Abstract
RuCl3 anchored onto imine functionalized silica has been developed as a heterogeneous catalyst for the selective oxidation of benzylic alcohols to aldehydes using molecular oxygen as an oxidant. SiO2-RuCl3 was found to be highly stable, passes hot filtration test successfully, and could be recycled several times without significant loss of activity. The SiO2-RuCl3 was characterized by Fourier Transform Infrared Spectroscopy (FTIR), Thermogavimetric Analysis (TGA), Scanning Electron Microscope (SEM), Transmission Electron Micrographs (TEM), X-Ray Diffractometry (XRD), and Atomic Absorption Spectrophotometric Analysis (AAS).
Introduction
The selective oxidation of alcohols to carbonyl compounds is highly desirable organic transformation both in laboratory and industry Citation1–4. In recent years, attention is being paid toward the development of environmentally friendly and eco-conscious processes, thus avoiding the production of metal salt wastes Citation5–7. In this direction, oxidations using green oxidants are at the forefront. Among green oxidants, molecular oxygen has taken the lead Citation8–10. However, for activation of oxygen, transition metal catalysts are required. Recently, much effort has been made to develop heterogeneous transition metal catalysts using molecular oxygen as the only oxidant Citation11–13. Among these, covalently anchored metal catalysts Citation4, 14–21 onto solid supports are preferred because these overcome the problems associated with physisorbed metal catalysts such as leaching of the metal salt leading to poor recyclability; difficult work-up procedure; generally give low activity and selectivity; long reaction time, low conversions, and, thus, low turnover frequencies for the catalyst, making the process less practical. Covalently anchored metal catalyst possesses high temperature resistant, monolayer adsorption, recyclability, and easy separation from reaction mixture at the end of the reaction.
Ruthenium-based solid catalysts Citation22–24 have attracted more attention in alcohol oxidations because of wide substrate scope, high selectivity, mild reaction conditions, and cost-effectiveness. A number of ruthenium-based solid catalysts have been reported Citation25–29 such as Ru/Al2O3, Ru(OH)x/TiO2, Ru/Ni(OH)2, Pt-Ru/C, RuHAP-γ-Fe2O3. Very recently, Ca-doped CePO4 supported ruthenium was reported as a highly efficient catalyst for the aerobic oxidation of alcohols Citation30 . However, very few covalently anchored ruthenium catalysts have been reported Citation31–33. Keeping in view our interest in covalently anchored metal catalysts for organic synthesis Citation4, 14–16, 34, we report here covalently anchored RuCl3 onto organically modified silica [SiO2-RuCl3] as a recyclable heterogeneous catalyst for the selective aerobic oxidation of alcohols to corresponding carbonyl compounds using molecular oxygen as a green oxidant.
Results and discussion
Catalyst preparation and characterization
SiO2-RuCl3 was prepared by refluxing RuCl3 with ligand-grafted silica (imine) in dry acetone (). The characterization of SiO2-RuCl3 was done by Fourier Transform Infrared Spectroscopy (FTIR), Thermogavimetric Analysis (TGA), X-Ray Diffractometry (XRD), Scanning Electron Microscope (SEM), Transmission Electron Microscope (TEM), and Atomic Absorption Spectrophotometric Analysis (AAS) analysis.
The FTIR of 3-aminopropylsilica (AMPS) displays characteristic –CH2 stretching bands at 2028 and 2974 cm−1. After Schiff condensation, the –CH2 stretching band shifted to 2927 cm−1 and the characteristic band due to C = N appears at 1631 cm−1 which gets shifted to 1591 cm−1 due to weakening of C = N bond after complexation with RuCl3. The stability of SiO2-RuCl3 was determined by thermal analysis. The TGA curve () showed an initial weight loss of up to 99 °C which may be attributed to loss of the residual solvent and water trapped onto the surface of silica. Furthermore, weight loss occurred from 200 to 699 °C, which was attributed to the loss of organic functionality. Thus, the catalyst is stable up to 200 °C and it is safe to carry out the reaction up to 100 °C.
