Abstract
A highly stable ternary complex of copper(II) with 5-(4-nitrophenylazo) salicylic acid (NPAS) and 2,2′-Dipyridyl (Dp) in ethanol medium at room temperature gave a maximum absorption at 520 nm with a molar absorptivity 2.60×104 L mol-1 cm-1. This method was characterized by safety to the environment as there are no uses of hazardous solvents, which may harm our environment. The linear range for copper determination was 2.2–6.3 mg L-1. The method was sensitive, accurate, and tolerant to many foreign ions. All the reagents were stable under the working conditions. Moreover, the method was easy to perform for the determination of copper in pharmaceutical, biological, and water samples.
Introduction
Although considered as a highly toxic element in drinking water at high concentrations, copper is an essential element for human life and its deficiency causes ischemic heart disease, anemia, abnormal wool growth, and bone disorders, although considered as a highly toxic element in drinking water at high concentrations. Thus, the determination of trace amounts of Cu(II) is becoming increasingly important.
In recently reported references, Cu(II) was determined in different samples by using graphite furnace atomic absorption spectrometry (GF-AAS) Citation1 Citation2, inductively coupled plasma optical emission spectrometry (ICP-OES) Citation3–5, and high performance liquid chromatography Citation6 Citation7. Anodic stripping voltammetry Citation8 Citation9 has also been used for the determination of copper with high sensitivity and selectivity, but suffer from more or less time-consuming procedures and complicated instrumentation.
Some of the previous methods for spectrophotometric determination (1973–2002) of Cu(II) have some disadvantages such as time-consuming, labor intensive, and the use of large amounts of organic solvents that can increase the environmental pollution (). Some of these previous methods use hazard solvents such as carbon tetrachloride.
Table 1. Comparison of previous methods for spectrophotometric determination of Cu(II).
Carbon tetrachloride is one of the most potent hepatotoxins (toxic to liver), and is widely used in scientific research to evaluate hepatoprotective agents. Exposure to high concentrations of carbon tetrachloride (even vapor) can affect the central nervous system, degenerate the liver, and may result (after prolonged exposure) in coma or even death. Chronic exposure to carbon tetrachloride can damage the liver, kidney, and cause cancer Citation10–15.
In 2008, a study of common cleaning products found the presence of carbon tetrachloride in very high concentrations (up to 101 mg m-3) because of manufacturer's mixing of surfactants or soap with sodium hypochlorite (bleach). Carbon tetrachloride is also both ozone depleting and a greenhouse gas. However, since 1992 its atmospheric concentrations have been in decline for the reasons described above Citation16.
For polyvinyl chloride, the State of California is currently considering a bill that would ban the use of PVC in consumer packaging due to the threats it poses to human and environmental health and its effect on the recycling stream. Specifically, the language of the bill analysis stipulates that EPA has listed PVC as a carcinogen. Postconsumer PVC is not typically recycled due to the prohibitive cost of regrinding and decompounding the resin compared to the cost of virgin (unrecycled) resin. The environmentalist group Greenpeace has advocated the global phase-out of PVC because they claimed that dioxin is produced as a by-product of vinyl chloride manufacture and from incineration of waste PVC in domestic garbage Citation17–20.
PVC produces HCl upon combustion almost quantitatively related to its chlorine content. Extensive studies in Europe indicated that the chlorine found in emitted dioxins was not derived from HCl in the flue gases. Instead, most dioxins arise in the condensed solid phase by the reaction of inorganic chlorides with graphitic structures in char-containing ash particles. Copper acts as a catalyst for these reactions Citation21.
For chloroform, chloroform vapors depress the central nervous system. It is immediately dangerous to life and health at approximately 500 mg L-1, according to the US National Institute for Occupational Safety and Health; breathing about 900 mg L-1 for a short time can cause dizziness, fatigue, and headaches. Chronic chloroform exposure can damage the liver (where chloroform is metabolized to phosgene), kidneys, and some people develop sores when the skin is immersed in chloroform. Animal studies have shown that miscarriages occur in rats and mice that have breathed air containing 30–300 mg L-1 of chloroform during pregnancy. Chloroform once appeared in toothpastes, cough syrups, ointments, and other pharmaceuticals, but it has been banned as a consumer product in the United States since 1976 Citation20.
