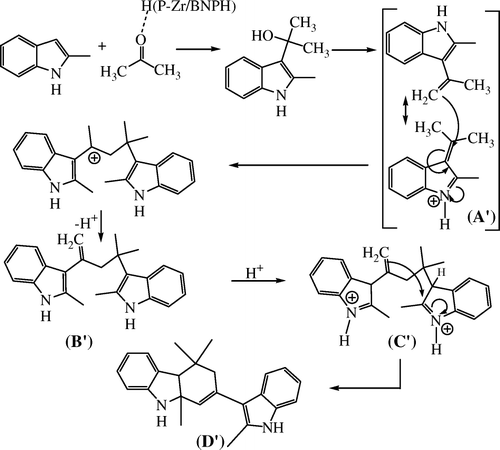
Table 1. Synthesis of substituted cyclo[b]indoles.a
The reactions when carried out by using BNPH as the catalyst separately gave a mild and expedient synthesis of symmetrical DIPs (). BNPH Citation17–20 and P-Zr Citation16 are used as solid acid catalysts for various organic transformations, therefore, their application for the synthesis of DIPs has generated interest and prompted for this work. The reaction was carried out in acetone, which plays a dual role, as a reactant and as a solvent. Addition of any other solvent in the reaction decreased the yield of the product. Maximum yield of the product was obtained at 45°C, which was considered as the optimum temperature. The yield of the product decreased with decrease in temperature, whereas, increase in temperature did not have any positive effect on the yield. A 3.5–4 h of reaction time was adequate to carry out the reaction to give an excellent yield of the product. The reaction time and temperature required for the synthesis of DIAs were reduced as compared to the reported method Citation4–9. For a reaction of 1 mmol of indole 10 mol%, i.e. 0.10 mmol of BNPH was required for the completion of the reaction. Increase in the amount of BNPH from 10 to 40 mol% did not have any significant effect on the yield of the product and therefore 10 mol% was considered as the optimum catalyst quantity. The reaction was carried out with various metal nitrates () and BNPH was selected as the preeminent metal nitrates. Encouraged by these initial results we went on to investigate the generality of this procedure for P-Zr. A 25 wt% of P-Zr was used as a catalyst to give moderate yields of DIAs after 24 h reaction time at 45°C for various indoles.
Table 2. Synthesis of 2,2'-diindolylpropanes using a range of indoles and ketones.a
Indole molecule first reacts with the acetone molecule to give an addition reaction which results in the formation of alcohol. Loss of water molecule from the alcohol gives an alkene substituted indole. Addition of a second indole molecule to the unsaturation gives the formation of 2,2'-diindolylpropanes ().
Reactions of indoles and substituted indoles with ketones were carried out under optimized conditions. indicates various products formed in these reactions. The yield of the reaction in a span of 3.5–4 h was almost same for different indoles. The yield was increased for methyl substituted indoles at 2-position and N-position (, Entries 2 and 3) which could be attributed to the activating effect of the ring due to +I effect of the methyl group. 3-Methylindole when reacted with acetone did not give any product (, Entry 6). The third position is the most reactive position in case of indole, blocking the third position stops the addition of an acetone molecule to indole, thereby inhibiting the reaction. We can thus infer that the reaction on indole takes place at the third positions in case of indoles. Reactions with haloindoles show a slight decrease in the yield of product (, Entries 4 and 5). This could be due to the –I effect of the halo group which decreases the electron density on the ring, thereby deactivating the third position of indole.
Conclusions
The synthesis of cyclo[b]indoles and less-known 2,2'-diindolylpropanes was carried out efficiently. The reactions were carried out at benign reaction temperatures and required less time for completion. The catalysts used for the synthesis of 2,2'-diindolylpropanes were Bi(NO3)3.5H2O and P-Zr separately. Cyclo[b]indoles were synthesized using a mixture of Bi(NO3)3.5H2O and P-Zr as solid acid catalysts. In conclusion, the catalytic system used for both the reactions was established as cheap, reusable, convenient, and efficient.
Procedure for the synthesis of 2,2'-diindolylpropanes (DIP) and cyclo[b]indoles
Synthesis of cyclo[b]indoles
A mixture of indole 0.117 g (1 mmol), 0.021 g (20 wt%) of P-Zr, and 0.160 g (0.4 mmol) of BNPH in 1 ml acetone was stirred at 45°C for 2 h. The reaction was monitored using TLC (10% ethyl acetate/pet ether). After completion of the reaction, ethyl acetate (3×5 ml) was added to the reaction mixture and the catalyst was separated by filtration process. The combined organic layer was washed with water and dried over anhydrous sodium sulfate. The organic layer was concentrated in vacuum evaporator and purified by column chromatography (ethyl acetate:petroleum ether) to obtain the pure product. The product was characterized using 1H NMR, 13C NMR, DEPT 60, FT-IR, and LCMS techniques.
