Abstract
A new corrosion inhibitor, namely acid extract of leaves of Hibiscus sabdariffa, has been synthesized, and its inhibiting action on the corrosion of mild steel in acidic bath (1.2 N HCl and 1.2 N H2SO4) has been investigated by corrosion-monitoring techniques. The results of the present study show that this compound has decent inhibiting property for mild steel corrosion in 1.2 N H2SO4 than 1.2 N HCl. Four sorption isotherms are tested for the data, namely Langmuir, Frumkin, Florry–Huggins, and Langmuir–Freundlich isotherms; of these the Langmuir isotherm fits the data well having correlation coefficient over 0.99 in both the acid environments.
Introduction
Metals are affected by different forms of corrosion in various environments. The exposures can be most severe, but in many cases the corrosion may be controlled by means of inhibitors (Citation1, Citation2). Naturally occurring compounds from bio-materials can be interface or interphase inhibitors in acid solution (Citation1). Interface inhibitors function primarily by retarding surface reactions through direct adsorption, while interphase inhibitors, after an initial adsorption, alter the metal surface reactivity through the formation of an extended phase at the metal-solution interphase. Most of the well-known acid inhibitors are organic compounds containing nitrogen, sulfur, oxygen, phosphorous, alkaloids, proteins, carbohydrates, and aromatic ring or triple bonds. It has been reported (Citation3–Citation8) that the inhibition efficiency decreases in the order: O < N < S < P. A broad range of corrosion inhibitors is used for mild steel under various conditions (Citation2, Citation4–Citation9). Organic chemicals commonly used against uniform corrosion have a little effect on pitting (Citation10). Moreover, some of the organic inhibitors like benzotriazole (BTA) and its derivatives are excellent corrosion inhibitors for mild steel and its alloys in a wide range of media, but the disadvantage of BTA is its toxicity. The use of BTA is nowadays quite limited, as toxic inhibitors that are widely used in industrial processes should be replaced with new environmentally friendly inhibitors to be in line with environmental protection requirements. Biomaterials and their derivatives have received a lot of interest from the researchers due to their commercial importance, cheap availability, less toxic, and some of them with antifungal activity (Citation11). The present work is aimed at making a bio-compound, namely acid extracts of leaves of Hibiscus sabdariffa (AELHS), which is easily biodegradable, and to test inhibition efficiency against mild steel corrosion in acid environments, and fit the data with different sorption isotherms.
Results and discussion
Solution composition
The composition of the AELHS is shown in (Citation12, Citation13).
Table 1. The % composition of AELHS.
Effect of immersion period on inhibition efficiency
The inhibition efficiency is calculated from the weight loss experiments performed for mild steel in 1.2 N HCl and 1.2 N H2SO4 for 5 g inhibitor concentration per 100 mL of corrosive medium and at temperature 30 ± 1°C. It is evident from that the inhibitor efficiency of AELHS on mild steel increases for first three hours and then decreases thereafter in the case of HCl. The reason behind this increase in inhibition efficiency initially may be attributed to the formation of a barrier film. But, the weight loss experiments of mild steel in 1.2 N H2SO4 for 5 g inhibitor concentration per 100 mL of corrosive medium and at temperature 30 ± 1°C are slightly different from HCl as the inhibition efficiency decreases continuously with time and stabilizes at a value of 66.67% after 24 hours.
Effect of inhibitor concentration on inhibition efficiency
From the inhibition efficiencies obtained from the weight loss experiments for 1.2 N HCl and 1.2 N H2SO4, it is found that the inhibition efficiency increases with increase in inhibitor concentration for both acidic media, which is evident from . Values of inhibition efficiency of AELHS for various time periods and concentrations are shown in and . The increase in efficiency of the inhibitor with increase in concentration may be attributed to increase in number of molecules occupied by the inhibitor on the steel–acid solution interface. As the number of molecules increases, the corrosion reactions are prevented from occurring over the active sites of the mild steel surface covered by adsorbed inhibitor species, whereas the corrosion takes place on the surface not covered by the inhibitor molecules. Thereby, one may conclude that the greater the surface coverage the greater the inhibition efficiency. This assumption has been applied to deduce the effect of concentration on the adsorption of inhibitors. This is in total agreement with the results of several investigators (Citation11, Citation14).