Some of the new spectrophotometric methods for determination of copper(II) from 2003 are present. Kendüzler and Türker proposed a sensitive method for atomic absorption spectrophotometric determination of trace copper, but they used potassium cyanide in the procedure, which is highly toxic and poisonous Citation21. Shinsuke Ohno et al. proposed a method for spectrophotometric determination of trace copper but they used expensive reagents Citation22.
B.K. Reedy proposed another method for spectorophotometric determination of copper but with a narrow range of determination and low molar absorptivity than our method 1.6×104 mol-1 cm-1L Citation23. Pinto et al. used the flow system, which has high RSD (2.11%) with more steps in the procedure for optimization Citation24. Shamsa and Barazandeh-Tehrani's method has a high detection limit of 13 ng L–1 with using chloroform (hazard solvent) Citation25. Massoud's method has low sensitivity for reaction with copper, as it has low molar absorptivity 9.3×103 mol-1 cm-1L Citation26. Fu and Yuan and Yuan et al.'s methods were characterized by their simplicity and high sensitivity toward copper(II) but they were applied only with a maximum concentration of copper equal to 0.6 and 1.02 mg L-1, respectively Citation27 Citation28. Reddy et al.'s method has low molar absorptivity that equals to 0.847×104 mol-1 cm-1L Citation29. Adriana do Nascimento Rocha et al.'s method has poor sensitivity toward copper with molar absorptivity of 0.65×104 mol-1 cm-1L Citation30.
As reported by Mitić et al.'s paper Citation31, the Reddy method is still attractive from an analytical point of view since it is cheap, sensitive, and simple to implement in laboratories using spectrophotometric techniques; but with reference to the Sigma Company, the reagents in Reddy et al.'s method cost about US$64.00 (€48) for two grams, while the reagents in the present method cost about US$5.00 (€4) for two grams, this is besides the high sensitivity and selectivity of this method Citation29. In this study we hoped to find a more clean spectrophotometric method for copper(II) than the previous methods which were expensive and more dangerous to our environment due to more dangerous waste products. We used NPAS and Dp as two ligands that made strong bonds with Cu(II), as NPAS has two groups (–OH, –COOH) and (–N=) in Dp, which led to a chelate effect around the Cu(II) ion and produced a high stability complex. The purpose of using two ligands is to form a ternary complex that is more stable and selectable toward copper than using NPAS alone.
Results and discussion
Acid-base properties of NPAS
The absorption spectra of 0.5×10-4 mol L-1 of the reagent in 50% ethanol with various concentrations of HClO4 and NaOH were recorded as the dependence A=f(λ) for various pH. The UV-visible spectra of NPAS in all mixtures investigated, display four absorption bands within the pH range 1.4 to 12.5 – the maximum absorption of these bands located at 365, 380, 390, and 520 nm. The different acid-base equilibria existing in the solution of NPAS may be represented by the following scheme Citation33:
Absorption spectra and optimum pH
The solution spectra of NPAS exhibited a main absorption band at λ=390 nm in the pH range of 6.0 and 9.3. The spectra of Cu(II)-NPAS (1:1) complex with the reagent blank as reference were characterized by an absorption at λmax 445 nm in the pH 4.0–6.3; a similar behavior was observed for the spectrum of the Cu(II)–Dp complex; neither Dp nor its 1:1 complex with Cu(II) has a measurable absorbance above 250 nm. The effect of pH in the range of 5.0 and 10.3 on the spectrum of solutions containing equimolar concentrations of the NPAS, Dp and Cu(II) against a reagent blank containing the same concentrations of the two ligands, showed an absorption band at λmax 525 nm at pH 7.3 (, ). This band was unambiguously due to the formation of the mixed ligands complex of Cu(II) with NPAS and Dp. The solution spectra of the ternary system in a 1:1:1 molar ratio and the spectra of Cu(II) complex with NPAS and Dp in 50% (v/v) ethanol with reagent blank as reference are given in .