The powdered XRD diffraction patterns for SiO2-RuCl3 showed the peaks in the pattern which were indexed on the bases of crystallographic data for the known structure of silica (). Three major reflection patterns were found in the XRD pattern corresponding to 2θ=46°, 53°, and 61.5°. The microstructure and morphology were studied using the SEM. The surface of SiO2-RuCl3 was found to be homogenous powder with porous structure. The regular arrangement of pores can be clearly observed (). The TEM images provide a direct observation of the morphology and distribution of ruthenium with average diameter of 6 nm (a) onto SiO2-RuCl3. Furthermore, no bulk aggregation of the metal occurred, indicating that the ruthenium was finally dispersed onto the surface of silica. The amount of ruthenium supported onto silica was determined by AAS analysis. It was found that 0.0176 g of Ru per g of SiO2-RuCl3 was present.
Catalyst testing for the selective oxidation of alcohols to aldehydes
To test the activity of SiO2-RuCl3 as completely heterogeneous catalyst for the oxidation of benzylic alcohols (), 4-chlorobenzylic alcohol was selected as the test substrate. Different sets of reactions were carried out with respect to different solvents, molar ratios of catalyst and different temperatures using test substrate. First of all, oxidation with test substrate was carried out in acetonitrile, toluene and water using balloon filled with oxygen as well as under air atmosphere (). Among various solvents, acteonitrile was found to be the best one, and this may be due to the high substrate solubility and solvent polarity, thus favoring oxidation. Furthermore, using acetonitrile as solvent, it was found that without oxygen atmosphere, the reaction was very sluggish and only 30% conversion of 4-chlorobenzylic alcohol was observed. The same reaction was then carried out under oxygen atmosphere using balloon filled with oxygen and found that oxidation takes place efficiently, leading to 4-chlorobenzaldehyde in 85% isolated yield, with no traces of corresponding carboxylic acid. 80 °C was selected as the reaction temperature, since at low temperature (25 and 50 °C) the reaction was slow and at 100 °C no change in product yield was observed. Furthermore, different molar ratios of Ru in SiO2-RuCl3 were tried () and it was found that 0.2 g of SiO2-RuCl3 (3.5 mol% Ru) gave the best results. Furthermore, base was required to obtain the optimum results, and easily available and inexpensive base K2CO3 was selected. For all experiments, 1 mmol of the test substrate was used. After carrying out the above experiments, the optimized conditions selected are: 4-chlorobenzylic alcohol (1 mmol), SiO2-RuCl3 (0.2 g, 3.5 mol% Ru), K2CO3 (2 mmol), acetonitrile (5 mL), and 80 °C as the reaction temperature. Oxygen in the form of balloons was used as the oxidant.
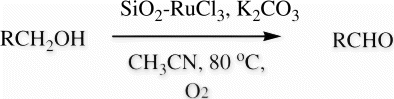
Table 1. Effect of different solvents and catalyst loading for SiO2-RuCl3 catalyzed oxidation of 4-chlorobenzylic alcohol at 80°C.
To demonstrate the generality and versatility of the procedure, benzylic alcohols substituted with different groups possessing both electron-withdrawing and electron-donating groups were subjected to oxidation under the selected conditions and excellent results were obtained (). To make the catalytic system more general, one reaction was also performed with aliphatic alcohol, n-butanol but unfortunately very low yield of the n-butanal was obtained. Hence, the developed protocol is not much successful for the aliphatic alcohols, and efforts are being made in our laboratory to improve the reaction conditions for successful oxidation of aliphatic alcohols.
Heterogeneity and recyclability
When using a supported metal catalyst, a crucial issue is the possibility that some active metal migrates from the solid support to the liquid phase and that this leached ruthenium would become responsible for a significant part of the catalytic activity. To rule out the contribution of homogenous catalysis in the results shown in , one reaction in case of 4-chlorobenzylic alcohol was carried out in the presence of SiO2-RuCl3 by stirring in acetonitrile at 80 °C using molecular oxygen, until the conversion was 25% and at that point, SiO2-RuCl3 was filtered off at the reaction temperature after 2 h. The liquid phase was then transferred to another flask containing K2CO3 and then again allowed to react, but no further significant conversion was observed. This indicates that no active species was present in the supernatant (not detectable by AAS analysis). In case of oxidation catalysis, the most important point is the deactivation and recyclability of the catalyst. To test this, a series of five consecutive runs were carried out () with the same catalyst in case of entry 3, . Thus, there is a slight change in the activity of the catalyst up to fifth use. However, the amount of Ru in SiO2-RuCl3 in the fresh and after fifth use remains the same as indicated by AAS analysis. TEM of the catalyst after fifth use (b) indicated that some aggregation has taken place, which may be the cause of slight decrease in catalytic activity.