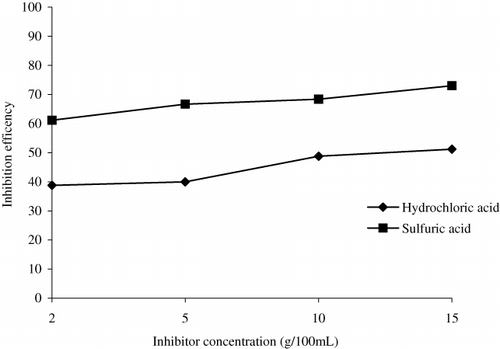
Table 2. Inhibition efficiency of mild steel in 1.2 N HCl with AELHS for various time intervals.
Table 3. Inhibition efficiency of mild steel in 1.2 N H2SO4 with AELHS for various time intervals.
Effect of inhibitor concentration on corrosion rate
In the present case, corrosion rate can be defined as the weight loss per unit area and unit time. To assess its protection value, AELHS is added in 2, 5, 10, and 15 g/100 mL concentration in corrodent (1.2 N HCl and H2SO4) at 30 ± 1°C. shows plot of corrosion rate of mild steel specimens as a function of concentration of the inhibitor. It is evident from the results, say for the exposure period of three hours reported, that corrosion rate decreases with increase in concentration. It is assumed that as the concentration of inhibitor increases, the number of inhibitor molecules occupying the active site increases, thereby offering resistance to the activity of acid (i.e. decreases the corrosion rate). The basis of assumption may be explained, according to Bockris and Drazic (Citation15), by the inhibition mechanism between Fe and inhibitor, as given below:
Effect of acid concentration on inhibition efficiency of AELHS on mild steel
It is evident from that with increase in acid concentration (i.e. 1 N, 1.2 N, 1.5 N), the inhibition efficiency of 2 g per 100 mL of AELHS for a period of three hours at a temperature of 30 ± 1°C decreases slightly. The spontaneous corrosion of mild steel in acidic solutions may be represented by the anodic dissolution reaction:
Adsorption isotherms
Basic information on the interaction between the inhibitors and the mild steel surface (Citation18) and mechanism of electrochemical reaction (Citation19) may be provided by the adsorption isotherm. The surface coverage of different concentrations of inhibitor in acidic media has been evaluated from weight loss measurements and attempted to fit these values to different sorption isotherms.
Langmuir isotherm
According to the Langmuir isotherm (Citation20, Citation21), θ is related to the inhibitor concentration C i (g/100 mL) given by the following relation (Citation22):
Frumkin isotherm
The Frumkin isotherm (Citation25) (shown in ) helps to evaluate the interactions of adsorbed molecule on the mild steel surface. The equation of Frumkin isotherm is given by:
Table 4. Adsorption coefficients of corrosion inhibitor on mild steel in acid media based on different adsorption isotherm models.
