Abstract
Spent engine oil, a mixture of aliphatic and aromatic compounds, is one of the frequent environmental pollutants. In this paper, biodesulfurization of spent oil using Rhodococcus sp. was studied. Batch studies were conducted varying the oil to aqueous medium ratio of 10:90 to 90:10. The results demonstrated that maximum desulfurization of 80% was obtained at the oil to aqueous phase ratio of 70:30. Kinetic parameters of Monod type growth model, namely, µmax, maximum specific growth rate and Ks, the half saturation constant were determined by varying the initial sulfur content of spent oil in the range of 0.16–1.05% (w/v). Gas chromatography–mass spectrometry was done to identify the compounds present in treated and untreated spent engine oil. The surface tension and emulsification indices of both oil and aqueous phases were also determined at different reaction times.
1. Introduction
Spent engine oil, also known as used mineral-based crankcase oil, is a mixture of aliphatic hydrocarbons (C15–C20), aromatic hydrocarbons, polychlorinated biphenyls, chlorodibenzofurans, lubricants, metal contaminants like Al, Cr, Sn, Pb, Mn, Ni, Zn, etc. generated due to the wearing of engine parts. The concentration of >C25 molecules is very high in spent engine oil (Citation1). Worldwide large volume of spent engine oil is generated during the replacement of motor oil of automobiles. Although a minor fraction of spent engine oil is regenerated following different processes (Citation2–Citation7), a large fraction is disposed off, particularly causing soil pollution. According to Montagnolli et al. (Citation8), approximately 1.1 million tons per year of spent lubricant oil is wasted in Europe. As reported by Jhanani et al. (Citation9), the used oil generation rate in India is 1296 KL/year. Therefore, there is ample scope for recycling of spent engine oil after proper processing. According to Kim et al. (Citation1), liquid fuels of two categories, namely gasoline grade (<C12) and diesel grade (>C12) are obtained through the pyrolysis of spent engine oil. Due to very stringent rules on sulfur content of diesel, the sulfur level of the feed material, i.e., spent engine oil should be very low. However, the sulfur content of spent engine oil is usually very high and lies in the range of 1–2%. Mainly the refractory sulfur compounds like 4,6-dimethyl dibenzothiophene, naphthothiophenes, etc. having high boiling points are present in lubricating oil obtained from vacuum distillation. Moreover, many sulfur compounds like zinc dithiophosphate, molybdenum disulphide, and zinc dialkyldithiophosphate, etc. are mixed with this fraction as additives to enhance the lubricity of engine oil. As a consequence, the diesel fractions obtained through pyrolysis of spent engine oil has high sulfur content. The reduction of sulfur in the spent engine oil is necessary to obtain ultra low sulfur diesel through pyrolysis. Biodesulfurization of spent oil using suitable microorganisms may serve as a promising process to eliminate the organosulfur compounds, as already studied in case of hydrodesulfurized diesel obtained from petroleum crude (Citation10–Citation24). In case of diesel, dibenzothiophene (DBT) and its alkylated homologues are biodesulfurized by the action of microorganisms like Rhodococcus sp. Pseudomonas putida, etc., to 2-hydroxybiphenyl (2-HBP) and other methylated hydroxyphenyls which are potential biosurfactants. For Rhodococcus sp., the conversion is assisted by the enzymes monooxygenases (Dsz C, Dsz A) supported by flavin reductase (Dsz D) and a desulfinase (Dsz B) via Kodama 4S pathway and the calorific value of the fuel remains unaltered. This microorganism secretes some other biosurfactants like phospholipids, rhamnolipids, glycolipids, etc. The presence of biosurfactant eases the uptake of water-immiscible hydrocarbon and sulfur nutrients by the microorganisms, suspended in aqueous medium through the reduction of interfacial tension. The extraction of biosurfactants simultaneously with the desulfurization of the waste oil is expected to make the recycling process more attractive from the perspective of environmental impact as well as financial benefit. These biosurfactants are value-added products which can be used for different industrial purposes, mainly as drilling mud in advanced oil recovery from wells (Citation25). Although a few research studies on the biodesulfurization of diesel have already been reported (Citation10–Citation24). Only a few (Citation26) have been reported on the biodesulfurization of spent engine oil are available in the literature.