Figure 5. Recyclability of SiO2-RuCl3. Reaction conditions: 4-chlorobenzylic alcohol (0.143 g, 1 mmol), SiO2-RuCl3 (0.2 g, 3.5 mol% Ru), K2CO3 (2 mmol), acetonitrile (5 mL) at 80 °C for 10 h.
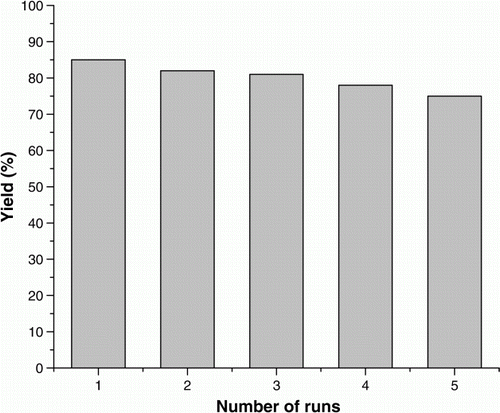
Table 2. SiO2-RuCl3 catalyzed selective oxidationa of benzylic alcohols to corresponding aldehydes by stirring in acetonitrile at 80°C using molecular oxygen as an oxidant.
A catalytic cycle has been proposed for the oxidation of benzylic alcohols using SiO2-RuCl3 in the presence of molecular oxygen (). An oxygen molecule reacts with ruthenium to form oxo complex “A,” which undergoes oxidative addition with alcohol to form an intermediate “B.” Finally, “B” undergoes reductive elimination to give aldehydes, and RuIII is regenerated with the elimination of water. The formation of oxo complex “A” from RuIII and O2 has already been reported elsewhere Citation35.
Experimental
General remarks
The chemicals used were either prepared in our laboratories or purchased from Aldrich Chemical Company and Merck. The products were characterized by comparison of their physical data with those of known samples or by their spectral data. The 1H NMR data were recorded in CDCl3 on Bruker DPX 200 (200 MHz) spectrometer using TMS as an internal standard. The IR spectra were recorded on Perkin-Elmer FTIR spectrophotometer using KBr windows, and mass spectra were recorded using Bruker Esquires 3000 (ESI). XRD spectra were recorded on Bruker AXSD8 X-ray Diffraction Spectrometer and SEM using Jeol make T-300 Scanning Electron Microscope. TEM images were recorded in a H7500 Hitachi. The amount of the ruthenium supported on silica was determined by stirring the sample in dilute HNO3 and subjecting to AAS analysis on GBC Avanta-M Atomic Absorption Spectrometer manufactured by GBC Scientific agencies.
Preparation of silica functionalized RuCl3
[SiO2-RuCl3]
Silica (K100, 0.063–0.200 mm) was activated by refluxing in a mixture of concentrated HCl and distilled water (1:1) for 10 h and then washed thoroughly with distilled water and dried at 110 °C for 5 h. Activated silica (10 g) was added to the solution of 3-aminopropyl(trimethoxy) silane (1.79 g, 10 mmol) in dry toluene (150 mL) and refluxed for 24 h. The AMPS was filtered off, washed with hot toluene (100 mL), and dried at 110 °C for 3 h to give the surface bound amino groups (AMPS). The oven dried aminopropyl silica (AMPS, 5 g) was added to sodium dried toluene (50 mL) in a round bottom flask (100 mL) followed by 2-acetylpyridine (0.605 g, 5 mmol). The reaction mixture was refluxed at 120 °C for 12 h and water formed during the reaction was removed using dean-stark apparatus. The ligand-grafted silica (imine) was filtered at the reaction temperature and washed with hot toluene thoroughly to remove unreacted 2-acetylpyridine. It was dried in an oven at 100 °C for 5 h. For the preparation of SiO2-RuCl3, a mixture of imine (2 g) and RuCl3 (0.052 g, 0.25 mmol) in dry acetone (20 mL) was stirred at room temperature for 12 h. The catalyst was filtered off, washed with acetone till the washings were colorless and dried in hot air oven at 100 °C for 5 h. To remove any physisorbed RuCl3, the catalyst was conditioned by refluxing in toluene at 110 °C (2 × 2 h), water at 100 °C (2 × 2 h), and acetonitrile at 80 °C (2 × 2 h). Finally, the conditioned catalyst was dried under vacuum for 5 h before use. The complete preparation procedure of SiO2-RuCl3 is represented in .
