Abstract
Synthetic utility of sulfated and borate zirconia for the synthesis of α,α′-bis(substituted benzylidine)cycloalkanones using microwave irradiation in solvent-free conditions has been investigated. Both sulfated zirconia (SO4 2−/ZrO2) and borate zirconia (B2O3/ZrO2) demonstrated excellent catalytic activity for cross-aldol reaction of cycloalkanones with mono- and poly-substituted aromatic aldehydes. The significance of our finding relates to the minimization of volatile organic solvents, low reaction times, good yield of products, and ease of work up affording the reusability of catalyst.
Introduction
Due to their wide importance as precursors of potentially bioactive pyrimidine derivatives, α,α′-bis(substituted benzylidene)cycloalkanones have attracted considerable attention Citation1. Bis-benzylidene cycloalkanones displays a significant range of properties such as cytotoxicity Citation2 and histone acetyl transferase (HAT) inhibitory activities Citation3. Moreover, these compounds find several applications in perfumeries, agrochemical industries, as well as in the preparation of nonlinear optical materials and liquid crystalline polymers Citation4–6.
Conventionally, α,α′-bis(substituted benzylidene)cycloalkanones are synthesized via cross-aldol reaction of cycloalkanones and aromatic aldehydes using strong acidic or basic conditions, but there lies a possibility of self-condensation leading to the formation of unwanted side products with subsequent reduction in the yield Citation7. To overcome the problems associated with traditional reaction pathways, a search for alternative catalyst systems was necessary. A variety of metal (II) ions have been used to replace acids or bases, but the yields were not satisfactory in most cases Citation8. The continuing thrust to develop novel catalysts have resulted in the introduction of various reagents such as anhydrous RuCl3 Citation9, BMPTO Citation10, La+3 immobilized organic solids Citation11, Cp2TiPh2 Citation12, combination of KF with alumina Citation13, FeCl3.6H2O Citation14, InCl3 Citation15, SmI3 Citation16, TiCl3(CF3SO3) Citation17, TMSCl/NaI Citation18, TMSCl/Pd–C Citation19, SOCl2 Citation20, Yb(OTf)3 Citation21, Cu(OTf)2 Citation22, tri-ethyl amine with LiClO4 Citation23, and Mg(HSO4)2 Citation24 as catalyst for aldol condensation reactions. The use of ionic liquids Citation25, acidic alumina Citation26, CTAB/NaOH Citation27, copper(II) trifluoroacetate Citation28, lanthanide amides Citation29, sulphonic acid Citation30, silica sulfuric acid Citation31, K2CO3/PEG Citation32, and TCT Citation33 has also been proven effective for the synthesis of title compounds. Several recent literature have reported the use of molecular iodine Citation4, NaOAc Citation34, ZrCl4 Citation35 as benign catalysts for the synthesis of bis-benzylidene cycloalkanones. However, in most cases the yields are good at high temperatures and some of the reagents require longer reaction times with tedious purification procedures utilizing large quantities of volatile organic solvents.
In continuation of our efforts to develop a mild and sustainable protocol to carry out organic transformations Citation36 Citation37, we investigated the utility of solid super acids, specifically anion-modified zirconia toward the synthesis of title compounds. Abundant literature is available on SO4 2−/ZrO2 Citation38; however, its analogue superacid B2O3/ZrO2 is relatively less explored even though it showed almost similar catalytic performance like that of SO4 2−/ZrO2 toward several organic transformations Citation39. This prompted us to initiate systematic investigations on SO4 2−/ZrO2 and B2O3/ZrO2 catalyzed cross-aldol reaction of cycloalkanones with aromatic aldehydes.
Based on the previous successful studies within our group Citation40, we investigated the potential of SO4 2−/ZrO2 and B2O3/ZrO2 as heterogeneous catalysts for the synthesis of bis-benzylidine cycloalkanones using microwave (MW) irradiation (Scheme ). The use of MW irradiation with solvent-free conditions has been employed for a number of organic syntheses to minimize the reaction time, improve atom efficiency, energy savings, and to increase the product selectivity and yields Citation41 – the key requirements of sustainable chemistry Citation42.