The aim of this work was to study the biodesulfurization of spent engine oil using Rhodococcus sp. in batch mode and to assess the potential for the production of biosurfactant.
2. Experimental
2.1. Materials
Beef extract (E. Merck), peptone (E. Merck), NaCl (Ranbaxy), methanol (E. Merck), acetone (E. Merck), DBT (Aldrich Chemical), N2 (Prakash traders), chloroform (E. Merck), NaOH (E. Merck), pentane (E. Merck), hexadecane (E. Merck), HCl (E. Merck), isopropyl alcohol (Process Chemical Industries), diethyl ether (E. Merck), 2-HBP (Fluka), acetonitrile, Gibbs reagent (2,6-dichloroquinine-4-chloroimide; E. Merck), 1-(2-pyridylazo)-2-napthol (Aldrich Chemical) have been used during the present investigation.
2.2. Microorganism
The pure bacterial strain of Rhodococcus sp. (NCIM 2891) was purchased from National Collection of Industrial Microorganisms (NCIM), India. Cells were cultivated and enriched in a sulfur-free medium supplemented with spent oil in 50-ml Erlenmeyer flasks. This culture, adapted to spent engine oil environment, was used as stock culture for further inoculation.
2.3. Spent oil used
Spent engine oil was collected from local automobile service stations. Characteristics of spent engine oil are given in .
Table 1. Characteristics of spent engine oil.
2.4. Composition of the sulfur-free growth medium
Basis: 1 dm3
Beef extract: 10 g
NaCl (AR): 5 g
Peptone (for bacteriology): 10 g
Distilled water: 1 dm3.
3. Analytical methods
3.1. Dry weight method for the determination of bacterial mass
The biomass concentration in the reaction broth was determined by dry weight method. In this method, the broth was centrifuged at the rate of 10,000 rpm for 15 minutes at −15°C. The bacterial mass was then transferred to a pre-weighted aluminum cup and dried at 50°C overnight. The exact weight of the bacterial mass was determined by subtracting the weight of dry cup from that of the cup containing dry bacterial mass.
3.2. Sulfur analysis
X-ray fluorescence (XRF), energy dispersive X-ray fluorescence (EDXRF), gas chromatography (GC) using flame photometric detector and CHNSO (2400 Series II, PerkinElmer, USA) analysis have been followed to determine the concentration of sulfur in the waste oil samples before and after the microbial treatment.
3.3. FESEM analysis
The affinity of microbial cells for the oil–water interface was determined by visualization of maximum bacterial growth on the oil–water interface. The morphology of bacterial growth was studied under field emission scanning electron microscopy (FESEM; JEOL-JSM-6700F) after 48 hours of fermentation in the bioreactor at 28°C.
3.4. Analysis of treated and untreated spent engine oil in GC–MS
Analysis of different compounds present in original and biodesulfurized spent engine oil was done using GC–mass spectrometry (MS; Agilent Technologies model 6890N), equipped with inert mass selective detector. The gas chromatograph was fitted with a 25.0 m capillary column coated with a 0.33 ml thick film of 5% phenyl methylpolysiloxane. Helium was employed as a carrier gas at a constant flow of 1.0 ml min−1 with a pressure of 21.4 psi. Sample was prepared by diluting spent oil sample with hexadecane (1:50). The initial oven temperature of 303 K was held for 1.0 minute and then programmed from 313 K to 598 K at 5°C min−1 with an isotherm held for 40 minutes. A splitless injection was carried out for first 5 minutes. It was followed by split injection (ratio 16:1) and the injection was carried out at 523 K. The ion source and transfer line temperatures were 503 K and 598 K, respectively. Data were collected in the full-scan mode and a solvent delay of 2.0 minutes was used. The chromatographic peaks were identified with the help of the NBS 75 K.N and Wiley 7n.1 data libraries. In addition, identification was also carried out on the basis of the fragmentation observed and taking into account the retention times of the peaks using standard compounds, whenever available.
