ABSTRACT
Regioselective ring opening of aliphatic and aromatic epoxides with nitrogen heterocycles such as indoles and imidazoles was accelerated using an ultrasonic technique as a green approach. An optimized procedure with the catalyst of choice, MCM-41, represents a real alternative to the conventional reaction protocols owing to the catalyst recyclability, simplicity, green conditions and time-saving aspects.
GRAPHICAL ABSTRACT
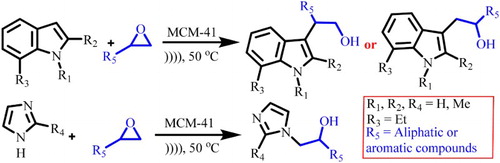
Introduction
In recent years, ultrasound irradiation has been demonstrated as an alternative energy source for organic and inorganic reactions ordinarily accomplished by heating (Citation1). Particularly in organic chemistry, there is a growing demand for designing efficient and eco-safe synthetic under ultrasound irradiation (Citation2–4). Compared with traditional methods, the notable features of the ultrasound approach are rapidity and formation of pure products in high reaction yields, easier manipulation, energy conservation and waste minimization (Citation5, Citation6).
MCM-41, one member of the M41S family, possesses a regular hexagonal array of uniform pore openings with a broad spectrum of pore diameters between 15 and 100 Å (Citation7). The mesoporous structure can be controlled by a sophisticated choice of templates such as surfactants and changing reaction parameters such as temperature and compositions. This heterogeneous solid acid catalyst has shown versatile usage and widely applied in the synthesis of various chemicals due to their well-defined micro-structure which forms the basis of uniform active sites and well-controlled steric effects, high acid strength, hydrophilic and hydrophobic properties and reusability that make them promising candidates for catalytic applications (Citation8, Citation9). Therefore, several derivatives of MCM-41 including Mn(bpdo)2Cl2/MCM-41 (Citation10), Mn-MCM-41 (Citation11, Citation12), Cr-MCM-41 (Citation13) and Al-MCM-41 (Citation14) have been prepared and utilized to catalyze various reactions.
Indoles and imidazoles alkylated especially 3-alkylindoles are a key structural motif in many biologically active compounds (Citation15, Citation16) as well as in many natural products (Citation17). For example, soraphinol A (Citation18) and actinopolymorphol B (Citation19) are known as protein farnesyl transferase inhibitors that have anticancer properties ().
Due to the nucleophilic nature of indoles and imidazoles and versatile usage of these compounds in medicine and biochemistry, various methods have been developed for alkylation of these compounds (Citation20, Citation21). On the other hand, epoxides are one of the most practical compounds in alkylation of nitrogen heterocycles. Some methodologies for opening of epoxides with indoles have been reported by using acid catalysts such as RuCl3·nH2O (Citation22), Bi(OTf)3 (Citation23), nano TiO2 (Citation24), SbCl3/K-10 (Citation25), S-ZrO2 (sulfated zirconia) (Citation26), HBF4-SiO2 (Citation27), nano MgO (Citation28), magnetic nano Fe3O4 and CuFe2O4 (Citation29), activated silica (Citation30) and high-pressure conditions (Citation31). While such methods have expedited alkylation of nitrogen heterocycles, they showed varying grades of success as well as limitations such as prolonged reaction times, low yields, use of toxic solvents and expensive catalysts. Consequently, there is scope for further work toward the development of an inexpensive, cleaner, eco-friendly procedure that takes a short reaction time.
As a consequence of our interest in the synthesis of organic compounds accelerated by ultrasound (Citation4, Citation32) and to develop an environmentally benign synthesis in chemical research (Citation9, Citation33–35), we investigated an efficient and green method in alkylation of nitrogen heterocycles under ultrasound irradiation. The objective of this article is to report the details of alkylation of indoles and imidazoles with epoxides to prepare alcohols using catalytic amount of mesoporous MCM-41 ().