To the best of our knowledge reports on this type of protocol for the synthesis of title compounds are very scanty. Herein we have reported our primary results for the synthesis of title compounds using SO4 2−/ZrO2 and B2O3/ZrO2 as benign heterogeneous catalysts. Furthermore, the present procedure is readily amenable to parallel synthesis and generation of combinatorial α,α′-bis(substituted benzylidene)cycloalkanones as reported in literature and as a consequence of one or more benefits associated with it such as mild conditions and shorter reaction times ().
Table 1. Synthesis of α,α′-bis(tolulidine)cyclohexanone c using different catalysts and reaction conditions.a
Results and discussion
A series of bis-benzylidine cycloalkanones was prepared by reacting cyclohexanone or cyclopentanone with various aromatic aldehydes using SO4 2−/ZrO2 and B2O3/ZrO2 as solid acid catalysts using MW irradiation (). The cross-aldol reaction of cyclohexanone with 4-methyl benzaldehyde was studied as a model reaction to synthesize the α,α′-bis(tolulidine)cyclohexanone. The catalysts used in the present study were synthesized by impregnating aqueous solution of corresponding acid over the zirconia surface. To optimize the acidity of the catalyst, the strength of sulfuric acid (H2SO4) solution used for surface modification of ZrO2 as well as the effect of calcination temperature of the SO4 2−/ZrO2 catalyst has been investigated. Two SO4 2−/ZrO2 catalysts were synthesized by impregnating H2SO4 solutions of concentration 1N and 2N designated as SZ-1 and SZ-2, respectively. The sulfated zirconia samples were calcined over a temperature range of 650–850°C. For the B2O3/ZrO2 catalyst, two samples were synthesized with variable borate loading; viz, 15 mol% and 30 mol% designated as BZ-15 and BZ-30, respectively.
Table 2. Synthesis of α,α’-bis(substituted benzylidene)cycloalkanones catalyzed by SO4 2−/ZrO2 and B2O3/ZrO2.a
Initially, we performed the reaction in organic solvents, but the yields were very low, even on prolonged reaction time with high catalyst loading. However, the reaction proceeded efficiently in the solvent-free conditions, completed within 15–20 minutes with good yields and purity of the products using both catalytic systems. In the case of SO4 2−/ZrO2 catalysts, although SZ-1 resulted in good yields, the required catalyst loading was quite high. For SZ-2, the requisite catalyst loading drops significantly to almost half, in comparison to SZ-1, using identical reaction conditions and substrate stichiometries ().
Table 3. Effect of catalyst loading on the modified zirconia catalyzed reaction of cyclohexanone with 4-methyl benzaldehyde.a
Among SO4 2−/ZrO2 samples, SZ-2 catalyst calcined at 650°C gave good results, which may be ascribed to formation of catalytically active tetragonal phase with strongly bonded sulfur species. On increasing the calcination temperature beyond 700°C, the tetragonal phase gradually transforms to a catalytically less active monoclinic phase () Citation38.
Figure 1. PXRD pattern of sulfated zirconia samples calcined at different temperatures: (a) 650°C, (b) 750°C, and (c) 850°C.
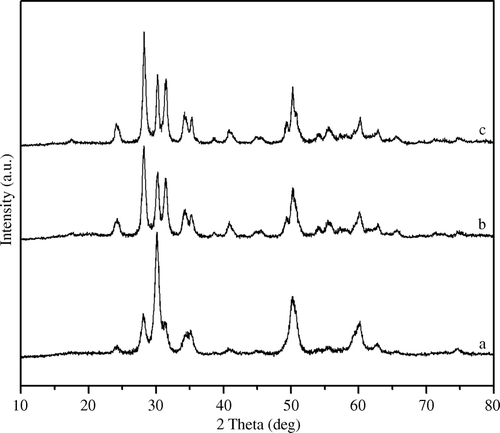
Sulfate group binding with the zirconia surface in a chelating bidentate fashion shows the IR bands of the SO4 2- group in the region of 1200–900 cm-1 with peaks at 1241, 1142, 1073, 1042, and 997 cm-1, characteristics of the inorganic chelating bidentate sulfate and are assigned to asymmetric and symmetric stretching frequencies of partially ionized S=O double bonds and S–O bonds Citation43. The FT-IR spectra of thermally regenerated catalyst showed the presence of almost similar bands (), illustrating no major leaching of sulfate groups from the zirconia surface; thereby, showing reusability up to five cycles with a slight loss in catalytic activity (). Sulfur content of sulfated zirconia is dependent on calcination temperature of the catalyst. It has been reported that sulfated zirconia catalyst calcined at 850°C, showed complete removal of surface sulfate species Citation44–46, as evident from its FT-IR spectroscopic studies.
