Abstract
Commercially available lithium hydroxide monohydrate (LiOH·H2O) was found to be a novel “dual activation” catalyst for Knoevenagel condensation between malononitrile (1) or 3-methyl 1-phenyl-1H-pyrazol-5-(4H)-one (6) with aromatic aldehydes 2a–e leading to an efficient and easy synthesis of arylidenemalononitriles 3a–d and 4,4′-(arylmethylene)bis(3-methyl-1-phenyl-1H-pyrazol-5-ols) 7a–c in short times. The reaction of aryl aldehydes with malononitrile afforded excellent yields after 1–6 min in aqueous media at room temperature. In case of 3-methyl-1-phenyl-1H-pyrazol-5-(4H)-one (6) and aromatic aldehydes afforded good yields after 60–75 min at 90°C.
Introduction
The literature revealed that organic reactions under solvent-free Citation1, and aqueous Citation2, conditions have increasingly attracted chemists’ interests, particularly from the view point of green chemistry Citation3. Generally, Knoevenagel reactions are carried out by the condensation of active methylene compounds with aldehydes in the presence of organic bases, or ammonium salts Citation4. Alternative protocols for Knoevenagel condensations catalyzed by Lewis acids such as TiCl4 Citation5, ZnCl2 Citation6, LiCl Citation7, and various heterogeneous solid bases sulfate-ion promoted Zirconia Citation8, clay Citation9, and layered double hydroxides (LDHs) Citation10, have been reported in literature. Recently, ionic liquids such as [hmim] PF6 Citation11 have been proven to be an efficient catalyst for Knoevenagel condensation.
Furthermore, Bigi's group described the same reactions which could proceed efficiently in water Citation12. More recently, we found that aldehydes reacted with malononitrile efficiently in the absence of catalyst and solvent under microwave irradiation and thermal heating conditions Citation13. 4,4′-(Arylmethylene)bis(1H-pyrazol)-5-ols are applied as fungicides Citation14, pesticides Citation15, dyestuffs Citation16, and as the chelating and extracting reagents for different metal ions Citation17. The conventional chemical approach to 4,4′-(arylmethylene)bis(3-methyl-1-phenyl-1H-pyrazol-5-ols) involves the successive Knoevenagel synthesis of the corresponding arylidinepyrazolones and its base-promoted Michael reaction and also one-pot tandem Knoevenagel–Michael reaction of arylaldehydes with two equivalents of 3-methyl-1-phenyl-1H-pyrazol-5(4H)-one Citation6 performed under a variety of reaction conditions Citation18. The first set of procedures utilizes the catalysis of the components with piperidine in ethanolic solution Citation19. The second set of methods involves the noncatalyzed tandem Knoevenagel–Michael reaction under neutral conditions in either ethanol Citation20 or benzene Citation21 solutions. Although it affords the corresponding (4,4-arylmethylene)bis(1H-pyrazol)-5-ols in reliable 70–90% yields, the reaction requires 3–12 h of initial reflux with a further 24 h under ambient temperature to go to completion. Finally, Wang et al. Citation22 reported its synthesis in water using sodium dodecyl sulphate as the surfactant catalyst over a 1-h period, but the process needs a temperature of 100°C. Further, Elinson et al. Citation23 utilized electro-catalytic procedure for its synthesis. However, most of the methods suffer from at least one limitation that may include moderate yields, long reaction times, harsh reaction conditions or tedious workup procedures Citation12. Recently, the use of LiOH·H2O has received considerable attention as a cheap and easily available reagent in organic reactions. We have found that, it acts as a “dual activation” agent for chemoselective methyl ether Citation24 and methyl ester Citation25 formation providing an alternating method to the toxic diazomethane protocol. Furthermore, it acts as “dual activation” catalyst for tandem cross aldol condensation between acyclic/cyclic ketones and aromatic, heteroaromatic/styryl/alkyl aldehydes Citation26. Herein, we report our study on the Knoevenagel condensations of aldehydes with malononitrile Citation1 or 3-methyl-1-phenyl-1H-pyrazol-5(4H)-one Citation6 under aqueous conditions in the presence of LiOH·H2O.