3.5. Extraction and determination of lipid content of cell-free aqueous medium
Bligh and Dyer (Citation27) procedure has been followed for lipid extraction. According to this method, chloroform (20 ml), methanol (10 ml), and 10 ml cell-free aqueous reaction broth were mixed thoroughly using a homogenizer for 5–7 minutes. The mixture was taken for centrifugation for 10 minutes. Two layers were separated with bottom layer containing lipids dissolved in chloroform. These steps were repeated for three times so that all lipid parts can be transferred in the chloroform layer. Finally, this layer was taken through filtration in a pre-weighed round bottom flask. Chloroform was evaporated under vacuum. Final weight of lipid was determined by the difference of final and initial weights of the flask. Thin layer chromatography (TLC) was done to separate polar lipids from nonpolar lipids and free fatty acids.
3.6. Separation of water-soluble lipids by TLC
TLC method was followed to separate the constituents of the extracted lipids from the aqueous part of the reaction broth. Glass plates (100 cm × 200 cm) coated with silica gel GF 254 (stationary phase with binder) were dried naturally under atmospheric condition. Subsequently they were activated at 100°C for 30 minutes and were cooled at room temperature (28°C). The extract was separated by using the polar mobile phase chloroform:methanol:aqueous acetic acid (65:15:2). The plates were visualized under UV lamp. Three golden spots appeared. The Rf (relative fronts of the solute and the solvent) values of the separated spots were calculated by the following equation:
3.7. Characterisation of biosurfactants
3.7.1. Measurement of surface tension and interfacial tension
The changes in surface tension of culture medium (aqueous layer), spent oil and the interfacial tension were evaluated by using a Leconde Du Nouy Tensiometer (Ring method).
3.7.2. Measurement of emulsification index (E24)
Emulsification index, E24 of culture samples was determined by adding 2 ml of hydrocarbon to the same volume of culture broth and by subsequently mixing in a vortex for 2 minutes and leaving to stand for 24 hours. The E24 index was calculated as percentage of height of emulsified layer (mm) on the basis of total height of the liquid column (mm).
4. Experimental
4.1 Optimization of oil to water phase ratio
Batch type experiments were conducted in 50-ml Erlenmeyer flasks by varying aqueous to spent oil ratio from 10:90 to 90:10. The total volume of oil–medium mixture was always kept at 20 ml. This medium was inoculated with 10% V/V stock culture. Batch type experiments were also conducted in 50-ml Erlenmeyer flasks with 20-ml aqueous culture (blank) and 2-ml stock culture to compare the growth in blank and spent oil medium. Each experiment was carried out under shaking condition at pH 8.0 and 160 rpm at 28°C. The values of initial specific growth rates were determined at all oil to water phase ratios. Maximum growth rate was obtained at oil to aqueous phase ratio of 70:30.
4.2 Determination of kinetic parameters
For the determination of kinetic parameters of Monod type growth model, experiments were conducted in 50-ml Erlenmeyer flasks by varying the initial concentrations of sulfur in spent engine oil in the range of 0.16–1.05% (w/v) by diluting with hexadecane. The ratio of oil to aqueous phase ratio was maintained at 70:30. Inoculation was done using the stock culture already adapted to spent engine oil. For each initial concentration of sulfur, one Erlenmeyer flask was withdrawn at an interval of 2 hours up to 48 hours. Concentration of biomass was determined using dry weight method described in Section 3.1. The oil and aqueous parts of the supernatants were analyzed for sulfur and biosurfactants respectively. The kinetic parameters, namely, µmax, Ks, Yx/s and have been determined using these data through regression analysis.
4.3. Operation in a bioreactor
Experiments were carried out in batch mode in a 5 L bioreactor with working volume of 2 L. Spent oil was used as the sulfur source. The sterile sulfur-free aqueous medium was used as nutrient broth of microorganisms. Based on the performance of the microorganisms in the small bioreactors (E. flasks), the oil to aqueous phase ratio was maintained at 70:30. The bioreactor was then inoculated by adding 10% v/v preadapted stock culture. Air was supplied through a compressor at a rate of 25 lph. Samples of reaction broth was collected at 2-hour interval up to 48 hours. The biomass concentrations of all samples and the sulfur concentration of oil phase of the samples were determined. The values of surface tension and the emulsification index of both the oil and the aqueous phases of the samples were also determined.