Florry–Huggins isotherm
The substitution of water molecules by inhibitor forms the basis of assumption in Florry–Huggins isotherm (Citation27). The equation of Florry–Huggins isotherm (shown in ) is given by:
Langmuir–Freundlich isotherm
The Langmuir–Freundlich isotherm (Citation28) (shown in ) is given by the equation:
Specific reaction rate
The rate equation that correlates specific reaction rate (k) with corrosion rate (r) is given by Mathur and Vasudevan (Citation29) for the corrosion rate of iron against the molar concentration (C) of H3PO4, and obtained straight-line relationship, whose slope decreased sharply at approximately 1.5 M H3PO4. The following rate equation is set up to describe straight-line relationship:
Effect of acid solution
It has been evident from that AELHS inhibits corrosion of mild steel in 1.2 N H2SO4 much better than in 1.2 N HCl environment for a time period of three hours at all inhibitor concentrations. The reason for the difference in inhibiting efficiency may be due to the competition between the inhibitor molecules and the anions (of HCl and H2SO4) to get adsorbed onto the surface of mild steel. This competition may lead to a random arrangement of the adsorbed inhibitor molecules and hence there is variation in inhibition efficiency of AELHS of mild steel in HCl and H2SO4. In HCl, the decrease in efficiency of the inhibitor may be due to the basic species in the inhibitor which might have got protonated and these cationic species might have been adsorbed electrostatically with Cl− ions on the mild steel surface by vertical adsorption (Citation31, Citation32). Similarly, the higher ionization of basic species of inhibitor in sulfuric acid may be the reason of these compounds exhibiting higher inhibition efficiencies in sulfuric acid as compared to hydrochloric acid, because of increased ionization. Moreover, the adsorption of inhibitor on anodic sites through lone pair of electrons on sulfuric ions () reduces the anodic dissociation of mild steel may be the cause for better inhibiting properties of AELHS in sulfuric acid than in hydrochloric acid (Citation18).
Table 5. Results of corrosion rate, weight loss, inhibition efficiency, surface coverage of mild steel in acid environment (HCl and H2SO4) for a period of three hours and for the area exposed for corrosion (Area = 18.25 cm2).
Experimental
Synthesis of inhibitor AELHS
The inhibitor selected for the study is the leaves of fresh H. sabdariffa. A large amount of leaves of H. sabdariffa are collected from the local area. The leaves are washed with deionized water and then dried in oven at 80 ± 1°C for a period of one hour. A 15 g of dried leaves is taken and to that 10 mL each of H2SO4, HCl, and HNO3 are added, along with this 300 mL of double-distilled water is added (Citation33). The mixture is boiled for a period of 30 minutes and then kept aside for 16 hours. The resultant mixture is filtered and the filtered solution (AELHS) is directly used for experimental purpose. The schematic of the experimental setup and the flowchart of inhibitor preparation process are shown in and , respectively.
The chemical constituents that are likely to be present in the leaves of H. sabdariffa are α-terpinylacetate, anisaldehyde, β-carotene, β-sitosterol, β-D-galactoside, β-sitosteryl benzoate, niacin, fat, isoamyl alcohol, iso-propyl alcohol, methanol, 3-methyl-1-butanol, benzyl alcohol, ethanol, malic acid, fiber, and ash (Citation34). It is important to note that the information on the level of extraction of these chemicals from leaves by acids is not available in the literature.
Specimen
Rectangular specimens of mild steel of dimensions 4.5 cm × 2.5 cm × 0.5 cm are employed for the determination of weight loss measurements. The chemical composition of test specimens used for the experiments is shown in . Prior to immersion, specimens are cleaned by buffing to produce a mirror finish with the help of a sandpaper and degreased with acetone and dried in atmospheric air for about 15 minutes. After each and every test, specimens are cleaned with 2 N NaOH to remove any corrosion products. The specimen is then cleaned with acetone and stored in moisture-free desiccators (Citation35).
Table 6. The composition of mild steel used in the study.
Solution
The aggressive solution used is made of analytical reagent (AR)-grade HCl, H2SO4, and HNO3 (Merck). Appropriate concentration of acid is prepared using double-distilled water. Concentration range of inhibitor used for experimentation is between 2 and 15 g per 100 mL of aggressive media.