Result and discussion
This work was initiated with the reaction of 4-methoxybenzaldehyde with malononitrile in a variety concentration of LiOH·H2O. Thus, malononitrile Citation1 (1 mmol) was treated with 4-methoxybenzaldehyde Citation2 (1 mmol) in the presence of LiOH·H2O (5, 10, and 15 mmol%) in H2O (10 mL) as shown in , we found that the reaction was accelerated by LiOH·H2O (5 mmol) in 87% after 1 min ().
Table 1. The reaction of 4-methoxybenzaldehyde with malononitrile in a variety concentrations of lithium hydroxide.
Furthermore, the same reaction in the presence of NaOH, KOH, and piperidine under similar conditions was examined. As shown in ; LiOH·H2O produce good yield of 3b. No Knoevenagel condensation was observed when 1 was treated with 2b in the absence of LiOH·H2O either in the presence or absence of solvent () (Table 1).
Table 2. The reaction of malononitrile (1) with 4-methoxybenzaldehyde (2b) in the presence and absence of different bases.
The dual role of LiOH·H2O, that is, generates the enolate from the malononitrile and activates the aldehyde carbonyl by coordination with Li+ is demonstrated in . Proton abstraction from 1 by LiOH·H2O (present in catalytic amount) generates the lithium enolate I. Coordination of the Li+ cation of I with the aldehyde carbonyl oxygen forms the six-membered cyclic transition state II and increases the electrophilicity of the aldehyde carbonyl group and makes it more susceptible to nucleophilic attack in an intramolecular fashion to form the iminolate anion III. The iminolate anion III subsequently abstracts the proton of 1 and generates the iminolate I to complete the catalytic cycle. The ol IV on dehydration results in the formation of arylidenemalononitrile V (, ) ().
Figure 3. Dual activation role of LiOH·H2O Knonovenagel condensation between a malononitrile and aldehydes.
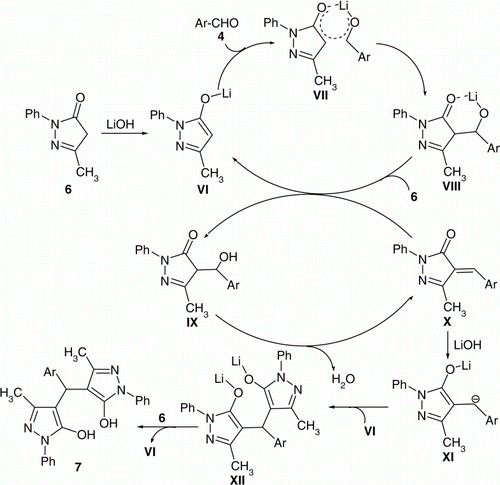
To check the generality of the catalytic system, malononitrile was treated with various aryl aldehydes under the catalytic influence of LiOH·H2O (5 mol%, ). Excellent results (76–94 yields) were obtained and the reactions were completed after 1–7 min. The reactions could be monitored visually and precipitated out in the reaction medium due to poor solubility in H2O at room temperature. Thus, the formation of a yellow or light orange precipitate indicated completion of the reaction (; ).
Table 3. The reaction of malononitrile with different aldehydes with different reaction times and yield percent.
The reaction of malononitrile Citation1 with salicyaldehyde Citation4 under the same condition afforded the corresponding iminocoumarin derivative 5 ().
The synthesis of 4,4′-(arylmethylene)bis(3-methyl-1-phenyl-1H-pyrazol-5-ols) 7a–d involved the reaction of the electrophilic substitution reaction of 3-methyl-1-phenyl-1H-pyrazol-5(4H)-one Citation6 with aryl aldehydes in water. As a test case, 4-methoxybenzaldehyde reacted with 3-methyl-1-phenyl-1H-pyrazol-5(4H)-one Citation6 in water with different catalytic amounts of LiOH·H2O and at different reaction temperatures in order to optimize the reaction conditions. Thus, 3-methyl-1-phenyl-1H-pyrazol-5(4H)-one Citation6 (2 mmol) was treated with 4-methoxybenzaldehyde (2b) (1 mmol) in the presence of LiOH·H2O (5, 10, and 15% mmol) in H2O (10 mL) at room temperature and 90°C as shown in , we found that the reaction was accelerated by 10 mmol of LiOH·H2O at 90°C. in 83.5% after 1 h ().
Table 4. The reaction of 3-methyl-1-phenyl-1H-pyrazol-5(4H)-one (6) with 4-methoxybenzaldehyde (2b) using different concentrations of lithium hydroxide.