5. Theoretical analysis
Assuming balanced uninhibited growth, Monod type model has been attempted. The correlation between the specific growth rate, µ, and the concentration of sulfur in the spent oil phase may be written by the following equation:
6. Results and discussion
6.1. Zone of growth of microorganisms
From the visual observation of both small and large bioreactors, it was clear that the microorganisms grew only at the interface between the oil and the aqueous phase. This was also verified by FESEM analysis. Emulsion was also formed at the interface. and shows field emission scanning electron micrographs of the interfaces. It is apparent that in both the cases the microorganisms particularly reside at the interface to get nutrients from both the water and oil phases. Microorganisms, growing in the spent engine oil are smaller in size than those grown in diesel phase. This may be due to the presence of metal contaminants present in the spent engine oil. The biosurfactants like glycolipids, phospholipids, and rhamnolipids, secreted by the microorganisms, are preferentially transferred to the water phase to lower the surface tension. This facilitates the assimilation of nutrients by the microorganisms from the aqueous phase. Due to existence of the microorganisms only at the interface the treated spent oil can easily be separated from water phase and the interface containing microorganisms.
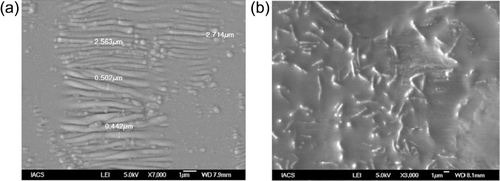
shows the initial value of the specific growth rate, µ as a function of the ratio of spent oil to aqueous phase in the small bioreactors. The sulfur content of the spent engine oil was maintained as the original value, i.e., 1.05%. From the figure, it is evident that maximum value of specific growth rate has been obtained at the oil to water ratio of 70:30. From the figure, it is also clear that as the fraction of oil is increased, the value of µ increases up to 70:30 and beyond this value a decreasing trend of µ is noticed. The increasing trend of specific growth rate with the increase of oil to aqueous phase ratio may be due to increase of availability of hydrocarbon and sulfur sources. Beyond 70:30, the availability of oxygen in the interfacial zone may decrease sharply due to the development of a thick layer of viscous spent engine oil. This ratio of 70:30 has therefore been maintained constant during the further experiments in small and large reactors.
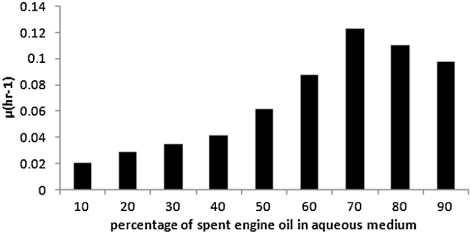
In , the double reciprocal plot has been made using the inverse of initial specific growth rates against the inverse of corresponding initial sulfur concentration in spent oil. The linearity of the plot validates the Monod type growth model indicating uninhibited growth of Rhopdococcus sp on sulfur on spent oil. Through regression analysis of the plotted data the values of µmax and Ks have been determined. The values of µmax, Ks, and Yx/s were determined as 0.12 h−1 and 73.5 mg/L and 0.32, respectively. The kinetic parameters are compared with those obtained for the growth of Rhopdococcus sp on sulfur of diesel oil (Citation24). The comparison unwinds the fact that the maximum specific growth rate, µmax and saturation constant, Ks are higher and lower respectively for growth on diesel, indicating higher growth rate on the latter, compared to that on spent oil. This may be due to the same reason as that for the shrinkage of cells, revealed by the FESEM photographs, shown in and .
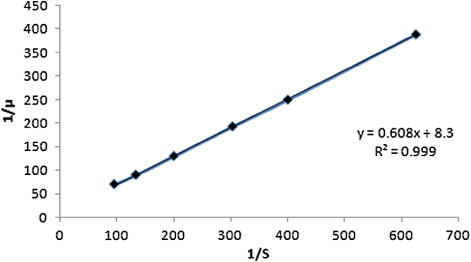
In and , the experimental values of concentrations of biomass in the reaction broth and sulfur in the oil phase of the large bioreactor respectively have been plotted against the batch time. It is observed from that the biomass concentration increases with growth time and reaches a saturation level at 30 hours. On the other hand, shows that the sulfur level of spent oil decreases as time progresses. Maximum sulfur conversion of 80% has been achieved. The results obtained from the large-scale reactor are in agreement with those (not shown) of small scale reactors. Sulfur present in the organo-sulfur compounds of spent oil was converted to corresponding sulfates which may migrate toward the aqueous phase. This was confirmed by the precipitation of BaSO4 when BaCl2 was added to the aqueous part of the growth medium.