Figure 2. FT-IR spectrum of sulfated zirconia catalyst: (a) fresh SZ-2 catalyst calcined at 650°C, (b) recycled SZ-2 catalyst activated at 450°C, and (c) SZ-2 catalyst calcined at 850°C.
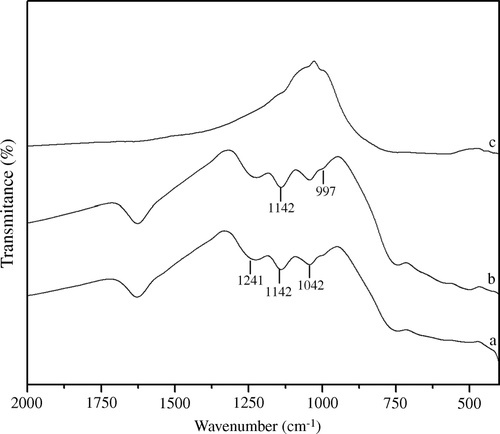
Table 4. Reusability studies of SO4 2−/ZrO2 and B2O3/ZrO2 catalyst on the reaction of cyclohexanone with 4-methyl benzaldehyde.a
In the case of B2O3/ZrO2 catalysts, BZ-15 and BZ-30 calcined at 650°C and showed almost similar catalytic performance, except for the shorter completion time for the BZ-30 reaction. The PXRD studies of the BZ catalyst calcined at 650°C revealed the cubic structure suggesting stabilization of zirconia into the cubic phase () Citation39. The presence of borate ions on the zirconia surface was confirmed with FT-IR spectroscopic studies. Fresh and recycled B2O3/ZrO2 catalyst showed IR peaks in the range of 1630 and 1100 cm−1 corresponding to borate ions () Citation47. In the first catalytic run, B2O3/ZrO2 catalysts demonstrated excellent catalytic activity but were reduced to some extent in successive runs, which may be attributed to leaching of borate from the zirconia surface.
Figure 3. PXRD patterns of borate zirconia catalyst: (a) 15 mol% B2O3/ZrO2, and (b) 30 mol% B2O3/ZrO2 calcined at 650°C.
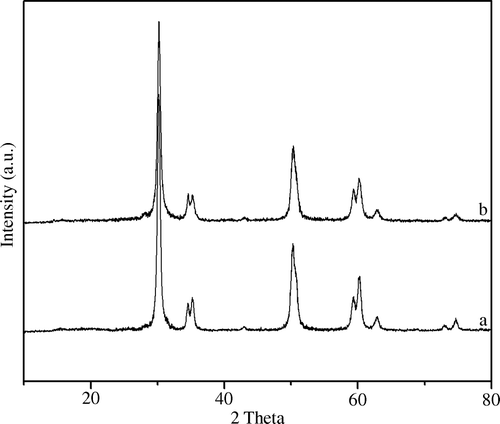
Figure 4. FT-IR spectrum of borate zirconia catalyst calcined at 650°C: (a) 15 mol% B2O3/ZrO2, (b) 30 mol% B2O3/ZrO2, and (c) recycled borate zirconia catalyst activated at 450°C.