To show the generality and scope of this synthetic method, the electrophilic substitution reaction of 3-methyl-1-phenyl-1H-pyrazol-5(4H)-one Citation6 with different aldehydes was studied in the presence of LiOH·H2O in water, using optimized reaction conditions (, ; ).
Table 5. The reaction of 3-methyl-1-phenyl-1H-pyrazol-5(4H)-one (6) with different aldehydes 2a–d catalyzed by LiOH·H2O (10 mol%) in water as solvent.
The possible mechanism for the synthesis of 7a–d is representing in . Proton abstraction from 6 by LiOH·H2O (present in catalytic amount) generates the lithium enolate VI. Coordination of the Li+ cation VI with the aldehyde carbonyl oxygen forms the six-membered cyclic transition state VII and increases the electrophilicity of the aldehyde carbonyl group and makes it more susceptible to nucleophilic attack in an intramolecular fashion to form the aldolate anion VIII. The aldolate anion VIII subsequently abstracts the proton from 6 and generates the enolate VI to complete the catalytic cycle. The aldol IX on dehydration results in the formation of 4-arylidene-3-methyl-1-phenyl-1H-pyrazol-5(4H)-one X which reacted with LiOH·H2O to form the enolate XI, condensation with VI to form the corresponding bis-enolate anion XII, which subsequently abstracts proton from 6 and generate the lithium enolate VI to complete the catalytic cycle. The chemical structures of the newly synthesized compounds were characterized by IR (Infrared) and NMR (Nuclear Magnetic Resonance) spectral analysis (C.f. “Experimental” section).
Experimental
All melting points are recorded on Gallenkamp electric melting point apparatus and are uncorrected. The IR spectra υ (cm−1) (KBr) were recorded on a Perkin Elmer Infrared Spectrophotometer Model 157. The 1H NMR spectra were obtained on a JEOL Spectrophotometer at 500 MHz, using Tetramethylsilane (TMS) as an internal reference and DMSO-d 6 as solvent and were carried out in the National Research Centre, Dokki, Giza, Egypt. Elemental analyses (C, H, and N) were carried out at the Microanalytical Centre of Cairo University, Giza, Egypt.
Synthesis of 2-(substituted-methylene)malononitriles 3a–e and 2-imino-2H-chromene-3-carbonitrile (5)
Malononitrile Citation1 (330.3 mg, 5 mmol) in water (10 mL) was treated with LiOH·H2O (1.05 mg, 0.25 mmol) under magnetically stirred condition for 1 min at room temperature (25–30°C) followed by aromatic aldehyde namely; benzaldehyde (53.06 mg, 5 mmol), 4-methoxybenzaldehyde (68.08 mg, 5 mmol), 4-chlorobenzaldehyde (70.29 mg, 5 mmol), furfuraldehyde (48.04 mg, 5 mmol), 4-dimethylamino-benzaldehyde (74.6 mg, 5 mmol), or salicyaldehyde (61.06 mg, 5 mmol). The reaction mixture was stirred at room temperature for 1–7 min. After the completion of the reaction, a precipitate was formed and this served as indicator for monitoring the reaction visually. The formed precipitate was filtered and crystallized from ethanol to give 3a–e and 5, respectively.
2-Benzylidenemalononitrile (3a)
Yellowish white powder, mp 85°C [Lit. Citation27 83°C]. IR (KBr) νmax. 2219 (CN), 1581 (C = C) cm−1; 1H NMR (DMSO-d 6) δ 7.58 (t, 2H, J = 8.45 Hz), 7.64 (t, 1H, J = 8.25), 7.90 (d, 2H, J = 8.4 Hz), 8.48 (s, 1H, CH=); 13C NMR (DMSO-d 6) δ 169.1, 134.6, 131.0, 130.7, 129.8, 113.6, 112.5, 82.6. Anal. Calc. for C10H6N2 (154.17): C, 77.91; H, 3.92; N, 18.17. Found: C, 77.88; H, 3.87; N, 18.13.