Synthesis of 2,2'-diindolylpropanes (DIP)
A mixture of 0.234 g of indole (2 mmol) and 0.117 g of BNPH (0.2 mmol) in 1 ml of acetone was stirred at 45°C for 4 h. After completion of the reaction (TLC), ethyl acetate (3×5 ml) was added to the reaction mixture and the catalyst was separated by filtration process. The combined organic layer was washed with water and dried over anhydrous sodium sulfate. The organic layer was concentrated in vacuum evaporator and purified by crystallization process (ethyl acetate:petroleum ether) to obtain the pure product. The products were characterized using 1H NMR, FT-IR, and LCMS techniques.
Product characterization: spectroscopic data of the reaction products
Entry 1 ()
1H NMR (350 MHz, CDCl3): δ = 1.64 (s, 6H, 2CH3), 1.90 (s, 3H, CH), 2.56 (d, 1H, CH), 3.09 (d, 1H, CH), 6.94–7.93 (Ar–11H); 13C NMR (350 MHz, CDCl3): δ = 123–149 PPM (q, 7C-Ar), 112–123 PPM (t, 9C-Aromatic), 63 PPM (s, 1C), 40–44 PPM (q, 2C-non Ar), 29–32 PPM (p, 3C); IR (KBr): 3061 cm−1, 1465 cm−1, 749 cm−1, 1529 cm−1 and 3410 cm−1; DEPT 135: CH3– positive peaks, 29–32 PPM (p, 3C), CH2– negative peaks, –63 PPM (s, 1C), CH– positive peaks, 112–123 PPM (t, 9C-Aromatic), quaternary carbon not observed on DEPT 135; MS/MS Analysis (positive ESI mode): calculated for (M–1) = 314, found (M + 1) = 315.02.; (negative ESI mode): found (M + 1) = 313.14.
Entry 2 ()
1H NMR (350 MHz, CDCl3): δ = 0.82 (s, 3H, 2CH3), 1.19–1.34 (2s, 6H, CH3), 2.09–2.25 (2s, 2H, CH), 2.44 (s, 3H, CH3), 2.78 (s, 1H, CH), 3.45 (s, 1H, NH), 5.77 (s, 1H, CH), 6.66–7.82 (Ar–9H); IR (KBr): 3031 cm−1, 1455 cm−1, 744 cm−1, 1532 cm−1 and 3410 cm−1; MS/MS Analysis (positive ESI mode): calculated for (M–1) = 343.24, found (M) = 343.27.
Entry 1 ()
1H NMR (350 MHz, CDCl3): δ = 1.84 (s, 6H, 2CH3), 7.09 (s, 2H, CH), 7.16 (s, 4H, CH), 7.43 (s, 2H, CH), 8.00 (s, 2H, NH); IR (KBr): 3046 cm−1, 2961 cm−1, 1455 cm−1, 744 cm−1, 1322 cm−1, 822 cm−1, and 1541 cm−1; MS/MS Analysis (negative ESI mode): calculated for (M–1) = 274.15, found (M–1) = 273.13.
Entry 2 ()
1H NMR (350 MHz, CDCl3): δ = 1.90 (s, 6H, 2CH3), 2.47 (s, 6H, CH3), 6.79 (s, 4H, CH), 6.91 (s, 2H, CH), 7.23 (s, 2H, CH), and 7.97(s, 2H, NH); IR (KBr): 3039 cm−1, 2954 cm−1, 1469 cm−1, 744 cm−1, and 1330 cm−1; MS/MS Analysis (positive ESI mode): calculated for (M–1) = 302.18, found (M) = 302.13.
Entry 3 ()
1H NMR (350 MHz, CDCl3): δ = 1.89 (s, 6H, 2CH3), 3.72 (s, 6H, CH3), 6.87 (s, 5H, CH), 7.11 (s, 2H, CH), 7.23 (s, 1H, CH), and 7.43 (s, 2H, NH); IR (KBr): 2981 cm−1, 1469 cm−1, 740 cm−1, 1325 cm−1, and 1541 cm−1; MS/MS Analysis (positive ESI mode): calculated for (M–1) = 302.18, found (M + 23), Adduct with Na = 325.27.
Entry 4 ()
1H NMR (350 MHz, CDCl3): δ = 1.85 (s, 6H, 2CH3), 7.10 (s, 3H, CH), 7.16 (s, 3H, CH), 7.41 (s, 2H, CH), 8.00 (s, 2H, NH); IR (KBr): 3041 cm−1, 2965 cm−1, 1462 cm−1, 794 cm−1, 1333 cm−1, 822 cm−1, and 1558 cm−1; MS/MS Analysis (negative ESI mode): calculated for (M–1) = 343.25, found (M–1) = 341.47.