Figure 1. Absorption spectra for NPAS–Cu(II)–Dp complexes, CNPAS=CDp=CM=5×10-5 mol L-1, I=0.1 mol L-1 NaClO4, 50% ethanol, pH; (1) 5.0, (2) 5.8, (3) 6.1, (4) 6.6, (5) 7.0, (6) 7.3, (7) 8.5, (8) 9.2, (9) 9.5.
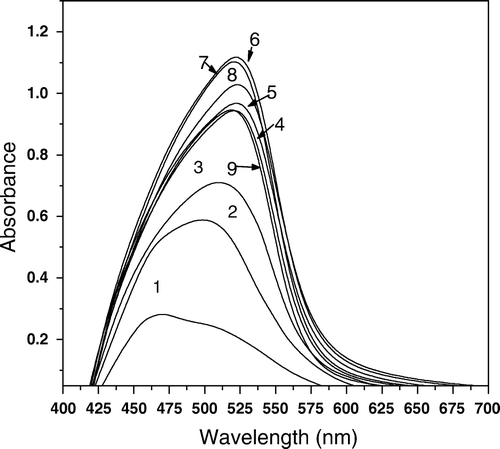
Composition of the Cu(II) ternary complex
Job's method Citation34 was applied to establish the composition of the ternary Cu(II) NPAS and DP complex; the molar fractions of two of the components were varied continuously, keeping the third component in a large excess for all solutions in the series (Figures ). The obtained results indicated that the overall Cu(II)–NPAS–Dp complex had a 1:1:1 composition at the pH of the study. The molar ratio method was also determined the stoichiometry of the ternary complex Citation34 Citation35.
Figure 4. Absorption spectra for NPAS–Cu(II)–Dp complexes, CNPAS=12.5×10-5 M, Ctotal=15×10-5 mol L-1, I=0.1 mol L-1 NaClO4, 50% ethanol, CDp; (1) 12.5×10-5. (2) 10×10-5, (3) 7.5×10-5, (4) 5×10-5, (5) 2.5×10-5 mol L-1.
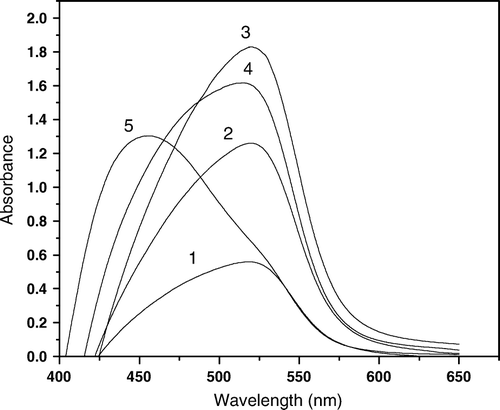
Figure 5. Absorption spectra for NPAS–Cu(II)–Dp complexes, CDp=12.5×10-5 mol L-1, Ctotal=15×10-5 M, I=0.1 mol L-1 NaClO4, 50% ethanol, CNPAS; (1) 12.5×10-5, (2) 10×10-5, (3) 7.5×10-5, (4) 5×10-5, (5) 2.5×10-5, (6) 0.25×10-5 mol L-1.
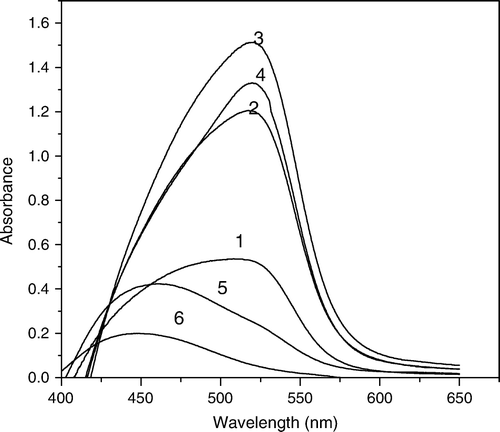
Figure 6. Contentious variation for ternary varied Dp λmax=525 nm, I=0.1 mol L-1 NaClO4, 50% ethanol, pH=7.3.