2-(4-Methoxybenzylidene)malononitrile (3b)
Yellow crystals, mp 118°C [Lit. Citation6 118–119°C]. IR (KBr) νmax. 2219 (CN), 1578 (C=C) cm−1; 1H NMR (DMSO-d 6) δ 3.82 (s, 3H), 7.14 (d, 2H, J= 9.28 Hz), 7.92 (d, 2H, J= 9.15 Hz), 8.33 (s, 1H, CH=); 13C NMR (DMSO-d 6) δ 162.0, 158.8, 132.9, 124.1, 115.4, 114.0, 113.8, 78.9, 56.1. Anal. Calc. for C11H8N2O (184.19): C, 71.73; H, 4.38; N, 15.21. Found: C, 71.74; H, 4.40; N, 15.24.
2-(4-Chlorobenzylidene)malononitrile (3c)
Yellowish white crystals, mp 167°C [Lit. Citation26, 165°C]. IR (KBr) νmax. 2221 (CN), 1583 (C = C) cm−1; 1H NMR (DMSO-d 6) δ 7.65 (d, 2H, J = 8.4 Hz); 7.89 (d, 2H, J = 8.4 Hz), 8.4 (s, 1H, CH = ); 13C NMR (DMSO-d 6) δ 158.8, 141.6, 132.0, 130.1, 129.0, 113.8, 112.3, 83.0. Anal. Calc. for C10H5ClN2 (188.61): C, 63.68; H, 2.67; N, 14.85. Found: C, 63.71; H, 2.65; N, 14.87.
2-(Furan-2-ylmethylene)malononitrile (3d)
Pale yellow solid, mp 70 [Lit. Citation28, 68−69°C]. IR (KBr) νmax. 2221 (CN), 1583 (C=C) cm−1; 1H NMR (DMSO-d 6) δ 6.87–6.88 (m, 1H, furyl), 7.41 (d, 1H, J = 3.05 Hz, furyl), 7.81 (d, 1H, J= 1.6 Hz, furyl), 8.22 (s, 1H, C=CH). Anal. Calc. for C8H4N2O (144.13): C, 66.67; H, 2.80; N, 19.44. Found: C, 66.71; H, 2.78; N, 19.41.
2-(4-(Dimethylamino)benzylidene)malononitrile (3e)
Red crystals, mp 179°C [Lit. Citation29, 180°C]. IR (KBr) νmax. 2208 (CN), 1565 (C=C) cm−1; 1H NMR (DMSO-d 6) δ 3.04 (brs, 6H, 2CH3), 7.79 (d, 2H, J = 9.2 Hz), 7.91 (d, 2H, J = 9.15 Hz), 8.05 (s, 1H, CH = ). Anal. Calc. for C12H11N3 (197.24): C, 73.07; H, 5.62; N, 21.30. Found: C, 73.12; H, 5.67; N, 21.35.
2-Imino-2H-chromene-3-carbonitrile Citation5
Yellow solid, mp 140–141°C [Lit. Citation30 140–141°C]. IR (KBr) νmax. 3332 (NH), 2190 (CN), 1639 (C=N), 1256 (C–O) cm−1; 1H NMR (DMSO-d 6) δ 7.09–7.47 (m, 4H, Ar–H), 8.31 (s, 1H, C4–H, coumarin), 8.77 (s, 1H, NH). Anal. Calc. for C10H6N2O (170.17): C, 70.58; H, 3.55; N, 16.46. Found: C, 70.51; H, 3.63; N, 16.52.
Typical procedure for the synthesis of 4,4′-(substituted-methylene)bis(3-methyl-1-phenyl-1H-pyrazol-5-ols) 7a–d
To aromatic aldehydes namely; benzaldehyde (1.06 g, 10 mmol), 4-anizaldehyde (1.36 g, 10 mmol), 4-chlorobenzaldehyde (1.41 g, 10 mmol) or furfuraldehyde (0.96 g, 10 mmol) and pyrazolone 6 (3.48 g, 20 mmol) was added to a stirring solution of LiOH·H2O (0.042 g, 1 mmol) in water (20 mL). The mixture was heated over a water bath at 90°C for an appropriate time (). The formed precipitate was filtered, dried, and recrystallized from ethanol to give 7a–d.