Weight loss study
The solutions of 1 N, 1.2 N, 1.5 N HCl, and H2SO4 each are prepared by mixing the acid in deionized double-distilled water. All the batch corrosion studies are carried out using 100 mL of appropriate corrosion medium. Once the specimen is prepared, the initial weight of the specimen is measured. Each reading reported is an average of three experimental readings recorded to nearest of 0.0001 g on a weighing machine. Requisite quantity of inhibitor is added to 250 mL plastic beakers containing 100 mL of synthetic corrosion medium and mild steel (Citation33). The bottles are maintained at temperature 30 ± 1°C for all experiments. The contact time in the batch is varied from 1 to 24 hours (i.e. 1, 2, 3, 4, 16, and 24 hours) for a particular concentration of inhibitor. Further, experiments are conducted for the same time period by varying the concentration of inhibitor. At the end of each test, the weight loss of the specimen is measured using Mettler-weighing machine. A corrosion inhibitor is a chemical substance which decreases the corrosion rate when added in small concentration. The corrosion efficiency of that inhibitor is thus expressed by a measure of this improvement.
The percentage inhibition efficiency (I) of the inhibitor is calculated (Citation36) as:
where ΔM ui and ΔM i are the weight loss of the metal in uninhibited acid and inhibited acid solution, respectively. The degree of surface coverage (θ) may be calculated (Citation11) as:
The corrosion rate (mg cm−2 h−1) may be obtained by the following equation (Citation35):
where weight loss is expressed in mg, area in cm2 of metal surface exposed, and time in hours.
Conclusions
The results clearly indicate that the AELHS is a decent corrosion inhibitor of mild steel. Out of the four sorption isotherms tested for the data, namely Langmuir, Frumkin, Florry–Huggins, and Langmuir–Freundlich isotherms, the Langmuir isotherm fits the data well having correlation coefficient over 0.99 in both the acid (HCl and H2SO4) environments. Type of acid, time, and acid concentration play a vital role in inhibition efficiency of the inhibitor. It has been observed that adsorption of inhibitor on mild steel in sulfuric acid environment is more spontaneous than in hydrochloric acid environment, leading to better inhibiting properties of inhibitor in sulfuric acid environment. Collectively, it may be concluded that the AELHS inhibitor works much better in sulfuric acid environment than in hydrochloric acid environment.
References
- Saremi, M.; Dehghanian, C.; Sabet, M.M. The Effect of Molybdate Concentration and Hydrodynamic Effect on Mild Steel Corrosion Inhibition in Simulated Cooling Water. Corros. Sci. 2006, 48, 1404–1412. 10.1016/j.corsci.2005.06.009
- Eddy, N.O.; Odoemelam, S.A.; Odiongenyi, A.O. Inhibitive, Adsorption and Synergistic Studies on Ethanol Extract of Gnetum Africana as Green Corrosion Inhibitor for Mild Steel in H2SO4. Green Chem. Lett. Rev. 2009, 2, 111–119. 10.1080/17518250903170868
- Sharma, S.K.; Mudhoo, A.; Gargi Jain, G.; Khamis, E. Corrosion Inhibition of Neem (Azadirachta indica) Leaves Extract as a Green Corrosion Inhibitor for Zinc in H2SO4. Green Chem. Lett. Rev. 2009, 2, 47–51. 10.1080/17518250903002335
- Eddy, N.O.; Odoemelam, S.A.; Ama, I.N. Ethanol Extract of Ocimum gratissimum as a Green Corrosion Inhibitor for the Corrosion of Mild Steel in H2SO4. Green Chem. Lett. Rev. 2010, 3, 165–172. 10.1080/17518251003636428
- Eddy, N.O.; Ekwumemgbo, P.A.; Mamza, P.A.P. Ethanol Extract of Terminalia catappa as a Green Inhibitor for the Corrosion of Mild Steel in H2SO4. Green Chem. Lett. Rev. 2009, 2, 223–231. 10.1080/17518250903359941
- Eddy, N.O.; Ita, B.I.; Dodo, S.O.; Paul, E.D. Inhibitive and Adsorption Properties of Ethanol Extract of Hibiscus sabdariffa calyx for the Corrosion of Mild Steel in 0.1 M HCl. Green Chem. Lett. Rev. 2012, 5, 43–53. 10.1080/17518253.2011.578589
- Sharma, S.K.; Mudhoo, A.; Jain, G.; Sharma, J. Corrosion Inhibition and Adsorption Properties of Azadirachta indica Mature Leaves Extract as Green Inhibitor for Mild Steel in HNO3. Green Chem. Lett. Rev. 2010, 3, 7–15. 10.1080/17518250903447100
- Beenakumari, K.S. Inhibitory Effects of Murraya koenigii (Curry Leaf) Leaf Extract on the Corrosion of Mild Steel in 1 M HCl. Green Chem. Lett. Rev. 2011, 4, 117–120. 10.1080/17518253.2010.514866
- Umoren, S.A.; Eduok, U.M.; Israel, A.U.; Obot, I.B.; Solomon, M.M. Coconut Coir Dust Extract: A Novel Eco-friendly Corrosion Inhibitor for Al in HCl Solutions. Green Chem. Lett. Rev. 2012, 5, 303–313. 10.1080/17518253.2011.625980
- Abiola, O.K.; Otaigbe, J.O.E. Adsorption Behaviour of 1-phenyl-3-methylpyrazol-5-one on Mild Steel from HCI Solution. Int. J. Electrochem. Sci. 2008, 3, 191–198.