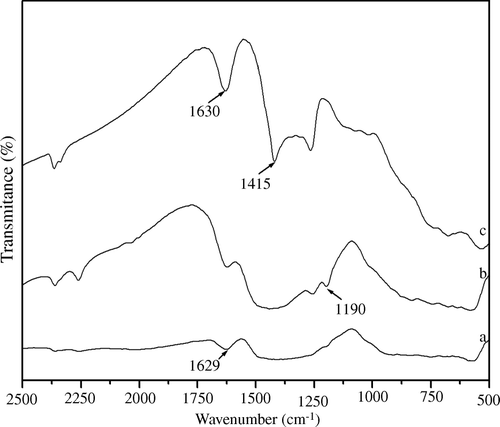
To optimize the reaction temperature, a set of reactions was performed over the range of 60 and 120°C, using identical catalyst loading and reaction time. In most of the cases, reaction temperature of 90–120°C resulted in good yields. Both cyclohexanone and cyclopentanone were subjected to condensation with aromatic aldehydes, containing either the electron-withdrawing or electron-releasing group in the presence of a catalyst in solvent-free conditions. The reaction leads exclusively to bis(benzylidine cycloalkanones). The attempted mono-condensation of cycloalkanones in the presence of 1:1 cyclic ketone:aldehydes resulted in a major bi-condensation product with minor mono-condensation products. Substituted aromatic aldehydes with either +I or −I effect, favored the product formation. The probable reaction mechanism of the present reaction can be derived as acid catalyzed cross-condensation of an enolizable ketone and nonenolizable aldehydes. The feasibility of various aldehydes toward product formation could be ascribed to the combination of various electronic effects such as inductive, mesomeric, and hyperconjugation. The reaction proceeds efficiently with substituents imparting the +I as well as the −I effect (). The electron releasing −CH3 group stabilizes the electrophilic carbonyl group with hyperconjugation effect, while electron withdrawing fluoro-substituted compounds generate the electrophilicity at carbonyl carbons of aromatic aldehydes with a strong −I effect, thereby favoring the attack of enolate at carbonyl site. The chloro- and bromo-substituted aromatic aldehydes with the −I effect and the +M mesomeric effect resulted in the backflow of electron density over an aromatic ring of the aldehydes, thereby decreasing the electron deficiency at carbonyl carbon of aldehydes, and thus slightly hindered the reaction. The fluoro-substituted aromatic aldehydes with no mesomeric effect resulted in corresponding bis(benzylidine cycloalkanones) in good yields.
The catalysts were found tolerant to the range of substituted aromatic aldehydes. Apart from several mono-substituted substrates, highly substituted aromatic aldehydes such as 2,3,4,5,6-pentafluoro benzaldehyde reacts efficiently with cyclohexanone and cyclopentanone. To the best of our knowledge, compounds depicted in entry 7; α,α′-bis(2,3,4,5,6-pentafluoro benzylidine)cyclohexanone and entry 14; α,α′-bis(2,3,4,5,6-pentafluoro benzylidine)cyclopentanone are hitherto unreported. Both these compounds were synthesized in good yields and optimal purity. All synthesized compounds were characterized with standard analytical techniques such as NMR, GC-MS and melting points were compared with those previously reported. The structure of the compound () in entry 7 is authenticated using single crystal X-ray diffraction data.Footnote1
Figure 5. ORTEP diagram of α,α′-bis(2,3,4,5,6-pentafluoro benzylidine)cyclohexanone, Entry 7 with atom numbering scheme (40% probability factor for the thermal ellipsoids).
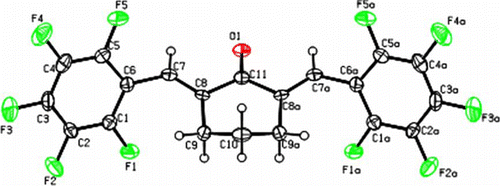
No product was obtained for the reaction of 4-nitro benzaldehyde with cyclohexanone as well as cyclopentanone even with high catalyst loading or prolonged reaction time. The 4-nitro benzaldehyde being solid, its solubility in studied cyclic ketones may be less, hence hindered the feasibility of reaction. There was no reaction even in the polar or nonpolar solvent. There was a slight decrease in yield for all aromatic aldehydes with cyclopentanone as compared with cyclohexanone and may be attributed to ring strains in the comparatively smaller ring size of cyclopentanone Citation32.
Experimental
Materials
Zirconium oxychloride was procured from Loba Chemie, India. Sulfuric acid, boric acid, aqueous ammonia (30% solution) – common organic solvents – were from S.d. fine chemicals Ltd, India. Cyclic ketones and aromatic aldehydes were of analytical grade purchased from commercial suppliers.