4,4′-(Phenylmethylene)bis(3-methyl-1-phenyl-1H-pyrazol-5-ol) (7a)
Colorless powder, mp 170–171°C [Lit. Citation31, 174°C]. IR (KBr) νmax. 3030 (br, OH), 1573 (C=C) cm−1; 1H NMR (DMSO-d 6, 500 MHz) δ 2.27 (s, 6H, 2CH3), 4.91 (s, 1H, CH), 7.13 (m, 1H), 7.22 (m, 6H), 7.39 (t, 4H, J = 7.6 Hz), 7.66 (d, 4H, J = 7.65 Hz), 13.9 (br, 2H, 2OH); 13C NMR (DMSO-d 6) δ 12.0, 33.5, 121.3, 126.4, 126.5, 127.7, 128.7, 129.5, 137.4, 142.5, 146.7. Anal. Calc. for C27H24N4O2 (436.51): C, 74.29; H, 5.54; N, 12.84. Found: C, 74.32; H, 5.59; N, 12.86.
4,4′-((4-Methoxycyclohexa-2,4-dienyl)methylene)bis(3-methyl-1-phenyl-1H-pyrazol-5-ol) (7b)
White solid, mp 162°C [Lit. Citation32, 160–161°C]. IR (KBr) νmax. 3035, 1578 (C=C) cm−1; 1H NMR (DMSO-d 6) δ 2.46 (s, 6H, 2CH3), 3.85 (s, 3H, OCH3), 4.84 (s, 1H, CH), 7.8 (d, 2H, J = 8.55 Hz), 7.12 (d, 2H, J = 8.6 Hz), 7.20 (t, 2H, J = 7.45 Hz), 7.39 (d, 4H, J = 7.45 Hz), 7.64 (d, 4H, J = 8.0 Hz), 13.85 (br, 2H, 2OH). Anal. Calc. for C28H28N4O3 (468.55): C, 71.78; H, 6.02; N, 11.96. Found: C, 71.81; H, 6.08; N, 12.03.
4,4′-((4-Chlorocyclohexa-2,4-dienyl)methylene)bis(3-methyl-1-phenyl-1H-pyrazol-5-ol) (7c)
White solid, mp 207°C [Lit. Citation31, 208°C]. IR (KBr) νmax. 3050 (O–H), 1581 (C=C) cm−1; 1H NMR (DMSO-d 6) δ 2.30 (s, 6H, 2CH3), 4.88 (s, 1H, CH), 7.20 (d, 4H, J = 8.55 Hz), 7.28 (d, 2H, J = 8.6 Hz), 7.39 (t, 4H, J = 8.0 Hz), 7.66 (d, 4H, J = 8.0 Hz), 14.0 (br, 2H, 2OH). Anal. Calc. for C27H25ClN4O2 (472.97): C, 68.56; H, 5.33; N, 11.85. Found: C, 68.48; H, 5.29; N, 11.77.
4,4′-(Furan-2-ylmethylene)bis(3-methyl-1-phenyl-1H-pyrazol-5-ol) (7d)
White solid (%), mp 192°C [Lit. Citation33, 189−90°C]. IR (KBr) νmax. 3060, 1568 (C=C) cm−1; 1H NMR (DMSO-d 6) δ 2.24 (s, 6H, 2CH3), 4.92 (s, 1H, CH), 6.06–6.18 (m, 1H), 6.3 (d, 1H, J = 3.1 Hz), 7.20 (t, 2H, J = 7.45 Hz), 7.40 (t, 4H, J = 8.0 Hz), 7.43 (d, 1H, J = 1.7 Hz), 7.69 (d, 4H, J = 8.0 Hz), 13.8 (br, 2H, 2OH); 13C NMR (DMSO-d 6) δ 12.3, 28.5, 106.7, 111.2, 121.4, 126.0, 129.8, 142.3, 146.1, 154.9. Anal. Calc. for C25H22N4O3 (426.47): C, 70.41; H, 5.20; N, 13.14. Found: C, 70.46; H, 5.18; N, 13.20.
Conclusion
In conclusion, we have discovered LiOH·H2O as a novel dual activation catalyst for Knovenagel condensation of aryl aldehydes with malononitrle and 3-methyl-1-phenyl-1H-pyrazol-5(4H)-one Citation6, respectively, for an easy and highly efficient synthesis of arylidene malononitrile 3a–e and 4,4′-(arylmethylene)bis(3-methyl-1-phenyl-1H-pyrazol-5-ols) 7a–d. The advantages are ( i ) use of cheap and easily available catalyst, ( ii ) requirement of small amount (5 or 10 mol%) of the catalyst, ( iii ) using of water as solvent, ( iv ) short reaction times, ( v ) high product yields, and ( vi ) clean product.