Result and discussion
At the onset of our work, different mesoporous materials including Mn(bpdo)2Cl2/MCM-41, Mn-MCM-41, Cr-MCM-41, Al-MCM-41 and MCM-41 were screened for their ability to catalyze the ring opening of epoxides with nitrogen heterocycles under ultrasound irradiation in solvent-free conditions. Treatment of indole 1a with styrene oxide 1b was selected as a model reaction. The presence of complex of Mn(bpdo)2Cl2 in the channels of MCM-41 led to a decrease in the silanol groups of MCM-41 and active sites in the surface of catalyst and decreased the yield of the reaction (, entry 7). On the other hand, introducing chromium and manganese ions into the MCM-41 surface led to reduce surface area of catalysts and as shown in , these catalysts afforded the desired products only in moderate yields (, entries 5 and 6). The higher catalytic activity of Al-MCM-41 is attributed to the presence of acidic sites arising from the AlO4 tetrahedral units in the framework (Citation14) and the desired product 3a could be obtained in isolated yields of 64% (, entry 4). However, MCM-41 could efficiently catalyze the ring opening reaction of epoxides to afford the desired products in higher yields and relatively shorter reaction time (, entry 3). Using different dosage of MCM-41 indicated that the highest yield (80%) could be obtained with 0.05 g. Therefore, the use of just 0.05 g of MCM-41 was chosen as a quantity of the catalyst to push the reaction forward with a maximum yield of the product.
Table 1. Screening of different catalysts for synthesis of 3a.
In this study, a comparison between conventional and ultrasound conditions for the ring opening of styrene oxide 1b with indole 1a in the presence of MCM-41 was investigated. As shown in , the synthesis of 3a under ultrasound irradiation was more efficient in terms of yield and reaction time.
Table 2. Compare of yields of conventional procedures with ultrasonic energy for synthesis of 3a in presence of MCM-41.
In another experiment, the reaction of 1a and 1b was used as the model system to evaluate the suitability of solvent-free conditions for this reaction. The reaction was also performed in various solvents including ethanol, chloroform, acetonitrile, toluene, water and solvent-free conditions for comparison purposes. The results are summarized in . It is noteworthy mentioning that in comparison with organic solvents, solvent-free conditions under ultrasonic irradiation was found to give the best results (, entry 3). These conditions can have substantial effects in realizing the goals of green chemistry.
Table 3. Optimization of the reaction conditions under ultrasound irradiation.
To evaluate the scope of catalyst's application, various structurally different epoxides were tested with substituted indoles under the optimized conditions and the results are presented in . The reactions proceeded smoothly to afford the corresponding products in good yields with high regioselectivity. Aryl epoxides (, entries 3a, 3d, 3e and 3f) underwent cleavage by indoles with favored attack at the benzylic position of the aromatic oxiranes, resulting in the formation of primary alcohols. For example, 1H NMR chemical shift and splitting pattern of compound 3e show these characteristic peaks: 4.18–4.09 (m, 2H, CH2), 4.41 (t, 1H, J = 6.7 Hz, CH) that is in agreement with the proposed structure. Hence, it was proposed that the electronic effect predominated over steric effect in aryl epoxides. On the other hand, in aliphatic oxiranes (, entries 3b, 3c, 3g and 3h), the reaction likely occurred through an attack by indole on the less-substituted carbon atom. 1H NMR data for compound 3c, 3.12–3.21 (2H, m, 2CH), 4.18–4.25 (2H, m, 2CH), 4.41–4.43 (1H, m, CH), clearly confirm this reaction pattern. It seems that the steric effect predominated over electronic effect in aliphatic oxiranes. In general, aliphatic epoxides were not suitable substrates for our reaction conditions, giving indolyl alcohols in low isolated yields and with small regiocontrol. Indoles bearing electron-donating groups provided higher reaction rates, afforded the alcohols in moderate to good yields. On the other hand, the presence of electron-withdrawing groups on the indole significantly decreased the rate of the reaction and a low yield of product was obtained even after prolonged reaction time.
Table 4. Ring opening of aliphatic and aromatic epoxides with nitrogen heterocycles catalyzed by MCM-41.
In addition, we also investigated the possibility of applying this method to the synthesis of alkylated imidazoles. Thus, the reaction of imidazoles with aromatic and aliphatic epoxides afforded the corresponding imidazoles derivatives in good yields (, entries 3i, 3j and 3k). Imidazole, with a pKa of 7.1, is a very much stronger base and nucleophile than indole, consequently imidazoles can be alkylated on nitrogen easily. Ring opening occurred exclusively at the less hindered carbon of the epoxide that carry out with SN2-type attack by imidazole nitrogen.