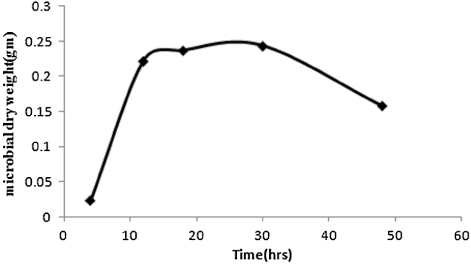
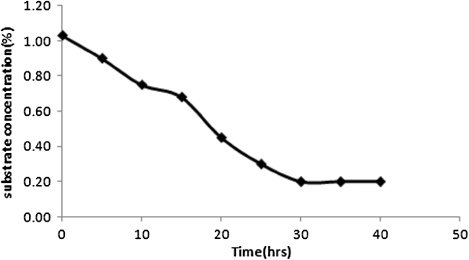
In and , the experimental values of surface tension and emulsification index of aqueous and oil phases of bioreactor samples have been plotted as a function of batch time. It has been observed that the surface tension decreases and the value of E24 increase with the increase in reaction time. The surface tensions of aqueous nutrient phase and spent oil have been observed to decrease from 69 dynes/cm to 24 dynes/cm and from 18 dynes/cm to 10 dynes/cm, respectively. Values of emulsification index (E24) of the spent oil layer of the culture broth have varied from 24 to 66 over the growth period of 32 hours in the bioreactor. Values of emulsification index (E24) of the aqueous layer of the culture broth have increased from 14 to 50 over the same growth period. This is an indication of the production of surface active reagents in the oil phase and other extracellular lipids like phospholipids, glycolipids, rhamnolipids, etc. in the aqueous phase with the propagation of reaction. It also establishes the effectiveness of the metabolic products, produced through biodesulfurization of spent oil, as surface active agents, and indicates that their generation is growth associated. Presence of phospholipids, glycolipids, rhamnolipids in the aqueous phase was confirmed by TLC. The Rf values were obtained as 0.92, 0.5, 0.81 for phospholipids, glycolipids, rhamnolipids, respectively which showed keen similarity with the corresponding values of literature. Glycolipid was confirmed by the formation of dark blue colorization as followed Morris test.
6.2. Gas chromatography
The GC–MS spectra of both treated and untreated spent engine oil were expressed as two wide overlaid bell-shaped curves from 10 minutes to 25 minutes (). The noise observed in these portions of both the chromatograms hindered identification of some abundant peaks. GC–MS spectrum of untreated spent oil showed the presence of more intense peaks compared to those of treated spent oil spectrum. The comparison between two spectra revealed that polyaromatic sulfur compounds like 1,1-dimethyltetradecyl hydrosulfide, 2-nonadecanone-(2,4-dinitrophenyl-hydrazone), S-[2-((3-[2,4-Dihydroxy-3,3-dimethylbutanoyl)amino] propanyl)amino)ethyl] 2-tetradecynethioate, etc., predominantly present in untreated oil, are absent in treated spent oil spectra. Mono- and di-aromatic compounds, long straight and branched chain hydrocarbons (carbon no. >15) are most abundant in both the cases. Treated spent oil spectra revealed the presence of some ethers, hydroxyls, aldehydes, ketones, and acids. Some examples are: 2-Octadecoxyethanol,2-methyl–hexadecan-1-ol,hexa-hydrofarsenol,valtrate,3-octyl-cis-oxyraneoctanoic acid,2E-5,7a isopropenyl-4,5-dimethyl-octahydro,1H-inden-4-yl)-3-methyl-2-pentenal. These types of compounds may contribute to the surface active properties (Citation28, Citation29) of microbially treated spent engine oil.