References
- Tanaka , K. ; Toda , F. Solvent-Free Organic Synthesis . Chem. Rev . 2000 , 100 , 1025 1074 .
- Li , C.-J. ; Chan , T.-H. Organic Reactions in Aqueous Media ; Wiley : New York , 1997 ; 64114 .
- Anastas , P.T. and Warner , J.C. 1998 . Green Chemistry, Theory and Practice , 30 New York : Oxford University Press .
- Jones , G. Organic Reactions ; Adams , R. ; Wiley 1967 ; New York 15 , 204 599
- Lehnert , W. 1970 . Verbesserte variante der knoevenagel-kondensation mit TiCl4/THF/pyridin(I). Alkyliden- und arylidenmalonester bei 0–25°C . Tetrahedron Lett , 11 ( 54 ) : 4723 – 4724 .
- Rao , P.S. and Venkataratnam , R.V. 1991 . Zinc Chloride as A New Catalyst for Knoevenagel Condensation . Tetrahedron Lett. , 32 ( 41 ) : 5821 – 5822 .
- Sabitha , G. , Reddy , B.V.S. , Babu , R.S. and Yadav , J.S. 1998 . LiCl Catalyzed Knoevenagel Condensation: Comparative Study of Conventional Method vs Microwave Irradiation . Chem. Lett. , 27 ( 8 ) : 773 – 774 .
- Reddy , B.M. , Patil , M.K. , Rao , K.N. and Reddy , G.K. 2006 . An Easy-to-use Heterogeneous Promoted Zirconia Catalyst for Knoevenagel Condensation in Liquid Phase Under Solvent-Free Conditions . J. Mol. Catal. A: Chem. , 258 : 302 – 307 .
- Bigi , F. , Chesini , L. , Maggi , R. and Sartori , G. 1999 . Montmorillonite KSF as An Inorganic, Water Stable, and Reusable Catalyst for the Knoevenagel Synthesis of Coumarin-3-Carboxylic Acids . J. Org. Chem. , 64 ( 3 ) : 1033 – 1035 .
- Kantam , M.L. ; Ravindra , A. ; Reddy , Ch.V. ; Sreedhar , B. ; Choudary , B.M. Layered Double Hydroxides-Supported Diisopropylamide: Synthesis, Characterization and Application in Organic Reactions . Adv. Synth. Catal . 2006 , 348 4–5 , 569 578 .
- Morrison , D.W. , Forbes , D.C. and Davis , Jr J.H. 2001 . Base-Promoted Reactions in Ionic Liquid Solvents. The Knoevenagel and Robinson Annulation Reactions . Tetrahedron Lett , 42 ( 35 ) : 6053 – 6055 .
- Bigi , F. , Conforti , M.L. , Maggi , R. , Piccinno , A. and Sartori , G. 2000 . Clean Synthesis in Water: Uncatalysed Preparation of Ylidenemalononitriles . Green Chem. , 2 : 101 – 103 .
- Wang , G.-W. and Wang , B.-L. 2004 . Uncatalyzed and Solvent-Free Knoevenagel Condensation Under Microwave Irradiation or Heating Conditions . Chin. J. Org. Chem. , 24 : 85 – 87 .
- Singh , D. ; Singh , D. J. Indian Chem. Soc . 1991 , 68 , 165 – 167 .
- Londershausen , M. 1996 . Approaches to New Parasiticides . Pestic. Sci. , 48 : 269 – 292 .
- Garnovskii , A.D. , Uraev , A.I. and Minkin , V.I. 2004 . Metal Complexes from Aryl and Hetarylazo Compounds . Arkivoc , iii : 29 – 41 .
- Sivaprasad , G. , Perumal , P.T. , Prabavathy , V.R. and Mathivanan , N. 2006 . Synthesis and Anti-Microbial Activity of Pyrazolylbisindoles-Promising Anti-Fungal Compounds . Bioorg. Med. Chem. Lett. , 16 : 6302 – 6305 .
- Hamama , W.S. 2001 . Pyrazolones as versatile precursors for the synthesis of fused and binary heterocycles . Synth. Commun. , 31 ( 9 ) : 1335 – 1345 .
