Abstract
Sulfonic acid-functionalized silica-coated nano-Fe3O4particles (Fe3O4@SiO2–SO3H) serve as a novel heterogeneous catalyst for the synthesis of acylals by direct condensation of aldehydes with acetic anhydride under solvent-free conditions at room temperature in short reaction times and excellent yields. The catalyst could be easily separated from the final product using an external magnet and reused several times without consequential loss of reactivity.
1. Introduction
One of the major challenging problems during organic reactions is selective protection of carbonyl functionality from unwanted attacks. Among different methods, formation of 1,1-diacetates (acylals) from aldehydes is the most versatile reaction in modern organic synthesis. Indeed, acylals are an important building block and a useful protective group (Citation1) that have attracted considerable attention in the past decade because of their remarkable stability in neutral as well as basic reaction media (Citation2–Citation5) and as an aldehyde protection group alternative to acetals (Citation6).
Recently in viewpoint of green chemistry, several heterogeneous catalysts including poly (4-vinylpyridinium)hydrogen sulfate (Citation7), HClO4–SiO2 (Citation8), titanium(IV)salophen (Citation9), N-sulfonic acid poly(4-vinylpyridinum) chloride (Citation10), polystyrene-supported TiCl4 (Citation11), MCM-41–SO3H (Citation12), propylsulfonic acid-based nanosilica (Citation13), NaHSO4–SiO2 (Citation14), P2O5/Al2O3 (Citation15), zirconium sulfophenyl phosphonate (Citation16), zeolites (Citation17), H3PW12O40-supported MCM-41 (Citation18), and KF/Al2O3 (Citation19) have been employed to catalyze the synthesis of acylals from aldehydes. Although these methods are quite satisfactory for the preparation of these compounds, some of these procedures suffer from an expensive reagent and problem of recovery of catalyst, either difficulty in preparation of catalyst is incurred. Therefore, any effort for finding a novel and cheap catalyst for the synthesis of acylals with higher efficiency, better yield, environmentally more friendly might be of high potential and interest.
Nowadays study of nano-scale materials is gaining importance in modern organic chemistry due to its exclusive properties such as easy and efficient separation from the final product using an external magnet (Citation20), high level of catalytic activity (Citation21, Citation22), simple filtration, reusable, low cost, non-toxic, and insolubility in reaction media (Citation23).
We recently prepared nano-Fe3O4-encapsulated silica particles bearing sulfonic acid groups in our laboratory and reported the synthesis of pyrimido[4,5-b] quinolines, indeno-fused pyrido[2,3-d]pyrimidines, and tetraketones in the presence of catalytic amount of it (Citation24, Citation25). As a part of our continuing activities in application of this nano-magnetic catalyst, in the present study, we now report the performance and recyclability behavior of this nano-magnetic catalyst in the synthesis of acylals from aldehydes under solvent-free conditions. To the best of our knowledge, only one report about the protection of aldehydes in the presence of nano-magnetic catalyst is addressed (Citation26).
2. Results and discussion
To standardize the reaction conditions, a series of experiments were performed with variation of solvent and the amount of Fe3O4@SiO2–SO3H as a catalyst for a representative reaction of 4-nitrobenzaldehde (1 mmol) and acetic anhydride (5 mmol) at room temperature (). In addition, in due course owing to the importance of green chemistry, we attempted this transformation in the absence of a solvent. As illustrated in , the best yield of respective 1,1-diacetate attends after 25 minutes with 0.05 g catalyst under solvent-free condition at room temperature. The reaction was also checked in the presence of bulk Fe3O4 and nano-Fe3O4 as the catalyst in the above reaction condition. The desired product was obtained in trace and 42% yield, respectively (, entries 2 and 3). This is probably due to higher the catalyst surface in the nano rather than bulk. Also, in the presence of Fe3O4@SiO2, no improvement in yield was observed (, entry 4). Thus, Fe3O4@SiO2–SO3H was necessary as Brønsted acid to obtain the desired product.
Table 1. Optimization of the model reaction.Footnotea
Therefore, we employed the optimized condition (0.05 g nano-magnetic catalyst and solvent-free condition) for the conversion of aldehydes into the corresponding acylals at room temperature.
Scope and generality of this protocol were demonstrated by subjecting a broad range of aldehydes carrying either electron-donating or electron-withdrawing substituent. All the arylaldehydes reacted very well, giving excellent yields of the desired products under optimized reaction conditions, except in the case of aliphatic aldehyde (, entry 15), which provide the desired product in low yield and long reaction time. Results are highlighted in .