SiO2-RuCl3 catalyzed selective oxidation of benzylic alcohols to aldehydes
A mixture of benzylic alcohol (0.108 g, 1 mmol), SiO2-RuCl3 (0.2 g, 3.5 mol% Ru), K2CO3 (2 mmol), and acetonitrile (5 mL) in a round bottom flask (25 mL) was stirred at 80 °C using balloon filled with oxygen for 12 h (). On completion (monitored by TLC), the reaction mixture was cooled to room temperature and filtered. The residue was washed with hot ethyl acetate (20 mL) followed by distilled water (200 mL). The organic layer was washed with water and dried over anhydrous Na2SO4. Finally, the product was purified by passing through a column of silica gel and elution with EtOAc/petroleum ether.
The structures of the products were confirmed by FTIR, 1H NMR, mass spectral data, and comparison with authentic samples available commercially or prepared according to literature procedures.
Spectral data of compounds
Benzaldehyde (entry 1)
IR (υmax in cm−1, KBr): 3045 (aromatic C–H str.), 1715 (C = O str.).
1H NMR (200 MHz, CDCl3): δ 7.56–7.71 (m, 5H, Ar–H), 10.03 (s, 1H, CHO).
m/z: 106 (M+).
3,4,5-Trimethoxybenzaldehyde (entry 2)
IR (υmax in cm−1, KBr): 3045 (aromatic C–H str.), 1694 (C = O str.).
1H NMR (200 MHz, CDCl3): δ 3.89 (s, 9H, 3 × OCH3), 6.79 (s, 2H, Ar–H), 9.87 (s, 1H, CHO).
m/z: 196 (M+).
4-Chlorobenzaldehyde (entry 3)
IR (υmax in cm−1, KBr): 3040 (aromatic C–H str.), 1705 (C = O str.).
1H NMR (200 MHz, CDCl3): δ 7.5–7.56 (d, 2H, J = 7.1 Hz, Ar–H), 7.78–7.85 (d, 2H, J = 7.5 Hz, Ar–H), 9.96 (s, 1H, CHO).
m/z: 140 (M+), 142 (M + 2).
2-Bromobenzaldehyde (entry 4)
IR (υmax in cm−1, KBr): 3000 (aromatic C–H str.), 1710 (C = O str.).
1H NMR (200 MHz, CDCl3): δ 7.39–7.70 (m, 4H, Ar–H), 10.24 (s, 1H, CHO).
m/z: 185 (M+).
2-Nitrobenzaldehyde (entry 5)
IR (υmax in cm−1, KBr): 3103 (aromatic C–H str.), 1698 (C = O str.).
1H NMR (200 MHz, CDCl3): δ 7.80–8.38 (m, 4H, Ar–H), 10.24 (s, 1H, CHO).
m/z: 151 (M+).
3-Nitrobenzaldehyde (entry 6)
IR (υmax in cm−1, KBr): 3056 (aromatic C–H str.), 1708 (C = O str.), 1470 (N–O str.), 1442 (C–N str.).
1H NMR (200 MHz, CDCl3): δ 7.78 (t, 1H, Ar–H), 8.3 (d, 1H, J = 7.3 Hz, Ar–H), 8.52 (d, 1H, J = 7.7 Hz, Ar–H), 8.65 (s, 1H, Ar–H), 10.15 (s, 1H, CHO).
m/z: 151 (M+).