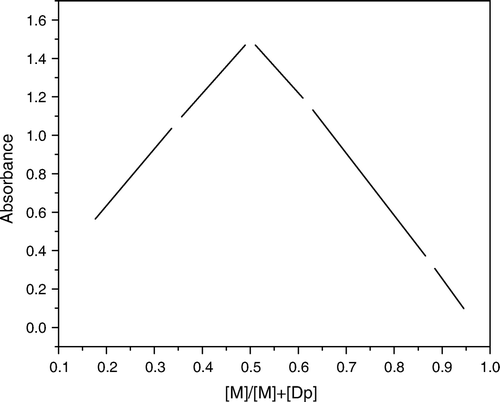
Figure 7. Contentious variation for ternary constant Cu2+ λmax=525 nm, I=0.1 mol L-1 NaClO4, 50% ethanol, pH=7.3.
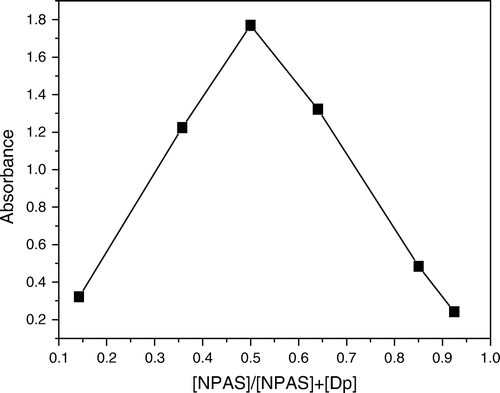
The formation of the mixed complex was investigated at different pH values using equimolar concentrations of components; the absorbance versus pH graphs at 525 nm indicated the existence of two basic equilibria that are sufficiently separated. The analysis of the two rising parts of these graphs within the pH ranges (5.7–6.6) and (6.7–7.8) were performed assuming the following scheme:
Transformation (1) was used for estimation of the molar absorptivity (ϵ2) of the [CuLA] ternary complex:
Table 2. Mean values of equilibrium constant (log K*), stability constants (log β), and molar absorptivity (ϵ) for Cu–NPAS–Dp, complexes [I=0.1M(NaClO4), 25°C, 50% (v/v) ethanol].
Calibration graph and reproducibility
At the optimum conditions and under the standard procedure, a linear calibration graph was obtained up to 2.2 µg mL-1 of Cu(II). A Ring boom plot showed that the optimum range for the determination of Cu(II) was 2.2–6.0 µg mL-1. The sensitivity of reaction was calculated according to Sandell and it was found to be 2.39×10-3 µg cm-2 of Cu(II). The reproducibility of the method was checked by analyzing two series of 10 solutions having Cu(II) concentrations of 2.5 and 4.5 µg mL-1. The relative standard deviations were found to be 0.61% and 0.58%, respectively. The detection limit was found to be 6.8 ng mL-1 (). The proposed three-dimensional (3D) structure is shown in .
Table 3. Conditions for the spectrophotometric determination of copper(II).
Effect of diverse ions
The effect of diverse ions on the determination of Cu(II) at the 3 µg mL-1 level was investigated. The criteria for interference are an absorbance value varying by more than ±2% from the expected value for Cu(II). The determination of Cu(II) as CuLA was possible in the presence of NH4 +, Cl-, Br-, I-, NO3 −, CIO4 −, acetate, EDTA, sulfate, citrate, borate, Ca2+, Zn2+, Hg2+, Ag+, MoO4 2–, Al3+, Ba2+, Sr2+ (up to 100-fold excess), Ni2+, Co2+, Fe3+, Mn2+, Sn2+, and Cd2+ (up to 90-fold excess). The alkali metal ions have no interfering effect on the determination of copper(II).