General
All the reactions were carried out in a Sineo MAS-II microwave synthesis workstation using a 10 ml quartz glass vessel fitted with a reflux condenser. Melting points were recorded with Metler-Toledo melting point apparatus and are uncorrected. The 1H and 13C NMR spectra were recorded in CDCl3 on a Bruker AX 500 spectrometer (500 MHz for 1H NMR and 125 MHz for 13C NMR). The GC-MS analyses were performed on Shimadzu QP-2010 spectrometer. Elemental analyses were carried out on a Perkin-Elmer 2400 CHNS/O analyzer. The FT-IR spectra were recorded in the range of 400 and 4000 cm−1 as KBr pellets on Perkin-Elmer GX spectrophotometer. Crystallinity of SO4 2−/ZrO2 and B2O3/ZrO2 after calcination was measured by the X-ray powder diffractometer (Rigaku) using Cu Kα radiation (?=1.54056 Å). The samples were scanned in 2θ range of 2 and 80°.
Catalyst synthesis
The SO4 2−/ZrO2 and B2O3/ZrO2 catalysts were synthesized by reported procedures Citation38 Citation39. A two-step process involves the preparation of zirconium hydroxide, followed by acid impregnation in the second step. The synthesized catalysts were preliminary characterized with PXRD and FT-IR analyses.
Representative procedure for the synthesis of α,α′-bis(substituted benzylidene)cycloalkanones
Cyclic ketone (5 mmol), aromatic aldehyde (10 mmol), and calculated quantity of catalyst (SO4 2−/ZrO2 or B2O3/ZrO2, preactivated at 450°C for 2 hours) were subjected to microwave irradiation at 90–120°C working at 800 W with magnetic stirring. The progress of the reaction was monitored using TLC. After completion of reaction, the reaction mixture was cooled, poured in ethanol, and filtered to recover the catalyst. After the evaporation of solvent, corresponding bis-benzylidene cycloalkanones were recovered in the form of needles. The catalyst was washed with warm acetone, dried at 110°C for 2 hours and activated at 450°C for 2 hours prior to successive catalytic runs.
Selected spectral data for characterization of products
Entry 3: Mp 167–169°C, 1H NMR (500 MHz, CDCl3): δ=7.73 (s, 2H, =CH), 7.14 ∼ 7.32 (dd, 8H, ArH, J=8Hz), 2.85 (t, 4H, J=6 Hz), 2.31 (s, 6H), 1.71 (m, 2H, J=6Hz). MS (EI): m/z (%) 302 (100) [M]+.
Entry 7: Mp 184–186°C, 1H NMR (500 MHz, CDCl3): δ=7.43 (s, 2H, =CH), 2.63 (t, 4H, J=6 Hz, 1.82 (m, 2H, J=6Hz). MS (EI): m/z (%) 454 (100) [M]+.
Entry 8: Mp 241–243°C, 1H NMR (500 MHz, CDCl3): δ=7.57 (s, 2H, =CH), 7.23 ∼ 7.50 (dd, 8H, ArH, J=8Hz), 3.08 (s, 4H), 2.39 (s, 6H). MS (EI): m/z (%) 288 (100) [M]+.
Entry 14: Mp 138–140°C, 1H NMR (500 MHz, CDCl3): δ=7.40 (s, 2H, =CH), 2.76 (s, 4H), MS (EI): m/z (%) 440 (100) [M]+.
Conclusions
The SO4 2−/ZrO2 and B2O3/ZrO2, prepared using conventional methods, found a highly efficient catalyst for the cross-aldol condensation of cycloalkanones and aromatic aldehydes resulting in corresponding α,α′-bis(substituted benzylidene)cycloalkanones in high yields using microwave irradiation and solvent-free conditions. Besides various mono-substituted aromatic aldehydes, multi-substituted aldehydes substrates such as 2,3,4,5,6-pentafluoro benzaldehyde reacts efficiently with cycloalkanones using the present reaction protocol.
Acknowledgements
We gratefully acknowledge the Council of Scientific and Industrial Research (CSIR), New Delhi, India for funding under the network project (NWP-0010). Thanks are also due to the Analytical Sciences Discipline of the Institute for Analysis Facilities.