Table 2. Fe3O4@SiO2–SO3H-catalyzed synthesis of various acylals derivatives.
The structural variations in the aldehydes had no significant effect on the yield, and only the aldehyde with powerful electron donating group (, entry 17) was not converted to the corresponding acylal, that is in accordance with the literature report (Citation27). The reaction proceeded comparatively faster with aldehyde containing p-methoxy group and required only 15 min to give the corresponding acylal in a very excellent yield (95%). Longer reaction times were needed for the reaction of 2-substituted aldehydes. Vanillin could afford the target product in a high yield, but higher temperature (at 110°C) was needed.
To evaluate the chemoselectivity of this methodology, a competitive reaction for acylation of benzaldehyde in the presence of acetophenone was investigated. Under the employed condition, no acylation was observed for acetophenone.
We examined the recyclability of Fe3O4@SiO2–SO3H. After completion of the reaction of benzaldehyde and dimedone, EtOAc was added to the reaction mixture and the catalyst was recovered simply by applying an external magnet. Then the recovered catalyst was first washed with diethyl ether and then with methanol, and dried at 60°C for 1 h. The recovered catalyst was added to fresh model reaction mixture under same condition. The catalyst showed no substantial reduction in activity even after five runs. Most of the reactions were completed in 20–35 min and afforded yields of 90–98%.
The transmission electron microscopy (TEM) image of the used catalyst showed that size and shape of the catalyst particles remained almost the same after five runs () (Citation24). Furthermore, the intensity and peak positions between the used and fresh catalyst in the Fourier transform infrared (FTIR) spectra after the reaction indicated that structure of the catalyst had remained the same as that of the fresh catalyst ().
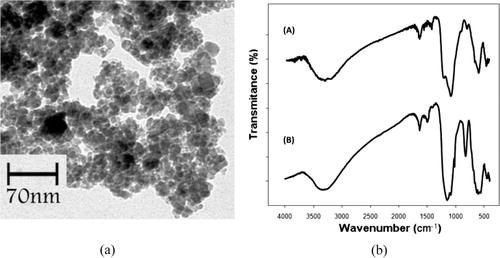
The X-ray powder diffraction (XRD) pattern of a reused catalyst () exhibits a broad peak at 2θ=25.5, which is typical for amorphous silica (Citation28). Some distinct peaks at 2θ values of 30.24, 35.60, 43.24, 53.7, 57.6, and 62.8 assigned to the (2 2 0), (3 1 1), (4 0 0), (4 2 2), (5 1 1), and (4 4 0) planes of Fe3O4 (Citation29).
According to the literature report (Citation11), we proposed a possible mechanism for the synthesis of acylals in the presence of Fe3O4@SiO2–SO3H, as shown in .
compares the efficiency of our catalyst for the synthesis of acylals with some other heterogeneous catalysts. Each of these methods has their own advantages, but using magnetically separable catalyst in this protocol could be making it green and economical than the other methods.
Table 3. Comparison of result using Fe3O4@SiO2–SO3H with results obtained by other published works for the synthesis of phenylmethylene diacetate.
3. Conclusion
In summary, we report an effective, green, and mild method for acetylation of aldehydes with acetic anhydride, which was catalyzed by Fe3O4@SiO2–SO3H. Operational simplicity, high yields, short reaction time, easy separation and reusability of catalyst and chemoselectivity are the key features of this methodology. This catalyst therefore has a great potential for use in green processes. Further investigation on the new application of Fe3O4@SiO2–SO3H as a Brønsted acidic nano-magnetic catalyst is extremely ongoing in our laboratory.
4. Experimental
Chemicals were purchased from the Fluka, Merck, and Aldrich chemical companies. Melting points were determined on Electrothermal 9100 without further corrections. Thin layer chromatography (TLC) on commercial aluminum-backed plates of silica gel 60 F254 was used to monitor the progress of reactions. Infrared spectra were recorded on a Shimadzu 8400s spectrometer with KBr plates. Only representative absorptions are given. NMR spectra were recorded in CDCl3 on Bruker Avance3-400 MHz instrument at 20–25°C. Elemental analyses were performed by Perkin-Elmer CHN analyzer, 2400 series II.
4.1. Preparation of catalyst
The synthesis of Fe3O4@SiO2–SO3H was carried in accordance with our earlier report (Citation24).