Applications
To confirm the usefulness of the proposed method, copper(II) was determined simultaneously in four pharmaceuticals vitamins, biological, and water samples (). The obtained results are in good agreement with the certified values of pharmaceuticals vitamins in their brochures, the RSD value was found in the range of 0.45% and 0.75% for copper(II) in all pharmaceutical vitamin samples.
Table 4. Determination of copper(II) in different samples.
Experimental
Instruments
Absorption measurements were performed on a Thermo Evolution 300 recording spectrophotometer using 10 mm matched quartz cells and slit width 2 nm (Thermo Company, England). The pH-meter (HANNA HI 223) equipped with a combined glass electrode was used for pH measurements (HANNA Egypt). The pH values in a water–ethanol medium were corrected as described elsewhere Citation32.
Chemicals
All chemicals were of analytical reagent grade and de-ionized water (or absolute ethanol) was used for preparation of solutions.
Standard procedure
Spectrophotometric determination of Cu(II)
A suitable portion of solution containing less than 75.0 µg of Cu(II) was introduced into a 25.0 mL calibrated flask, then a solution containing 2.5×10-4 mol L-1 of 5-(4-nitrophenylazo) salicylic acid NPAS and 2,2′-Dipyridyl Dp and 2.5 mL of 1.0 mol L-1 NaClO4 were added. After mixing with appropriate amount of ethanol, the pH of the solution was adjusted to pH 7.30 with dilute amounts of HClO4/NaOH and then complete to volume with bi-distilled water while keeping a final ethanol content at 50% (v/v). The absorbance was measured against the reagent blank at λmax 520 nm, after shaking for 3.0 min to attain the equilibrium. Absorbance of all solutions investigated remains constant for at least 5.0 hours.
Dissolution of commercial pharmaceutical preparations
Commercial pharmaceutical samples were provided from local Egyptian pharmaceutical industries. Ten capsules of each preparation were weighed accurately to determine the average weight of the capsules; the contents of them were grinded to fine powder, dissolved in a mixture of HNO3/H2SO4 (10:1) (50 ml+5 ml), and heated gently until charring began over a hot plate in the suction cupboard. The dropwise addition of HNO3 and boiling were continued until a colorless liquid was obtained. A few millimeters of water were added and the solution was evaporated to white fumes. A few millimeters of 0.1 mol L-1 perchloric acid were added, then we diluted to 100 mL with bi-distilled water.
Analysis of biological samples
The proposed method was applied for the determination of copper(II) in biological samples as tomato leaves and tea leaves. The foodstuffs were dried at 90°C for 24 hours. A 20–50 g of sample was digested with nitric acid and perchloric acid (1:1), then it was heated gently on a hot plate to dryness. The ash was taken up with 50 mL of HCl (10%) and evaporated to dryness. The residue was taken up in 20 mL concentration of HCl and was made up to 100 mL with distilled water. An aliquot of each solution was treated according to the present method and was compared with the AAS values.
Conclusions
Copper could be determined with high accuracy by a simple method. The reagents currently used for copper determination in aqueous media were limited and most of them require a chromogen for spectrophotometric methods. All methods that are sensitive for photometric determination of copper(II) contain hazard solvents. Our new method will lead to more use of green chemistry to save our health and environment. In addition, the ligands used in this study have several advantages over others currently utilized for photometric copper determination.These reagents form a highly stable 1:1:1 ternary complex with Cu(II) in 50% ethanol media at λmax 525 and molar absorptivity 2.60×104 L mol-1 cm-1.
Additional information
Notes on contributors
M.M. Seleim
DeceasedReferences
- Karadjova , I. ; Izgi , B. ; Gucer , S. Spectrochim. Acta, Part B 2002 , 57 , 581 – 590 .
- Ajtony , Z. ; Szoboszlai , N. ; Susko , E.K. ; Mezei , P. ; Gyorgy , K. ; Bencs , L. Talanta 2008 , 76 , 627 – 634 .