7. Conclusion
Rhodococcus sp exhibited good growth characteristics and high biodesulfurization activities on spent engine oil with simultaneous production of biosurfactants. Monod type model is valid for the growth of the bacterial strain on the sulfur compounds of spent oil. Same trends of conversion of sulfur and the growth of biomass have been observed in the small and the large bioreactors. Optimum value of oil to aqueous phase ratio with respect to the growth of biomass has been observed to be 70:30. Maximum sulfur conversion of 80% has been achieved in both small and large bioreactors. It may be concluded that surfactants, namely, hydroxyl compounds, carboxylic acids were in the oil phase and phospholipids, rhamnolipids, glycolipids, etc.(which were secreted from microorganisms) were in the aqueous phase. Both were effective surfactants and emulsifiers which were proved by the reduction of surface tension and enhancement of emulsification activities. GC–MS was done to characterize both untreated and treated spent engine oil. Thus, the biodesulfurization of spent engine oil may be recommended to generate ultra low sulfur diesel through pyrolysis and to produce useful biosurfactants.
Acknowledgment
We gratefully acknowledge Council of Scientific & Industrial Research (CSIR), India for their financial support.
References
- Kim, S.S.; Kim, S.H. Pyrolysis Kinetics of Waste Automobile Lubricating Oil. Fuel. 2000, 79 (15), 1943–1949. 10.1016/S0016-2361(00)00028-4
- Szewczyk, R.; D1ugonski, J. Pentachlorophenol and Spent Engine Oil Degradation by Mucor ramosissimus. Int. Biodeter. Biodegr. 2009, 63, 123–129. 10.1016/j.ibiod.2008.08.001
- Queiroga, C.L.; Nascimento, L.R.; Serra, G.E. Evaluation of Paraffins Biodegradation and Biosurfactant Production by Bacillus subtilis in the Presence of Crude Oil. Braz. J. Microbiol. 2003, 34 (4), 321–324. 10.1590/S1517-83822003000400006
- Achuba1, F.I.; Peretiemo-Clarke, B.O. Effect of Spent Engine Oil on Soil Catalase and Dehydrogenase Activities. Int. Agrophys. 2008, 22, 1–4.
- Achuba, F.I. Spent engine oil mediated oxidative stress in cowpea (Vigna unguiculata) seedlings. EJEAF Che. 2010, 9 (5), 910–917.
- Olugboji, O.A.; Ogunwole, O.A. Use of Spent Engine Oil. AUJT. 2008, 12 (1), 67–71.
- Ogbeide, S. O. An Investigation to the Recycling of Spent Engine Oil. J. Eng. Sci. Technol. Rev. 2010, 3 (1), 32–35.
- Montagnolli, R.N.; Lopes, P.R.M.; Bidoia, E.D. Applied Models to Biodegradation Kinetics of Lubricant and Vegetable Oils in Wastewater. Int. Biodeter. Biodegr. 2009, 63, 297–305. 10.1016/j.ibiod.2008.10.005
- Jhanani, S.; Kurian, J. Used Oil Generation and Management in the Automotive Industries. Int. J. Environ. Stud. 2011, 2 (2), 638–648.
- Mukhopadhyay, M.; Chowdhury, R.; Bhattacharya, P. Tricle Bed Biodesulfurizer of Diesel with Backwash and Recycle. AICHE. 2007, 53 (8), 2188–2197. 10.1002/aic.11240
- Mukhopadhyay, M.; Chowdhury, R.; Bhattacharya, P. Mathematical Simulation of Transient Behaviour of a Biotrickling Reactor for the Desulphurization of Diesel. Int. J. Chem. Sci. 2007, 5 (4), 1785–1796.
- Mukhopadhyay, M.; Chowdhury, R.; Bhattacharya, P. Biodesulfurization of Hydrodesulfurized Diesel in a Tricle Bed Reactor – Experiments and Modeling. J. Sci. Ind. Res. 2006, 65, 432–436.
- Guchait, S.; Chowdhury, R.; Biswas, D.; Bhattacharya, P. Biodesulfurization of Model Organo-sulfur Compounds and Hydrotreated Diesel – Experiments and Modeling. Chem. Eng. J. 2005, 112, 145–151. 10.1016/j.cej.2005.05.006
- Chowdhury, R.; DuttaGupta, A. Mathematical Modeling of the Transient Behavior of a Chemostat Undergoing Biodesulphfurization of Model Organo Sulphur Compounds and Diesel Using Pure and Isolated Bacterial Strains. IJCRE. 2005, 3, A60.