4.2. General procedure for the synthesis of acylals derivatives
In a 25-mL flask, a mixture of aldehyde (1 mmol), freshly excess distilled acetic anhydride (5 mmol), and Fe3O4@SiO2–SO3H (0.05 g, 0.016 mmol H+) (Citation24) was stirred at room temperature under solvent-free conditions for an appropriate time (). After completion of the reaction as monitored by TLC, EtOAc (10 mL) was added to the reaction mixture and stirred for 10 min. Then the catalyst was removed using the external magnet. The organic solution was washed with aqueous 10% NaHCO3 solution (2 × 10 mL) and water (10 mL), and then dried over anhydrous Na2SO4. The organic solvent was evaporated on a rotary evaporator under reduced pressure to give pure acylals. Some of the products with lower purity were further purified using flash column chromatography (eluent:ethylacetate/hexane, 1:8).
The removed catalyst was washed exhaustively with diethyl ether and methanol and dried at 60°C for 1 h prior to reuse in subsequent reactions. All the desired products were characterized by comparison of their physical data with those reported compounds.
4.3. The spectral data of selected compounds
6a (Citation32): IR (KBr, cm−1): υmax 3014, 1762, 1240; 1H NMR (400 MHz, CDCl3): δ 2.15 (s, 6H), 7.69–7.73 (m, 3H), 8.26–8.28 (m, 2H).
13a: IR (KBr, cm−1): υmax 3020, 1751, 1211; 1H NMR (400 MHz, CDCl3): δ 2.48 (s, 3H), 2.51 (s, 6H), 3.90 (s, 3H), 7.25 (s, 1H), 7.46–7.64 (m, 2H); 13C NMR (100 MHz, CDCl3): δ 20.5, 20.8, 56.1, 105.6, 116.4, 120.1, 122.9, 138.2, 139.6, 158.2, 169.0, 170.5. Anal. Calcd. for C14H16O7: C 56.75, H 5.40; found: C 57.09, H 5.11.
14a (Citation32): IR (KBr, cm−1): υmax 3014, 1751, 1211; 1H NMR (400 MHz, CDCl3): δ 2.15 (s, 6H), 7.54 (d, 2H, J = 4.4 Hz), 7.62 (d, 1H, J = 8.4 Hz), 7.85 (s, 1H), 7.85–7.90 (m, 3H), 8.00 (s, 1H).
Acknowledgments
We thank the Department of Chemistry and office of gifted student at Semnan University for their financial support.
References
- Liebermann, S.V.; Connor, R. Org. Synth. Coll. 1955, III, 441.
- Zolfigol, M.A.; Zebarjadian, M.H.; Mohammadpoor-Baltork, I.; Shamsipur, M. Synth. Commun. 2002, 32, 2803. 10.1081/SCC-120006463
- Jin, T.S.; Sun, G.; Li, Y.W.; Li, T.S. J. Chem. Res. (Synop), 2003, 30.
- Mohammadpoor-Baltork, I.; Aliyan, H. Synth. Commun. 1999, 29, 2741. 10.1080/00397919908086439
- Pinnick, H.W.; Kochhar, K.S.; Bal, B.S.; Deshpandy, R.P.; Rajadhyaksha, S.N. J. Org. Chem. 1983, 48, 1765.
- Greene, T.W. Protective Groups in Organic Synthesis, 1st ed.; Wiley: New York, 1981; p 116.
- Khaligh, N.G.; Shirini, F. Ultrason. Sonochem. 2013, 20, 19. 10.1016/j.ultsonch.2012.07.016
- Kumar, R.; Tiwari, P.; Maulik, P.R.; Misra, A.K. J. Mol. Catal. A: Chem. 2006, 247, 27. 10.1016/j.molcata.2005.11.019
- Yadegari, M.; Moghadam, M.; Tangestaninejad, Sh.; Mirkhani, V.; Mohammadpoor-Baltork, I.; Inorg. Chimica Acta 2012, 388, 102. 10.1016/j.ica.2012.02.008
- Shirini, F.; Goli Jolodar, O. J. Mol. Catal. A: Chem. 2012, 356, 61. 10.1016/j.molcata.2012.01.002
- Rahmatpour, A.; Mohammadian, S. C. R. Chimie 2013, 16, 912. 10.1016/j.crci.2013.01.012
- Tourani, H.; Naimi-Jamal, M.R.; Dekamin, M.Gh.; Amirnejad, M. C. R. Chimie 2012, 15, 1072. 10.1016/j.crci.2012.06.014
- Zareyee, D.; Moosavi, S.M.; Alaminezhad, A. J. Mol. Catal. A: Chem. 2013, 378, 227. 10.1016/j.molcata.2013.07.001
- Kannasani, R.K.; Peruri, V.V.S.; Battula, S.R. Chem. Central J. 2012, 6,136. 10.1186/1752-153X-6-136
- Hajipour, A.R.; Zarei, A.; Ruoho, A.E. Tetrahedron Lett. 2007, 48, 2881. 10.1016/j.tetlet.2007.02.090
- Curini, M.; Epifano, F.; Marcotullio, M.C.; Rosati, O.; Nocchetti, M. Tetrahedron Lett. 2002, 43, 2709. 10.1016/S0040-4039(02)00369-6
- Gigante, B.; Pereira, C.; Marcelo-Curto, M.J.; Carreyre, H.; Perot, G.; Guisnet, M. Synthesis 1995, 9, 1077.