Conclusion
A green and expeditious ultrasound irradiation-assisted method for the regioselective ring opening of epoxides using MCM-41-based catalyst has been developed. Enhanced yield and rate of the reaction was achieved by using MCM-41 catalyst with ultrasound and the method was applied to the differently substituted nitrogen heterocycles with epoxides. Such novel developed method offers high yield, simple and waste-free chemical process for the ring opening of epoxides.
Experimental
All the chemicals were purchased from Merck Company. All products are known compounds and were characterized by mp, IR, 1H NMR, 13C NMR and CHN. Melting points are measured using Barnstead Electro thermal. 1H NMR and 13C NMR spectra were recorded on a Bruker AQS-AVANCE spectrometer at 500 MHz, using tetramethylsilane as an internal standard. CHN performed by a Perkin Elmer 2004 (II) CHN analyzer. The reactions were carried out in an ultrasonic cleaning bath (Branson 5200 E4).
Typical procedure for alkylation of nitrogen heterocycles with epoxides
Typical stirring method
A mixture of indole (1 mmol) and styrene oxide (3 mmol) and MCM-41 (0.05 g) were stirred at room temperature (method A) and 50°C (method B) for the appropriate period of time. The progress of the reaction was monitored by TLC. After completion of the reaction, the crude was diluted with ethanol (10 mL), the catalyst was removed by filtration and the filtrate dried with MgSO4, and evaporated to give the product. Further purification was achieved by silica gel chromatography using ethyl acetate/petroleum ether as eluent to afford pure products.
Ultrasound irradiation
To a mixture of indole or imidazole (1 mmol) and epoxide (3 mmol), MCM-41 (0.05 g) was added, and the mixture was sonicated in a sonic bath under ultrasound irradiation maintained at 50°C for the appropriate period of time. The progress of the reaction was monitored by TLC. After completion of the reaction, the crude was diluted with ethanol (10 mL), the catalyst was removed by filtration and the filtrate dried with MgSO4, and evaporated to give the product. Further purification was achieved by silica-gel chromatography using ethyl acetate/petroleum ether as eluent to afford pure products.
Compound 3c: 1-(1H-indol-3-yl)-3-phenoxypropan-2-ol
IR (KBr): 3435, 3306, 3034, 2945, 1592, 1459, 1328, 1256 cm–1; 1H NMR (500 MHz, CDCl3): δ 1.63 (s, 1H, OH), 3.12–3.21 (m, 2H, 2CH), 4.18–4.25 (m, 2H, 2CH), 4.41–4.43 (m, 1H, CH), 6.94 (d, 1H, J = 8.0 Hz, ArH), 7.06 (t, 1H, J = 7.3 Hz, ArH), 7.18–7.27 (m, 4H, ArH), 7.38–7.43 (m, 2H, ArH), 7.47 (d, 1H, J = 8.0 Hz, ArH), 7.54 (d, 1H, J = 7.3 Hz, ArH), 8.11 (s, 1H, NH) ppm; 13C NMR (125 MHz, CDCl3): δ 29.5, 70.7, 71.1, 111.6, 111.8, 116.0, 119.3, 121.5, 121.9, 123.0, 123.3, 129.6, 129.7, 135.7, 159.9 ppm; Anal. Calcd for C17H17NO2: C, 76.38; H, 6.41; N, 5.24. Found: C, 76.36; H, 6.48; N, 5.29.
Compound 3e: 2-(4-chlorophenyl)-2-(1-methyl-1H-indol-3-yl) ethanol
IR (KBr): 3369, 2933, 1485, 1054, 813 cm−1; 1H NMR (500 MHz, CDCl3): δ 1.62 (s, 1H, OH), 3.74 (s, 3H, CH3), 4.18–4.09 (m, 2H, CH2), 4.41 (t, 1H, J = 6.9 Hz, CH), 7.01 (d, 1H, J = 8.0 Hz, Ar), 7.12–7.17 (m, 2H, Ar), 7.20 (d, 1H, J = 8.0 Hz, Ar), 7.25–7.31 (m, 4H, Ar), 7.39 (d, 1H, J = 7.2 Hz, Ar) ppm; 13C NMR (125 MHz, CDCl3): δ 32.8, 45.1, 66.3, 109.4, 114.0, 119.1, 119.3, 122.0, 126.8, 127.3, 128.2, 129.7, 132.4, 137.2, 140.5 ppm; Anal. Calcd for C17H16ClNO: C, 71.45; H, 5.64; N, 4.90. Found: C, 71.50; H, 5.68; N, 4.86.