- Singh , D and Singh , D . 1984 . Syntheses of 1,3-disubstituted 4-arylidenepyrazoline-5-one and the keto and enol forms of 4,4-arylidenebis(1,3-disubstituted pyrazolin-5-ones . J. Chem. Eng. Data , 29 ( 3 ) : 355 – 356 .
- Pavlov , P.T. , Goleneva , A.F. , Lesnov , A.E. and Prokhorova , T.S. 1998 . Biological activity of some pyrazolone derivatives . Pharm. Chem. J. (Engl. Trans.) , 32 : 370 – 372 .
- Buzykin , B.I. and Lonshchakova , T.I. 1971 . Reaction of azines with 5-pyrazolones . Russ. Chem. Bull. , 20 ( 10 ) : 2224 – 2226 .
- Wang , W. , Wang , S.-X. , Qin , X.-Y. and Li , J.-T. 2005 . Reaction of Aldehydes and Pyrazolones in the Presence of Sodium Dodecyl Sulfate in Aqueous Media . Synth. Commun. , 35 ( 9 ) : 1263 – 1269 .
- Elinson , M.N. , Dorofeev , A.S. , Nasybullin , R.F. and Nikishin , G.I. 2008 . Facile and convenient synthesis of 4,4¢-(arylmethylene)bis(1H-pyrazol-5-ols) by electrocatalytic tandem Knoevenagel–Michael reaction . Synthesis , 12 : 1933 – 1937 .
- Basak , A. ( née Nandi ); Nayak , M.K. ; Chakraborti , A.K. Chemoselective O-methylation of Phenols Under Non-aqueous Condition . Tetrahedron Lett . 1998 , 39 , 4883 – 4886 .
- Chakraborti , A.K. ; Basak , A. (née Nandi) ; A. , Grover , V. Chemoselective Protection of Carboxylic Acid as Methyl Ester: A Practical Alternative to Diazomethane Protocol . J. Org. Chem . 1999 , 64 , 8014 8017 .
- Bhagat , S. , Sharma , R. , Sawant , D.M. , Sharma , L. and Chakraborti , A.K. 2006 . LiOH·H2O As a Novel Dual Activation Catalyst for Highly Efficient and Easy Synthesis of 1,3-Diaryl-2-Propenones by Claisen-Schmidt Condensation Under Mild Conditions . J. Mol. Catal. A , 244 : 20 – 24 .
- Zabicky , J. The Kinetics and Mechanism of Carbonyl–Methylene Condensation Reactions. Part XI. Stereochemistry of the Products . J. Chem. Soc . 1961 , 683 687 .
- An-Guo , Y. , Luo , L. , Guo-Feng , W. , Xin-Zhi , C. , Wei-Dong , Y. , Jian-Hui , C. and Kai-Yuan , Z. 2009 . Chem. Res. Chin . Univ. , 25 : 876 – 881 .
- Dai , G.Y. , Shi , D.Q. and Zhou , L.H. 1996 . Chemical. Reag. , 18 : 39
- Evdokimov , N.M. , Kireev , A.S. , Yakovenko , A.A. , Antipin , M.Y. , Magedov , I.V. and Kornienko , A. 2007 . One-step Synthesis of Heterocyclic Privileged Medicinal Scaffolds by a Multi-component Reaction of Malononitrile with Aldehydes and Thiols . J. Org. Chem. , 72 : 3443 – 3453 .
- Porai-Koshits , A.E. ; Porai-Koshits , B.A. ; Lipina , N.G. Zh. Obshch. Khim . 1956 , 26 , 872 – 877 ; Porai-Koshits, A.E.; Porai-Koshits, B.A.; Lipina, N.G. Chem. Abstr. 1956, 50, 14720f .
- Ahluwalia , V.K. ; Sharma , P. ; Goyal , B. A Facile Synthesis of 3,5-Dialkyl/Diaryl-4-Aryl-8-(4-Methylphenyl)-1,7-Diphenyl-1H,4H,7H,8H-Dipyrazolo-3,4-b:4′,3′-Epyridines . Ind. J. Chem . 1997 , 36b , 1059 – 1061 .
- Sobhani , S. , Safaei , E. , Hasaninejad , A.-R. and Rezazadeh , S. 2009 . An Eco-Friendly Procedure for The Efficient Synthesis of Bis(Indolyl)Methanes in Aqueous Media . J. Organometall. Chem. , 694 : 3027 – 3031 .