- Chowdhary, R.; Jain, T.; Rathoria, M.K.; Mathur, S.P. Corrosion Inhibition of Mild Steel by Acid Extracts of Prosopis juliflora. Bull. Electrochem. 2004, 20 (2), 67–75.
- Lowry, O.H.; Rosebrough, J.N; Farr, A.L.; Randall, R.I. Protein Measurement with Folin-phenol Reagent. J. Biol. Chem. 1951, 193, 265–275.
- Nelson, N. A Photometric Adaptation of the Somogyii Method for the Determination of Glucose. J. Biol. Chem. 1944, 153, 375–380.
- Abboud, Y.; Abourriche, A.; Saffaj, T.; Berrada, M.; Charrouf, M.; Bennamara, A.; Cherqaoui, A.; Takkyet, D. The Inhibition of Mild Steel Corrosion in Acidic Medium by 2,2’-bis(benzimidazole). Appl. Surf. Sci. 2006, 252, 8178–8184. 10.1016/j.apsusc.2005.10.060
- Bockris, J.O'M.; Drazic, D. The Kinetics of Deposition and Dissolution of Iron: Effect of Alloying Impurities. Electrochim. Acta 1962, 7, 293–313. 10.1016/0013-4686(62)87007-8
- Quraishi, M.A.; Rawat, J.; Ajmal, M. Dithiobiurets: A Novel Class of Acid Corrosion Inhibitors for Mild Steel. J. Appl. Electrochem. 2000, 30, 745–751. 10.1023/A:1004099412974
- Oguzie, E.E. Studies on the Inhibitive Effect of Occimum viridis Extract on the Acid Corrosion of Mild Steel. Mater. Chem. Phys. 2006, 99, 441–446. 10.1016/j.matchemphys.2005.11.018
- Larabi, L.; Harek, Y.; Benali, O.; Ghalem, S. Hydrazide Derivatives as Corrosion Inhibitors for Mild Steel in 1M HCl. Prog. Org. Coat. 2005, 54, 256–262. 10.1016/j.porgcoat.2005.06.015
- Jeyaprabha, C.; Sathiyanarayanan, S.; Venkatachari, G. Effect of Cerium Ions on Corrosion Inhibition of PANI for Iron in 0.5 M H2SO4. Appl. Surf. Sci. 2006, 253, 432–438. 10.1016/j.apsusc.2005.12.081
- Langmuir, I. The Constitution and Fundamental Properties of Solids and Liquids. I. Solids. J. Am. Chem. Soc. 1916, 38, 2221–2295. 10.1021/ja02268a002
- Langmuir, I. The Constitution and Fundamental Properties of Solids and Liquids. II. Liquids. J. Am. Chem. Soc. 1917, 39, 1848–1906. 10.1021/ja02254a006
- Bentiss, F.; Gassama, F.; Barbry, D.; Gengembre, L.; Vezin, H.; Lagrenee, M.; Traisnel, M. Enhanced Corrosion Resistance of Mild Steel in Molar Hydrochloric Acid Solution by 1,4-bis(2-pyridyl)-5H-pyridazino[4,5-b]indole: Electrochemical, Theoretical and XPS Studies. Appl. Surf. Sci. 2006, 252, 2684–2691. 10.1016/j.apsusc.2005.03.231