Compound 3f: 2-(7-ethyl-1H-indol-3-yl)-2-phenylethanol
IR (KBr): 3421, 3389, 3058, 2955, 1432, 1129 cm−1; 1H NMR (500 MHz, CDCl3): δ 1.33 (t, 3H, J = 7.7 Hz, CH3), 1.83 (s, 1H, OH), 2.90 (q, 2H, J = 7.7 Hz, CH2), 4.16–4.03 (m, 2H, CH2), 4.38 (t, 1H, J = 6.9 Hz, CH), 6.88 (d, 1H, J = 7.9 Hz, Ar), 6.98–7.04 (m, 2H, Ar), 7.12 (d, 1H, J = 7.9 Hz, Ar), 7.20–7.26 (m, 3H, Ar), 7.31–7.35 (m, 2H, Ar), 8.12 (s, 1H, NH) ppm; 13C NMR (125 MHz, CDCl3): δ 13.2, 23.8, 46.8, 66.3, 115.3, 116.9, 119.2, 121.2, 121.8, 125.9, 126.4, 129.5, 129.9, 134.5, 141.6 ppm; Anal. Calcd for C18H19NO: C, 81.47; H, 7.22; N, 5.28. Found: C, 81.45; H, 7.27; N, 5.33.
Compound 3g: 1-chloro-3-(1H-indol-3-yl)-2-propanol
IR (KBr): 3425, 3370, 2993, 1423, 1132, 825 cm−1; 1H NMR (500 MHz, CDCl3): δ 1.87 (s, 1H, OH), 3.10–3.15 (m, 2H, CH2), 3.54–3.57 (m, 2H, CH2), 4.12–4.16 (m, 1H, CH), 6.98 (d, 1H, J = 7.9 Hz, Ar), 7.18–7.22 (m, 3H, ArH), 7.59 (s, 1H, ArH), 8.10 (s, 1H, NH) ppm; 13C NMR (125 MHz, CDCl3): δ 30.3, 46.4, 71.3, 115.2, 111.4, 118.6, 119.0, 121.4, 122.8, 129.3, 134.7 ppm; Anal. Calcd for C11H12ClNO: C, 63.01; H, 5.77; N, 6.68. Found: C, 63.05; H, 5.80; N, 6.65.
Compound 3k: 2-(2-methyl-1H-imidazol-1-yl)-1-phenylethanol
IR (KBr): 3223, 2988, 1500, 1435, 1118 cm−1; 1HNMR (500 MHz, CDCl3): δ 2.15 (s, 3H, CH3), 4.05–4.09 (m, 2H, 2CH), 4.37 (s, 1H, OH), 4.89–4.93 (m, 1H, CH), 6.73 (d, 1H, J = 1.5 Hz, CH), 6.84 (d, 1H, J = 1.5 Hz, CH), 7.27–7.32 (m, 3H, ArH), 7.42–7.45 (m, 2H, ArH) ppm; 13C NMR (125 MHz, CDCl3): δ 12.6, 53.2, 72.9, 121.4, 1254.9, 126.7, 129.0, 129.9, 143.3, 145.9 ppm; Anal. Calcd for C12H14 N2O: C, 71.26; H, 6.98; N, 13.85. Found: C, 71.23; H, 7.02; N, 13.89.
Catalyst preparation
MCM-41 was prepared according to the procedure described previously (Citation36). In all, 1.8 g of SiO2 was added to a solution prepared by dissolving 0.6 g of NaOH in 25 mL of water. The resultant mixture was stirred for 3 h, and then 1.9 g of cetyltrimethyl ammonium bromide (CTABr) in 20 mL of water was added to this solution and stirred for one more hour. The molar composition of the initial gel mixture was 1.0:7.5:5.2:2500 SiO2/Na2O/CTABr/H2O. The result reaction was kept overnight and poured into a Teflon lined stainless steel autoclave. Crystallization was performed at 100°C for 2 h under autogenous pressure. The solid product was obtained by filtration, washed with deionized water, dried in air at 353 K and calcined in air at 813 K for 24 h to remove the CTABr.
shows the general characteristic of catalyst including Brunauer–Emmett–Teller (BET) surface area, unit cell, d-spacing value, pore size and wall thickness. As shown in , the catalyst exhibits a typical behavior of a uniform mesoporous materials with three distinct stages in the isotherms. The initial increase in the nitrogen uptake at low relative pressure could be ascribed to monolayer absorption on the pore walls. However, a sharper step at P/P0∼0.35 indicates the capillary condensation in the mesopore and the increase at high relative pressure could be associated with multilayer adsorption on the external surface of the catalyst.