- Bandyopadhyay, S.; Chowdhury, R.; Bhattacharjee, C. Steady State Performance of a Bioreactor for Production of Near Zero Sulfur Diesel (NZSD) and Bio-surfactant. JOCET. 2013, 1 (3), 189–193. 10.7763/JOCET.2013.V1.43
- Bandyopadhyay, S.; Chowdhury, R.; Bhattacharjee, C. Mathematical Modeling of Production of Biosurfactant through Biodesulfurization of Hydrotreated Diesel in a Fermenter. International Conference on Modeling, Optimization, and Computing (ICMOS 2010), AIP Conference Proceedings. 2010, 1298, 232–237.
- Labana, S.; Pandey, G.; Jain, R.K. Desulfurization of Dibenzothiophene and Diesel Oils by Bacteria. Lett. Appl. Microbial. 2005, 40 (3), 159–163. 10.1111/j.1472-765X.2004.01648.x
- Yang, J.; Marison, I.W. Two Stage Process Design for the Biosulfurization of a Model of Diesel by a New Isolated Rhodococcus globerulus DAQ3. Biochem. Eng. J. 2005, 27, 77–82. 10.1016/j.bej.2005.08.012
- Gupta, N.; Roychowdhury, P.K.; Deb, J.K. Biotechnology of Desulfurization of Diesel: Prospects and Challenges. Appl. Microbial. Biotechnol. 2005, 66, 356–366. 10.1007/s00253-004-1755-7
- Raheb, J.; Hajipour, M.J.; Memari, B. Increasing of Biodesulfurization Activity of Newly Recombinant Pseudomonas aeruginosa ATCC 9027 by Cloning the Flavin Reductase Gene. IJBB. 2010, 6 (2), 219–229.
- Mohabali, G.; Bali, A.; Kayatash, A. Stabilization of Water/gas Oil Emulsions by Desulfurizing Cells of Gordonia alkanivorans RIPI90A. Microbiology. 2007, 153, 1573–1581. 10.1099/mic.0.2006/002543-0
- Yang, J.; Hu, Y.; Zhao, D.; Wang, S.; Lau, P.C.K.; Marison, I.W. Two-layer Continuous-process Design for the Biodesulfurization of Diesel Oils under Bacterial Growth Conditions. Biochem. Eng. J. 2007, 37, 212–218. 10.1016/j.bej.2007.04.012
- Ma, T.; Li, G.; Li, J.; Liang, F.; Liu, R. Desulfurisation of Dibenzothiothene by Bacillus subtilis recombinants carrying dszABC and dszD genes. Biotechnol. Lett. 2006, 28, 1095–1100. 10.1007/s10529-006-9056-0
- Bandyopadhyay, S.; Chowdhury, R.; Bhattacharya C.; Pan, S. Simultaneous Production of Biosurfactant and ULSD (Ultra Low Sulfur Diesel) using Rhodococcus sp. in a Chemostat. Fuel. 2013, 113, 107–112. 10.1016/j.fuel.2013.05.036
- Banat, I.M.; Makkar, R.S.; Cameotra, S.S. Potential Commercial Applications of Microbial Surfactants. Appl. Microbiol. Biotechnol. 2000, 53 (5), 495–508. 10.1007/s002530051648
- Bandyopadhyay, S.; Chowdhury, R.; Bhattacharjee, C. Kinetic Study of Production of Biosurfactant and Biodesulfurisation of Spent Engine Oil using Rhodococcus sp. 2012. CHEMCON-2012, NIT Jalandhar, Jalandhar, India, Dec 26–30, 2012.
- Bligh E.G.; Dyer, W.J. A Rapid Method for Total Lipid Extraction and Purification. Can. J. Biochem. Physiol. 1959, 37, 911–917. 10.1139/o59-099
- Muthusamy, K.; Gopalakrishnan, S.; Ravi, T.; Sivachidambaram, P. Biosurfactants: Properties, Commercial Production and Application. Curr. Sci. 2008, 94 (6), 736–747.
- Luna, J.M.; Rufino, R.D.; Sarubbo, L.A.; Campos-Takaki, G.M. Characterisation, Surface Properties and Biological Activity of a Biosurfactant Produced from Industrial Waste by Candida sphaerica UCP0995 for Application in the Petroleum Industry. Colloids and Surf. B. 2013, 102, 202–209. 10.1016/j.colsurfb.2012.08.008