Entry 5 ()
1H NMR (350 MHz, CDCl3): δ = 1.84 (s, 6H, 2CH3), 6.99 (s, 2H, CH), 7.23 (s, 4H, CH), 7.39 (s, 2H, CH), 8.00 (s, 2H, NH); IR (KBr): 2964 cm−1, 1469 cm−1, 795 cm−1, 1338 cm−1, and 1559 cm−1.
References
- Chakrabarty , M. ; Ghosh , N. ; Basaka , R. ; Hari Gayab , Y. Tetrahed. Lett . 2002 , 43 , 4075 4078 .
- Magesh , C.J. ; Nagarajan , R. ; Karthik , M. ; Perumal , P.T. Appl. Catal. A: Gen . 2004 , 266 , 1 10 .
- Khalafi-Nezhad , A. ; Parhami , A. ; Zare , A. ; Zare , A.R.M. ; Hasaninejad , A. ; Panahi , F. Synthesis 2008 , 617 621 .
- McDougal , A. ; Gupta , M.S. ; Morrow , D. ; Ramamoorthy , K. ; Lee , J.-E. ; Safe , S.H. Breast Cancer Res. Treat . 2001 , 66 , 147 157 .
- Mi , X. ; Luo , S. ; Hea , J. ; Chenga , J.P. Tetrahed. Lett . 2004 , 45 , 4567 4570 .
- Reddy , B.M. ; Sreekanth , P.M. ; Lakshmanan , P. J. Mol. Catal. A: Chem . 2005 , 237 , 93 100 .
- Hosseini-Sarvari , M. Synth. Commun . 2008 , 237 832 840
- Hagiwara , H. ; Sekifuji , M. ; Hoshi , T. ; Qiao , K. ; Yokoyama , C. Synlett 2007 , 1320 1322 .
- Zhang , Z.-H. ; Yin , L. ; Wang , Y.-M. Synthesis 2005 , 1949 1954 .
- Bandgar , B.P. ; Shaikh , K.A. Tetrahed. Lett . 2003 , 44 1959 1961
- Li , J.T. ; Dai , H.G. ; Xu , W.Z. ; Li , T.S. Ultra. Sonochem . 2006 , 13 , 24 27 .
- Sharma , G.V.M. ; Reddy , J.J. ; Lakshmi , P.S. ; Krishna , P.R. Tetrahed. Lett . 2004 , 45 , 7729 7732 .
- Fgdg Kong , Y.-C. ; Cheng , K.-F. ; Cambie , R.C. ; Waterman , P.G. Chem. Commun . 1985 , 2 , 47 48 .
- Kong , Y.-C. ; Ng , K.-H. ; Wat , K.-H. ; Wong , A. ; Saxena , I.F. ; Cheng , K.-F. ; But , P.P.-H. ; Chang , H.-T. Planta Med . 1985 , 44 , 304 307 .
- Wang , N.-G. ; Guan , M.-Z. ; Lei , H.-P. Ydoxue Xuebao 1990 , 25 , 85 ; Wang, N.-G.; Guan, M.-Z.; Lei, H.-P. Chem. Abstr. 1990, 113, 670v .
- Hammarstrom , M. , Venemalm , L. , Bergman , J. and Eneroth , P. 1990 . Am. J. Chin . Med , 18 : 1 – 4 .
- Bergman , J. , Norrby , P.-O. , Tilstam , U. and Venemalm , L. 1989 . Tetrahedron , 45 : 5549 – 5564 .
- Bergman , J. and Venemalm , L. 1992 . Tetrahedron , 48 : 759 – 769 .
- Black , D.C. , Craig , D.C. and Kumar , N. 1991 . Tetrahed . Lett , 32 : 1587 – 1590 .
- Chattterjee , A. ; Manna , S. ; Banerji , J. ; Pascard , C. ; Prange , T. ; Shoolery , J.N. J. Chem. Soc. Perkin Trans. 1: Org. Bioorg. Chem . 1980 , 33 , 553 555 .
- Yu , L.B. ; Chen , D.P. ; Wang , P. Tetrahed. lett . 1996 , 37 , 4467 4470 .
- Feng , X.L. ; Guan , C.J. ; Zhao , C.X. Synth. Commun . 2004 , 487 492 .
- Han , J.W. ; Tian , H. ; Sheng , J. ; Fan , Z.Y. ; Tang , X.P. Synlett 2005 , 337 339 .
- Nadkarni , S.V. ; Gawande , M.B. ; Jayaram , R.V. ; Nagarkar , J.M. Catal. Commum . 2008 , 1728 1733 .
- Alexander , V.M. ; Bhat , R.P. ; Samant , S.D. Tetrahed. Lett . 2005 , 46 , 6957 6959 .