- Bermejo , P. ; Pena , E. ; Fompedrina , R. ; Bermejo , A. ; Fraga , J.M. Analyst 2001 , 126 , 571 – 575 .
- Lara , R. ; Cerutti , S. ; Sajonia , J.A. ; Olsina , R.A. ; Martinez , L.D. Food Chem. Toxicol . 2005 , 43 , 293 – 297 .
- Moreno , I.M. ; Gonzalez-Weller , D. ; Gutierrez , V. ; Marino , M. ; Camean , A.M. ; Gonzalez , A.G. Microchem. J. 2008 , 88 , 56 – 61 .
- Katerina , P. ; Christian , P. ; Ilse , S. ; Gregory , R. ; Josef , F.K.H. Analyst 1999 , 124 , 657 – 663 .
- Cesar , A.G. ; Joanna , L. ; Jon , C.B. ; Richard , G.C. Analyst 1999 , 124 , 1053 – 1059 .
- Seifert , W.F. and Bosma , A. 1994 . Vitamin A Deficiency Potentiates Carbon Tetrachloride-Induced Liver Fibrosis in Rats . Hepatology , 19 ( 1 ) : 193 – 201 .
- Zeng , A. ; Liu , E. ; Tan , S. ; Zhang , J. ; Gao , J. Electroanalysis 2002 , 14 , 1294 – 1298 .
- Reusch , W. Nuclear Magnetic Resonance Spectroscopy . Virtual Textbook of Organic Chemistry . http://www2.chemistry.msu.edu/faculty/reusch/VirtTxtJml/Spectrpy/nmr/nmr1.htm ( 1999 ).
- Liu , K.X. , Kato , Y. , Yamazaki , M. , Higuchi , O. , Nakamura , T. and Sugiyama , Y. 1993 . Decrease in the Hepatic Clearance of Hepatocyte Growth Factor in Carbon Tetrachloride-Intoxicated Rats . Hepatology , 17 ( 4 ) : 651 – 660 .
- Recknagel , R.O. , Glende , E.A. , Dolak , J.A. and Waller , R.L. 1989 . Mechanism of Carbon-tetrachloride Toxicity . Pharmacol. Ther. , 43 : 139 – 154 .
- Recknagel , R.O. 1967 . Carbon Tetrachloride Hepatotoxicity . Pharmacol. Rev. , 19 ( 2 ) : 401 – 408 .
- Masuda , Y. 2006 . Learning Toxicology from Carbon Tetrachloride-Induced Hepatotoxicity (in Japanese) . Yakugaku Zasshi , 126 ( 10 ) : 827 – 831 .
- Rood , A.S. , McGavran , P.D. , Aanenson , J.W. and Till , J.E. 2001 . Stochastic Estimates of Exposure and Cancer Risk from Carbon Tetrachloride Released to the Air from the Rocky Flats Plant . Risk Anal. , 21 ( 4 ) : 675 – 695 .
- Odabasi , M. 2008 . Halogenated Volatile Organic Compounds from the Use of Chlorine-Bleach-Containing Household Products . Environ. Sci. Technol. , 42 ( 5 ) : 1445 – 1451 .
- Fraser , P. 1997 . Chemistry of Stratospheric Ozone and Ozone Depletion . Australian Meteorological Magazine , 46 ( 3 ) : 185 – 193 .
- Evans , W.F.J. and Puckrin , E. 1996 . A Measurement of the Greenhouse Radiation Associated with Carbon Tetrachloride (CCl4) . Geophys. Res. Lett. , 23 ( 14 ) : 1769 – 1772 .
- www.wikipedia.org/wikipolyvinyl-chloride/
- Steiglitz , L. ; Vogg , H. Formation Decomposition of Polychlorodibenzodioxins and Furans in Municipal Waste ; Report KFK4379, Laboratorium fur Isotopentechnik, Institute for Heize Chemi: Kerforschungszentrum Karlsruhe, February 1988 .