Table 5. Physicochemical characterization of the catalyst.
Reusability of MCM-41
The recovery and reusability of the catalyst, which are very important for industrial purposes and is highly recommended for green processes, were also explored in the model reaction under ultrasonic irradiation. At the end of the reaction, the catalyst could be recovered by a simple filtration, washed with methanol, dried in a vacuum at 70°C overnight and subjected to a second run of the reaction process. In , the comparison of efficiency of MCM-41 in synthesis of 3a after five times is reported. As shown, the first reaction using recovered MCM-41 afforded a similar yield to that obtained in the first run. In the second, third, fourth and fifth runs, the yields were gradually decreased.
Disclosure statement
No potential conflict of interest was reported by the authors.
References
- Mason, T.J. Ultrasound in Synthetic Organic Chemistry. Chem. Soc. Rev. 1997, 26 (6), 443–451. doi: 10.1039/cs9972600443
- Anastas, P.T.; Zimmerman, J.B. Peer Reviewed: Design Through the 12 Principles of Green Engineering. Environ Sci Technol. 2003, 37 (5), 94A–101A. doi: 10.1021/es032373g
- Cella, R.; Stefani, H.A. Ultrasonic Reactions. In Green Techniques for Organic Synthesis and Medicinal Chemistry: Zhang, W., Cue, B.W., Eds.; John Wiley & Sons, Ltd: New York, 2012; pp 343–361.
- Zakeri, M.; Nasef, M.M.; Abouzari-Lotf, E. Eco-safe and Expeditious Approaches for Synthesis of Quinazoline and Pyrimidine-2-amine Derivatives Using Ionic Liquids Aided with Ultrasound or Microwave Irradiation. J. Mol. Liq. 2014, 199, 267–274. doi: 10.1016/j.molliq.2014.09.018
- Almeida, Q.A.R.; Faria, R.B. Synthesis of Highly Substituted Pyrroles Using Ultrasound in Aqueous Media. Green Chem. Lett. Rev. 2012, 6 (2), 129–133. doi: 10.1080/17518253.2012.713999
- Pacheco, B.S.; Nunes, C.F.P.; Rockembach, C.T.; Bertelli, P.; Mesko, M.F.; Roesch-Ely, M.; Moura, S.; Pereira, C.M.P. Eco-friendly Synthesis of Esters Under Ultrasound with p-toluenesulfonic Acid as Catalyst. Green Chem. Lett. Rev. 2014, 7 (3), 265–270. doi: 10.1080/17518253.2014.941950
- Beck, J.S.; Vartuli, J.C.; Roth, W.J.; Leonowicz, M.E.; Kresge, C.T.; Schmitt, K.D.; Chu, C.T.W.; Olson, D.H.; Sheppard, E.W. A New Family of Mesoporous Molecular Sieves Prepared with Liquid Crystal Templates. J. Am. Chem. Soc. 1992, 114 (27), 10834–10843. doi: 10.1021/ja00053a020
- Maschmeyer, T.; Rey, F.; Sankar, G.; Thomas, J.M. Heterogeneous Catalysts Obtained by Grafting Metallocene Complexes onto Mesoporous Silica. Nature. 1995, 378 (6553), 159–162. doi: 10.1038/378159a0
- Heravi, M.M.; Zakeri, M.; Haghi, H. MCM-41 Mesoporous Silica: Efficient and Reusable Catalyst for the Synthesis of 2,4,5-Trisubstituted Imidazoles Under Solvent-Free Conditions. Synth. React. Inorg. Met.-Org. Nano-Met. Chem. 2011, 41 (10), 1310–1314. doi: 10.1080/15533174.2011.609211
- Malakooti, R.; Farzaneh, F.; Ghandi, M. Synthesis, Characterization and Studies on Catalytic Behavior of Mn(П) Complex with 2, 2′ Bipyridine, 1, 1′ Dioxide Ligand within Nanoreactors of MCM-41. J. Sci. Islamic Republic of Iran. 2006, 17 (1), 43.