Notes
1. X-ray crystal data for α,α′-bis (2,3,4,5,6-pentafluoro benzylidine) cyclohexanone; entry 7, Table 2 (CCDC 795857): Empirical formula: C20H8F10O, Formula weight: 454.26, T=293Citation2 K, λ=0.71073 Å, crystal system: Orthorhombic, space group: Pnma, unit cell dimensions: a=8.955Citation2 Å, b=32.636Citation9 Å, c=5.9410Citation18 Å, V=1736.3Citation8Å3, Z=4, density(calcd.)=1.738 Mg/m3, absorption coefficient: 0.180 mm−1, F(000)=904, crystal size: 0.23×0.11×0.06 mm, reflections collected: 7191, Independent reflections: 1387, R (int)=0.0887, data/restraints/parameters: 1387/0/163, Goodness of fit on F 2=1.290, final R indices [I≥2s(I)] R1=0.1071, wR2=0.1995, R indices (all data): R1=0.1406, wR2=0.2146, largest diff. peak and hole: 0.265 and −0.424 e.Å3. CCDC 795857 contains the supplementary crystallographic data for this paper. These data can be obtained free of charge from Cambridge Crystallographic Data Centre via http://www.ccdc.cam.ac.uk/data_request/cif
References
- Deli , J. ; Lorand , T. ; Szabo , D. ; Foldesi , A. Pharmazie 1984 , 39 , 539 – 540 .
- Dimmock , J.R. ; Hamon , N.W. ; Hindmarsh , K.W. ; Sellar , A.P. ; Turner , A.W. ; Rank , G.H. ; Robertson A.J. J. Pharm. Sci . 1976 , 65 , 538 – 543 .
- Costi , R. ; Santo , R.D. ; Artico , M. ; Miele , G. ; Valentini , P. ; Novellino , E. ; Cereseto , A. J. Med. Chem . 2007 , 50 , 1973 – 1977 .
- Das , B. ; Thirupathi , P. ; Mahender , I. ; Reddy , K.R. J Mol. Catal. A: Chem . 2006 , 247 , 182 – 185 .
- Kawamata , J. ; Inoue , K. ; Inabe , T. ; Kiguchi , M. ; Kato , M. ; Taniguchi , Y. Chem. Phys. Lett . 1996 , 249 , 29 – 34 .
- Gangadhara , K. ; Kaushal , K. Polymer . 1995 , 36 , 1903 – 1910 .
- Nakano , T. ; Irifune , S. ; Umano , S. ; Inada , A. ; Ishii , Y. ; Ogawa M. J. Org. Chem. 1987 , 52 , 2239 – 2244 .
- Irie , K. ; Watanabe , K. Bull. Chem. Soc. Jpn . 1980 , 53 , 1366 – 1371 .
- Iranpoor , N. ; Kazemi , F. Tetrahedron 1998 , 54 , 9475 – 9480 .
- Zheng , M. ; Wang , L. ; Shao , J. ; Zhong , Q. Synth. Commun . 1997 , 27 , 351 – 354 .
- Dewa , T. ; Saiki , T. ; Aoyama , Y. J. Am. Chem. Soc . 2001 , 123 , 502 – 503 .
- Nakano , T. ; Migita , T. Chem. Lett . 1993 , 12 , 2157 – 2158 .
- Yadav , J.S. ; Reddy , B.V.S. ; Nagaraju , A. ; Sarma , J.A.R.P. Synth. Commun . 2002 , 32 , 893 – 896 .
- Zhang , X. ; Fan , X. ; Niu , H. ; Wang , J. Green Chem . 2003 , 5 , 267 – 269 .
- Deng , G. ; Ren , T. Synth. Commun . 2003 , 33 , 2995 – 3001 .
- Wang , X. ; Zhang , Y. Chin. Chem. Lett . 2004 , 15 , 511 – 514 .
- Iranpoor , N. ; Zeynizadeh , B. ; Aghapour , A. J. Chem. Res. Synop . 1999 , 9 , 554 – 555 .
- Sabitha , G. ; Reddy , G.S.K.K. ; Reddy , K.B. ; Yadav , J.S. Synthesis 2004 , 2 , 263 – 266 .
- Zhu , Y. ; Pan , Y. Chem. Lett . 2004 , 33 , 668 – 669 .
- Hu , Z. ; Liu , J. ; Zeng , P. ; Dong , Z. J. Chem. Res. Synop . 2004 , 1 , 55 – 56 .
- Wang , L. ; Sheng , J. ; Tian , H. ; Han , J. ; Fan , Z. ; Qian , C. Synthesis 2004 , 18 , 3060 – 3064 .