- Kendüzler , E. ; Türker , A.R. Anal. Chim. Acta 2003 , 480 , 259 – 266 .
- Ohno , S. ; Teshima , N. ; Zhang , H. ; Sakai , T. Talanta 2003 , 60 , 1177 – 1185 .
- Reedy , B.K. Anal. Sci. 2003 , 19 , 423 – 432 .
- Pinto , J.J. ; Moreno , C. ; García-Vargas , M. Talanta 2004 , 64 , 562 – 565 .
- Shamsa , F. ; Barazandeh-Tehrani , M. DARU 2004 , 12 ( 2 ), 76 – 80 .
- Massoud , N.-Y. Anal. Sci. 2006 , 22 , 617 – 623 .
- Fu , D. ; Yuan , D. Spectrochim. Acta Part A 2007 , 66 , 434 – 437 .
- Yuan , L. ; Huo , S.H. ; Ren , X.N. ; Chen , H. Chin. Chem. Lett. 2008 , 19 , 92 – 94 .
- Reddy , S.A. ; Reddy , K.J. ; Narayana , S.L. ; Reddy , A.V. Food Chem. 2008 , 109 , 654 – 659 .
- Adriana do Nascimento Rocha , S. ; Dantas , A.F. ; Jaeger , H.V. Spectrochim. Acta Part A 2008 , 71 , 1414 – 1418 .
- Mitić , S.S. ; Micić , R.J. ; Simonović , R.M. Food Chem. 2009 , 117 , 461 – 465 .
- O'Haver , T.C. Anal. Proc. 1982 , 19 , 22 – 26 .
- Seleim , M.M. ; Abu-Bakr , M.S. ; Hashem , E.Y. ; El-Zohry, A. M. J. of Appl. Spectrosc. 2009 , 76 ( 4 ), 554 – 120 .
- Job , P. Ann. Chem. 1928 , 9 , 113 – 120 .
- Yoe , J. H. ; Jones , H.L. Indian Eng. Chem., Anal. Ed. 1944 , 16 , 111 – 119 .
- Shukla , Y.N. ; Tandon , J.S. ; Dhar , M.M. Indian J. Chem. 1973 , 11 , 528 – 535 .
- Marczenko , Z. Spectrophotometric Determination of Elements ; Geology Press: Beijing, 1983; p 211; translated by Y. Zheng .
- Inshizaki , T. ; Wada , H. ; Nakagawa , G. Anal. Chim. Acta 1988 , 212 , 253 – 260 .
- Ambhore , D.M. ; Joshi , A.P. J. Indian Chem. Soc. 1991 , 68 , 175 – 183 .
- Li , S.H. ; Li , S.Q. ; Chen , A.J. Talanta 1993 , 40 , 1085 – 1090 .
- Ali , A.E. Can. J. Chem. 1993 , 71 , 1002 – 1010 .
- Takashi , S. Talanta 1994 , 41 , 811 – 819 .
- Chimpalee , N. ; Chimpalee , D. ; Lohwithee , S. ; Nakwatchara , L. ; Burns , D.T. Anal. Chim. Acta 1996 , 329 , 315 – 318 .
- Xiaorong , Z. ; Yan , C. ; Zhenhua , F. Metall. Anal. 1998 , 18 , 26 – 33 .
- Nohut , S. ; Karaböcek , S. ; Güner , S. ; Gök , Y. J. Pharm. Biomed. Anal. 1999 , 20 , 309 – 315 .
- Chaisuksant , R. ; Palkawong-na-ayuthaya , W. ; Grudpan , K. Talanta 2000 , 53 , 579 – 585 .
- Ya-ling , Y. ; Qiu-fena , H. ; Ji-yuan , Y. Fenxi Shiyanshi 2002 , 21 , 73 – 78 .
- Dalman , Ö. ; Tüfekci , M. ; Nohut , S. ; Güner , S. ; Karaböcek , S. J. Pharm. Biomed. Anal. 2002 , 27 , 183 – 189 .