- Zhao, D.; Goldfarb, D. Synthesis of Mesoporous Manganosilicates: Mn-MCM-41, Mn-MCM-48 and Mn-MCM-L. J. Chem. Soc. Chem. Commun. 1995 (8), 875–876. doi: 10.1039/c39950000875
- Selvaraj, M.; Sinha, P.K.; Lee, K.; Ahn, I.; Pandurangan, A.; Lee, T.G. Synthesis and Characterization of Mn–MCM-41and Zr–Mn-MCM-41. Microporous Mesoporous Mater. 2005, 78 (2–3), 139–149. doi: 10.1016/j.micromeso.2004.10.004
- Atchudan, R.; Pandurangan, A. The Use of Bimetallic MCM-41 Mesoporous Catalysts for the Synthesis of MWCNTs by Chemical Vapor Deposition. J. Mol. Catal. A: Chem. 2012, 355, 75–84. doi: 10.1016/j.molcata.2011.11.028
- Wang, J.A.; Chen, L.F.; Noreña, L.E.; Navarrete, J. Spectroscopic Study and Catalytic Evaluation of Mesostructured Al-MCM-41 and Pt/H3PW12O40/Al-MCM-41 Catalysts. Appl. Catal. A: Gen. 2009, 357 (2), 223–235. doi: 10.1016/j.apcata.2009.01.023
- Russell, M.G.N.; Baker, R.J.; Barden, L.; Beer, M.S.; Bristow, L.; Broughton, H.B.; Knowles, M.; McAllister, G.; Patel, S.; Castro, J.L. N-Arylsulfonylindole Derivatives as Serotonin 5-HT6 Receptor Ligands. J. Med. Chem. 2001, 44 (23), 3881–3895. doi: 10.1021/jm010943m
- Zhang, H.-C.; Ye, H.; Moretto, A.F.; Brumfield, K.K.; Maryanoff, B.E. Facile Solid-Phase Construction of Indole Derivatives Based on a Traceless, Activating Sulfonyl Linker. Org. Lett. 1999, 2 (1), 89–92. doi: 10.1021/ol991255o
- Saxton, J.E. Recent Progress in the Chemistry of Indole Alkaloids and Mould Metabolites. Nat. Prod. Rep. 1989, 6 (1), 1–54. doi: 10.1039/np9890600001
- Li, X.; Zee, O.P.; Shin, H.J.; Seo, Y.; Ahn, J.-W. Soraphinol A, a New Indole Alkaloid from Sorangium cellulosum. Bull. Korean Chem. Soc. 2007, 28 (5), 835. doi: 10.5012/bkcs.2007.28.5.835
- Ghosh, N.; Nayak, S.; Sahoo, A.K. Gold-Catalyzed Regioselective Hydration of Propargyl Acetates Assisted by a Neighboring Carbonyl Group: Access to α-Acyloxy Methyl Ketones and Synthesis of (±)-Actinopolymorphol B. J. Org. Chem. 2010, 76 (2), 500–511. doi: 10.1021/jo101995g
- Kang, Q.; Zhao, Z.-A.; You, S.-L. Highly Enantioselective Friedel−Crafts Reaction of Indoles with Imines by a Chiral Phosphoric Acid. J. Am. Chem. Soc. 2007, 129 (6), 1484–1485. doi: 10.1021/ja067417a
- Jorapur, Y.R.; Lee, C.-H.; Chi, D.Y. Mono- and Dialkylations of Pyrrole at C2 and C5 Positions by Nucleophilic Substitution Reaction in Ionic Liquid. Org. Lett. 2005, 7 (7), 1231–1234. doi: 10.1021/ol047446v
- Tabatabaeian, K.; Mamaghani, M.; Mahmoodi, N.O.; Khorshidi, A. Solvent-free, Ruthenium-catalyzed, Regioselective Ring-opening of Epoxides, an Efficient Route to Various 3-alkylated Indoles. Tetrahedron Lett. 2008, 49 (9), 1450–1454. doi: 10.1016/j.tetlet.2008.01.001
- Yadav, J.S.; Reddy, B.V.S.; Satheesh, G. Bi(OTf)3-catalyzed Allylation of Epoxides: A Facile Synthesis of Homoallylic Alcohols. Tetrahedron Lett. 2003, 44 (34), 6501–6504. doi: 10.1016/S0040-4039(03)01559-4