4-Nitrobenzaldehyde (entry 7)
IR (υmax in cm−1, KBr): 3045 (aromatic C–H str.), 1710 (C = O str.), 1475 (N–O str.) 1440 (C–N str.).
1H NMR (200 MHz, CDCl3): δ 8.12 (d, 2H, J = 7.8, Ar–H), 8.24 (d, 2H, J = 7.3 Hz, Ar–H), 10.13 (s, 1H, CHO.).
m/z: 151 (M+).
Furfural (entry 8)
IR (υmax in cm−1, KBr): 3000 (aromatic C–H str.), 1710 (C = O str.).
1H NMR (200 MHz, CDCl3): δ 6.61–7.72 (m, 3H, Ar–H), 9.61 (s, 1H, CHO).
m/z: 96 (M+).
Cinnamaldehyde (entry 9)
IR (υmax in cm−1, KBr): 3045 (aromatic C–H str.), 1690 (C = O str.).
1H NMR (200 MHz, CDCl3): δ 6.73 (d, 1H, J = 12 Hz, J = 6 Hz), 7.4–7.9 (m, 5H, Ar–H and d, 1H burried = CH), 9.68 (d, 1H, J = 6 Hz, CHO).
m/z: 132 (M+).
Pentanal (entry 10)
IR (υmax in cm−1, KBr): 2925 (aliphatic C–H str.), 1705 (C = O str.).
1H NMR (200 MHz, CDCl3): δ 0.97 (t, 3H, CH3), 1.42 (m, 2H, CH2), 1.57 (m, 2H, CH2), 2.45 (t, 2H, CH2), 9.83 (s, 1H, CHO).
m/z: 86 (M+).
Conclusion
In conclusion, we have reported a novel heterogeneous SiO2-RuCl3 for the selective oxidation of benzylic alcohols to benzaldehydes using molecular oxygen as an oxidant. The salient features of this catalyst include mild reaction conditions, high yield of products, ease of work-up, high selectivity, recyclability, and ecologically clean procedure.
Acknowledgements
We are grateful to SAIF, Punjab University, Chandigarh, for SEM, TEM, and XRD and Head, Institute Instrumentation Centre, Indian Institute of Technology, Roorkee, for TGA. Financial support from DST, New Delhi, Government of India (FIST) and UGC, New Delhi (SAP), is highly acknowledged.
References
- Hajimohammadi , M. ; Safari , N. ; Mofakham , H. ; Deyhimi , F. Green Chem . 2011 , 13 , 991 – 997 .
- Ni , J. ; Yu , W.J. ; He , L. ; Sun , H. ; Cao , Y. ; He , H.Y. ; Fan , K.N. Green Chem . 2009 , 11 , 756 – 759 .
- Jamwal , N. ; Gupta , M. ; Paul , S. Green Chem . 2008 , 10 , 999 – 1003 .
- Choudhary , D. ; Paul , S. ; Gupta , R. ; Clark , J.H. Green Chem . 2006 , 8 , 479 .
- Manyar , H.G. ; Chaure , G.S. ; Kumar , A. Green Chem . 2006 , 8 , 344 – 348 .
- Thakur , K.G. ; Ganapathy , D. ; Sekar , G. Chem. Commun . 2011 , 47 , 5076 – 5078 .
- Najafpour , M.M. Dalton Trans . 2011 , 40 , 3805 – 3807 .
- Mitsudome , T. ; Umetani , T. ; Kaneda , K. Tetrahedron Lett . 2006 , 47 , 1425 .
- Hasan , K. ; Brown , N. ; Kozak , C.M. Green Chem . 2011 , 13 , 1230 – 1237 .
- Kuang , Y. ; Nabae , Y. ; Hayakawa , T. ; Kakimoto , M. Green Chem . 2011 , 13 , 1659 – 1663 .
- Dressen , M.H.C.L. ; Stumpel , J.E. ; van de Kruijs , B.H.P. ; Meuldijk , J. ; Vekemans , J.A.J.M. ; Hulshof , L.A. Green Chem . 2009 , 11 , 60 – 64 .