- Villamil, R.F.V.; Corio, P.; Rubin, J.C.; Agostinho, S.M.L. Effect of Sodium Dodecylsulfate on Copper Corrosion in Sulfuric Acid Media in the Absence and Presence of Benzotriazole. J. Electroanal. Chem. 1999, 472, 112–119. 10.1016/S0022-0728(99)00267-3
- Villamil, R.F.V.; Corio, P.; Rubin, J.C.; Agostinho, S.M.L. Sodium Dodecylsulfate-benzotriazole Synergistic Effect as Inhibitor of Processes on Copper Chlorideic Acid Interface. J. Electroanal. Chem. 2002, 535, 75–83. 10.1016/S0022-0728(02)01153-1
- Frumkin, A.N. A Multicomponent Isotherm for Gas Adsorption. Z. Phys. Chem. 1925, 116, 466–485.
- Kilislioglu, A. The Effect of Various Cations and pH on the Adsorption of U(VI) on Amberlite IR-118H Resin. Appl. Radiat. Isot. 2003, 58, 713–717. 10.1016/S0969-8043(03)00116-7
- Horsfall Jr, M.; Spiff, A.I. Studies on the Effect of pH on the Sorption of Pb2+ and Cd2+ Ions from Aqueous Solutions by Caladium bicolor (Wild Cocoyam) Biomass. Electronic J. Biotechnol. 2004, 7 (3), 313–323.
- Sips, R. On the Structure of a Catalyst Surface. J. Chem. Phys. 1948, 16, 490–495. 10.1063/1.1746922
- Mathur, P.B.; Vasudevan, T. Reaction Rate Studies for the Corrosion of Metals in Acid-1, Iron in Mineral Acids. Corrosion 1982, 38, 171–178. 10.5006/1.3579270
- Wang, L. Inhibition of Mild Steel Corrosion in Phosphoric Acid Solution by Triazole Derivatives. Corros. Sci. 2006, 48, 608–616. 10.1016/j.corsci.2005.02.007
- Hackerman, N.; Thompson, C.D. Effect of Nitriles on the Polarization of Iron in 1.0N HCl. Corros. Sci. 1973, 13, 317–325. 10.1016/0010-938X(73)90035-8
- Antropov, L.I. A Correlation between Kinetics of Corrosion and the Mechanism of Inhibition by Organic Compounds. Corros. Sci. 1967, 7, 607–620. 10.1016/0010-938X(67)80036-2
- Ebenso, E.E.; Ibok, U.J.; Ekpe, U.J.; Jackson, E.; Abiola, O.K.; Oforka, N.C.; Martinez, S. Corrosion Inhibition Studies of Some Plant Extracts on Aluminium in Acidic Medium. Trans. SAEST 2004, 39, 117–123.
- Mahadevan, N.; Shivali; Kamboj, P. Hibiscus Sabdariffa Linn. – An Overview. Nat. Prod. Radiance 2009, 8 (1), 77–83.
- Ekpe, U.J.; Ibok, U.J.; Ita, B.I.; Offiong, O.E.; Ebenso, E.E. Inhibitory Action of Methyl and Phenyl Thiosemicarbazone Derivatives on the Corrosion of Mild Steel in Hydrochloric Acid. Mater. Chem. Phys. 1995, 40, 87–93. 10.1016/0254-0584(94)01464-R
- Bansiwal, A.; Anthony, P.; Mathur, S.P. Inhibitive Effect of some Schiff Bases on Corrosion of Aluminium in Hydrochloric Acid Solutions. Brit. Corros. J. 2000, 35, 301–303. 10.1179/000705900101501380