- Kantam, M.L.; Laha, S.; Yadav, J.; Srinivas, P. Synthesis of 2-Indolyl-1-nitroalkane Derivatives Using Nanocrystalline Titanium(IV) Oxide. Synth. Commun. 2009, 39 (22), 4100–4108. doi: 10.1080/00397910902883728
- Liu, Y.-H.; Liu, Q.-S.; Zhang, Z.-H. An Efficient Friedel–Crafts Alkylation of Nitrogen Heterocycles Catalyzed by Antimony trichloride/montmorillonite K-10. Tetrahedron Lett. 2009, 50 (8), 916–921. doi: 10.1016/j.tetlet.2008.12.022
- Abbaraju, R.R.; Dasgupta, N.; Virkar, A.V. Composite Nafion Membranes Containing Nanosize TiO2∕SnO2 for Proton Exchange Membrane Fuel Cells. J. Electrochem. Soc. 2008, 155 (12), B1307–B1313. doi: 10.1149/1.2994079
- Bandgar, B.P.; Patil, A.V. Fluoroboric Acid Adsorbed on Silica Gel Catalyzed Regioselective Ring Opening of Epoxides with Nitrogen Heterocycles. Tetrahedron Lett. 2007, 48 (1), 173–176. doi: 10.1016/j.tetlet.2006.10.118
- Hosseini-Sarvari, M.; Parhizgar, G. Regioselective Friedel-Crafts Alkylation of Indoles with Epoxides Using Nano MgO. Green Chem. Lett. Rev. 2012, 5 (3), 439–449. doi: 10.1080/17518253.2012.666273
- Parella, R.; Naveen; Babu, S.A. Magnetic Nano Fe3O4 and CuFe2O4 as Heterogeneous Catalysts: A Green Method for the Stereo- and Regioselective Reactions of Epoxides with Indoles/pyrroles. Catal. Commun. 2012, 29, 118–121. doi: 10.1016/j.catcom.2012.09.030
- Mangu, N.; Kaiser, H.M.; Kar, A.; Spannenberg, A.; Beller, M.; Tse, M.K. Synthesis of Novel Hymenialdisine Analogues Using Solvent-free and Silica Gel-promoted Ring Opening of Epoxides. Tetrahedron. 2008, 64 (30–31), 7171–7177. doi: 10.1016/j.tet.2008.05.092
- Kotsuki, H.; Nishiuchi, M.; Kobayashi, S.; Nishizawa, H. High-pressure Organic Chemistry. 10. Novel Neutral Alkylations of Indoles and Pyrroles with Vinyl Epoxides at High Pressure. J. Org. Chem. 1990, 55 (9), 2969–2972. doi: 10.1021/jo00296a079
- Zakeri, M.; Nasef, M.; Abouzari-Lotf, E.; Haghi, H. Ultrasound-assisted Regioselective Ring Opening of Epoxides with Nitrogen Heterocycles using Pyrrolidonium and Imidazolium-based Acidic Ionic Liquids. Res. Chem. Intermed. 2015, 41 (12), 10097–10108.
- Heravi, M.M.; Zakeri, M. Use of Sodium Molybdate Dihydrate as an Efficient Heterogeneous Catalyst for the Synthesis of Benzopyranopyrimidine Derivatives. Synth. React. Inorg. Met.-Org. Nano-Met. Chem. 2012, 43 (2), 211–216. doi: 10.1080/15533174.2012.740717
- Zakeri, M.; Nasef, M.M.; Abouzari-Lotf, E.; Moharami, A.; Heravi, M.M. Sustainable Alternative Protocols for the Multicomponent Synthesis of Spiro-4H-pyrans Catalyzed by 4-dimethylaminopyridine. J. Ind. Eng. Chem. 2015 , 29, 273–281.
- Zakeri, M.; Heravi, M.; Abouzari-Lotf, E. A New One-pot Synthesis of 1,2,4-oxadiazoles from Aryl Nitriles, Hydroxylamine and Crotonoyl Chloride. J. Chem. Sci. 2013, 125 (4), 731–735. doi: 10.1007/s12039-013-0426-6
- Zhao, X.S.; Lu, G.Q.; Hu, X. Characterization of the Structural and Surface Properties of Chemically Modified MCM-41 Material. Microporous Mesoporous Mater. 2000, 41 (1–3), 37–47. doi: 10.1016/S1387-1811(00)00262-6