- Li , J. ; Su , W. ; Li , N. Synth. Commun . 2005 , 35 , 3037 – 3043 .
- Arnold , A. ; Markert , M. ; Mahrwald , R. Synthesis 2006 , 7 , 1099 – 1102 .
- Salehi , P. ; Khodaei , M. ; Zolfigol , A. ; Keyvan , A. Monatsh. Chem . 2002 , 133 , 1291 – 1295 .
- Zhang , X. ; Fan , X. ; Niu , H. ; Wang , Chin, Chem. Lett . 2003 , 14 , 1005 – 1007 .
- Esmaeili , A.A. ; Tabas , M.S. ; Nasseri , M.A. ; Kazemi , F. Monatsh. Chem . 2005 , 136 , 571 – 576 .
- Shrikhande , J.J. ; Gawande , M.B. ; Jayaram , R.V. Catal. Commun . 2008 , 9 , 1010 – 1016 .
- Song , D. ; Chen , Y. ; Wang , R. ; Liu , C. ; Jiang , H. ; Luo , G. Prep. Biochem. Biotechnol . 2009 , 39 , 201 – 207 .
- Zhang , L. ; Wang , S. ; Sheng , E. ; Zhou , S. Green Chem . 2005 , 7 , 683 – 686 .
- An , L.T. ; Zhou , J.P. ; Zhang , L.L. Catal. Commun . 2008 , 9 , 349 – 354 .
- Salehi , P. ; Dabiri , M. ; Zolfigol , M.A. ; Fard , M.A.B. J. Braz. Chem. Soc . 2004 , 15 , 773 – 776 .
- Cao , Y. ; Dai , Z. ; Zhang , R. ; Chen , B. Synth. Commun . 2005 , 35 , 1045 – 1049 .
- Bigdeli , M.A. ; Mahdavinia , G.H. ; Jafari , S. ; Hazarkhani , H. Catal. Commun . 2007 , 8 , 2229 – 2231 .
- Rahman , A.F.M.M. ; Jeong , B. ; Kim , D. ; Park , J. ; Lee , E. ; Jahng , Y. Tetrahedron 2007 , 63 , 2426 – 2431 .
- Kumar , A. ; Akanksha J Mol. Catal. A: Chem . 2007 , 274 , 212 – 216 .
- Jadhav , S.V. ; Ingole , P.G. ; Bajaj , H.C. Synth Commun . 2010 , 8 , 1142 – 1148 .
- Jadhav , S.V. ; Jinka , K.M. ; Bajaj , H.C. Appl Catal A: Gen 2010 , 390 , 158 – 165 .
- Reddy , B.M. ; Patil , M.K. Chem. Rev . 2009 , 109 , 2185 – 2208 .
- Madje , B.R. ; Patil , P.T. ; Shindalkar , S.S. ; Benjamin , S.B. ; Shingare , M.S. ; Dongare , M.K. Catal. Commun . 2004 , 5 , 353 – 357 .
- Tyagi , B. ; Mishra M.K. ; Jasra , R.V. J. Mol. Catal. A: Chem . 2008 , 286 , 41 – 46 .
- Loupy , A. , Microwave in Organic Synthesis ; Wiley-VCH : Weinheim , 2002 .
- Anastas , P.T. ; Warner , J.C. In Green Chemistry: Theory and Practice ; Oxford University Press: New York , 1998 .
- Yamaguchi , T. ; Jin T. ; Tanabe , K. J. Phys. Chem . 1986 , 90 , 3148 – 3152 .
- Chen , W.H. ; Ko , H.H. ; Sakthivel , A. ; Huang , S.J. ; Liu , S.H. ; Lo , A.Y. ; Tsai , T.C. ; Liu , S.B. Catal. Today 2006 , 116 , 111 – 120 .
- Chen , F.R. ; Coudurier , G. ; Joly , J.F. ; Védrine , J.C. J. Catal . 1993 , 143 , 616 – 626 .
- Song , X. ; Sayari , A. Catal. Rev. Sci. Eng . 1996 , 38 , 329 – 412 .
- Osiglio , L. ; Romanelli , G. ; Blanco M. J Mol Catal A: Chem . 2010 , 316 , 52 – 58 .