- Jermy, B.R.; Pandurangan, A. Catal. Commun. 2008, 9, 577. 10.1016/j.catcom.2007.02.016
- Zare, A.; Hasaninejad, A.; Beyzavi, M.H.; Moosavi Zare, A.R.; Khalafi-Nezhad, A.; Asadi, F.; Baramaki, L.; Jomhori-Angali, S.; Ghaleh-Golabi, R. Synth. Commun. 2009, 39, 139. 10.1080/00397910802369711
- Koukabi, N.; Kolvari, E.; Khazaei, A.; Zolfigol, M.A.; Shirmardi-Shaghasemi, B.; Khavas, H.R. Chem. Commun. 2011, 47, 9230. 10.1039/c1cc12693h
- Schatz, A.; Reiser, O.; Stark, W.J. Chem. Eur. J. 2010, 16, 8950. 10.1002/chem.200903462
- Yan, N.; Xiao, C.X.; Kou, Y. Coord. Chem. Rev. 2010, 254, 1179. 10.1016/j.ccr.2010.02.015
- Nasir Baig, R.B.; Varma, R.S. Green Chem. 2012, 14, 625. 10.1039/c2gc16301b
- Nemati, F.; Heravi, M.M.; Saeedi rad, R. Chin. J. Catal. 2012, 33, 1825. 10.1016/S1872-2067(11)60455-5
- Nemati, F.; Saeedirad, R. Chin. Chem. Lett. 2013, 24, 370. 10.1016/j.cclet.2013.02.018
- Esmaeilpour, M.; Sardarian, A.R.; Javidi, J. Appl. Catal. A: Gen. 2012, 445–446, 359.
- Jin, T.Sh.; Zhao, Y.; Gu, Sh.Q.; Liu, L.B.; Li, T.Sh. Indian J. Chem. 2006, 45B, 1054.
- Chen, Z.; Xue, Z.; Chen, L.; Geng, Z.; Yang, R.; Chen, L.; Wang, Z. New. J. Chem. 2013, 37, 3731. 10.1039/c3nj00490b
- Sun, Y.; Duan, L.; Guo, Z.; Duan Mu, Y.; Ma, M.; Xu, L.; Zhang, Y.; Gu, N. J. Magn. Mater. 2005, 285, 65. 10.1016/j.jmmm.2004.07.016
- Kang, L.Q.; Cai, Y.Q.; Cheng, L. Monatsh Chem. 2013, 144, 247. 10.1007/s00706-012-0777-6
- Jin, T.S.; Sun, G.; Li, Y.W.; Li, T.S. Green Chem. 2002, 4, 255. 10.1039/b200219a
- Khan, A.T.; Choudhury, L.H.; Ghosh, S. J. Mol. Catal. A: Chem. 2006, 255, 230. 10.1016/j.molcata.2006.04.008
- Nagy, N.M.; Jakab, M.A.; Konya, J.; Antus, S. Appl. Clay Sci. 2002, 21, 213. 10.1016/S0169-1317(02)00066-2
- Yin, L.; Zhang, Z.H.; Wang, Y.M.; Pang, M.L. Synlett 2004, 10, 1727.
- Saini, A.; Kumar, S.; Sandhu, J.S. Synth. Commun. 2007, 38, 106. 10.1080/00397910701650831
- Jermy, B.R.; Pandurangan, A. Catal. Commun. 2008, 9, 577. 10.1016/j.catcom.2007.02.016
- Freeman, F.; Karcherski, E.M. J. Chem. Eng. Data 1977, 22, 355. 10.1021/je60074a038