- Feng , B. ; Hua , L. ; Hou , Z. ; Yang , H. ; Hu , Y. ; Li , H. ; Zhao , X. Catal. Commun . 2009 , 10 , 1542 – 1546 .
- Chen , Y. ; Bai , L. ; Zhou , C. ; Lee , J.M. ; Yang , Y. Chem. Commun . 2011 , 47 , 6452 – 6454 .
- Paul , S. ; Clark , J.H. Green Chem . 2003 , 5 , 635 – 638 .
- Shamim , T. ; Gupta , M. ; Paul , S. J. Mol. Catal. A: Chem . 2009 , 302 , 15 – 19 .
- Shamim , T. ; Choudhary , D. ; Mahajan , S. ; Gupta , R. ; Paul , S. Catal. Commun . 2007 , 10 , 1931 – 1935 .
- Sujandi ; Park , S.E. Res. Chem. Intermed . 2008 , 34 , 871 – 880 .
- Fan , Q.H. ; Li , Y.M. ; Chan , A.S.C. Chem. Rev . 2002 , 102 , 3385 – 3466 .
- Yin , L. ; Liebscher , J. Chem. Rev . 2007 , 107 , 133 – 175 .
- Trindade , A.F. ; Gois , P.M.P. ; Afonso , C.A.M. Chem. Rev . 2009 , 109 , 418 – 514 .
- Molnar , A. Chem. Rev . 2011 , 111 , 2251 – 2320 .
- Korotchenko , V.N. ; Severin , K. ; Gagn′e , M.R. Org. Biomol. Chem . 2008 , 6 , 1961 – 1965 .
- Mori , S. ; Takubo , M. ; Makida , K. ; Yanase , T. ; Aoyagi , S. ; Maegawa , T. ; Monguchi , Y. ; Sajiki , H. Chem. Commun . 2009 , 5159 – 5161 .
- Hu , Z. ; Leung , C.F. ; Tsang , Y.K. ; Du , H. ; Liang , H. ; Qiu , Y. ; Lau , T.C. New J. Chem . 2011 , 35 , 149 – 155 .
- Berman , A. ; Karn , R.K. ; Epstein , M. Green Chem . 2007 , 9 , 626 – 631 .
- Yamaguchi , K. ; Kim , J.W. ; He , J. ; Mizuno , N. J. Catal . 2009 , 268 , 343 – 349 .
- Venkatesan , S. ; Kumar , A.S. ; Lee , J.F. ; Chan , T.S. ; Zen , J.M. Chem. Commun . 2009 , 1912 – 1914 .
- Sen , S. ; Sen , F. ; Gökağaç , G. Phys. Chem. Chem. Phys . 2011 , 13 , 6784 – 6792 .
- Mori , K. ; Kanai , S. ; Hara , T. ; Mizugaki , T. ; Ebitani , K. ; Jitsukawa , K. ; Kaneda , K. Chem. Mater . 2007 , 19 , 1249 – 1256 .
- Zhang , Y. ; Wang , J. ; Zhang , T. Chem. Commun . 2011 , 47 , 5307 – 5309 .
- Sahoo , S. ; Kumar , P. ; Lefebvre , F. ; Halligudi , S.B. J. Mol. Catal. A: Chem . 2007 , 273 , 102 – 108 .
- Zotto , A.D. ; Greco , C. ; Baratta , W. ; Siega , K. ; Rigo , P. Eur. J. Inorg. Chem . 2007 , 18 , 2909 – 2916 .
- Kobayashi , S. ; Miyamura , H. ; Akiyama , R. ; Ishida , T. J. Am. Chem. Soc . 2005 , 127 , 9251 – 9254 .
- Hamad , F.B. ; Mubofu , E.B. ; Makame , Y.M.M. Catal. Sci. Technol . 2011 , 1 , 444 – 452 .
- Kannan , S ; Ramesh , R. Polyhedron 2006 , 25 , 3095 – 3103 .
- Aldrich Advancing Science Handbook of Fine Chemicals , 2007 .