ABSTRACT
A green method for the synthesis of dihydropyrano[4,3-b]pyranes via a three-component reaction catalyzed by lipase is reported firstly. Under the optimal conditions, high yields of dihydropyrano[4,3-b]pyranes (80.8–96.8%) could be obtained in this enzymatic reaction. This study not only expands the application of lipase in organic synthesis, but also provides an alternative efficient method for the synthesis of dihydropyrano[4,3-b]pyranes.
GRAPHICAL ABSTRACT
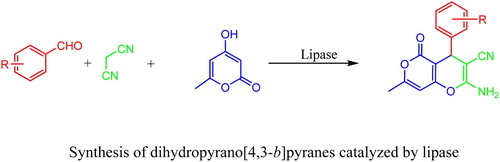
Introduction
Multi-component reactions (MCRs) can produce many complex molecules with interesting biological activities (Citation1–3) and have drawn much attention for their high atom and step economy (Citation4–7). In recent years, enzymes have been successfully introduced into MCRs and have become a research hotspot in organic chemistry. It is well known that enzymatic processes are environmentally friendly, cost-effective and sustainable. With the development of enzyme catalytic promiscuity, which refers to the ability of an enzyme to carry out different types of reactions relative to its main reaction, several elegant enzymatic MCRs have been designed to produce the structurally complex molecules, and these investigations have greatly widened the application of enzyme in MCRs (Citation8–15). For example, Kłossowski et al. presented an enzyme-catalyzed Ugi reaction (Citation16). They successfully combined the enzyme promiscuity and MCR advantages in the formation of dipeptide. A lipase-catalyzed three-component Hantzsch-type reaction has also been reported by Lin et al. (Citation17). A series of 1,4-dihydropyridines have been obtained in moderate to excellent yields through this green method. However, there still remains relatively little research so far on the application of enzyme in MCR.
It is well known that the derivatives of dihydropyrano[4,3-b]pyranes have a broad spectrum of biological and pharmaceutical activities (Citation18–20). Several synthesis methods have been developed with aromatic aldehyde, malononitrile and 4-hydroxy-2H-pyran-2-one analogs used as substrates. The condensation of these three components could be catalyzed by piperidine/triethylamine, ZrO2 nanoparticle, basic reagents (NaOH, K2CO3) or Lewis acids (TiCl4, InCl3) (Citation21–25). However, there is no literature reported about the synthesis of dihydropyrano[4,3-b]pyranes by a enzymatic method. In this study, we have designed a novel and green MCR for the synthesis of dihydropyrano[4,3-b]pyranes catalyzed by lipase (Scheme 1). Compared to the chemical processes, this method is mild, efficient and environment-friendly.
Results and discussion
Initially, we select benzaldehyde (1a), malononitrile (2) and 4-hydroxy-6-methyl-2H-pyran-2-one (3) as the substrates of the three-component reaction in this study. The catalytic performance of lipases from different sources for this reaction was primarily evaluated. As depicted in , no product could be detected in the control reactions (entry 6, 7), and the highest yield was achieved when PPL was used as the catalyst in this reaction. Thus, PPL was selected for further study. These results indicated that a special active conformation of enzyme played a crucial role in this MCR.
Table 1. Three-component reaction catalyzed by different lipasesa.
In some organic mediums, lipase can remain in active conformation and possess high catalytic ability (Citation26). In this study, various organic solvents with different log P-values were selected to investigate the effect of the reaction medium. As shown in , the catalytic activity of PPL was significantly influenced by the solvent and i-propanol afforded the highest yield. It seems that there is no evident correlation between the value of log P and catalytic efficiency of PPL. However, the solvent can actually lead to a conformational change of the enzyme and then affect the enzyme activity. Further investigation is being conducted and will be reported in the future.
Table 2. Effect of organic solvents on the three-component reactiona.
Temperature is another important factor in a lipase-catalyzed reaction. In the present study, the reaction temperature was changed from 20°C to 80°C to investigate its effect. The results in showed that the catalytic performance of PPL depended strongly on the reaction temperature. The yield increased as temperature was increased from 20°C to 60°C, followed by a decrease at higher temperature. According to Arrhenius theory, temperature can increase the internal energy of the substrate molecules to overcome the energy barrier and thus enhance the reaction rate. Besides, higher temperature will increase the collision chance between enzyme and substrate molecules, which might also help form the enzyme–substrate complexes and then improve the reaction rate. Further increasing of temperature would destruct the enzyme conformation and decrease the enzyme activity.
Figure 1. Effect of temperature on the three-component reaction.
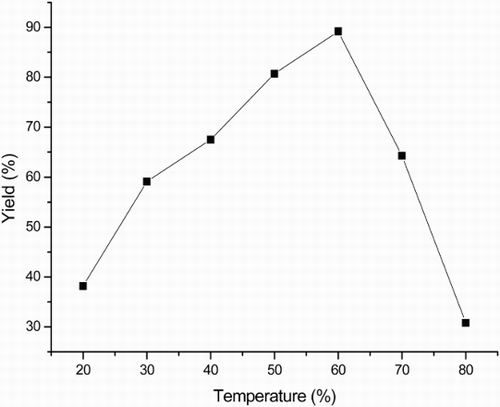
Generally, enzyme dosage can influence the reaction rate during the enzymatic reaction (Citation27). In this study, the effect of enzyme dosage has been investigated, and the results were shown in . It was found that the yield of dihydropyrano[4,3-b]pyranes (4) increased with increasing lipase dosage and changed slightly when the dosage was above 120 U. When the enzyme dosage was increased, more enzyme molecules would take part in the reaction and then increased the efficiency of the reaction. However, high enzyme dosage may cause the aggregation of enzyme, decrease the stirring effect or disrupt the water activity, which may have some negative effects on the reaction process. So, there was no obvious difference when higher enzyme dosage was used in this reaction system.
Figure 2. Effect of enzyme dosage on the three-component reaction.
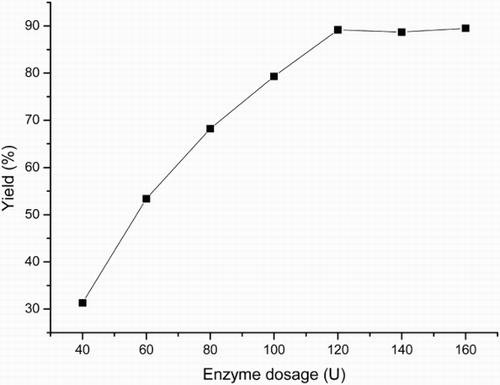
Water can influence the performance of enzyme in organic solvents apart from the organic solvent itself. The catalytic efficiency of an enzyme may depend on the hydration degree of the enzyme because it affects the microenvironment of the active site in the enzyme and cause the enzyme conformation to undergo a tremendous change (Citation28). The effect of water content in i-propanol was thus examined and the results are plotted in . The yield of the three-component reaction was increased with water content from 0% to 15% (water/i-propanol, v/v) and then decreased dramatically thereafter. This result suggested that the hydration-induced change of conformational flexibility could alter catalytic activity of lipase. Therefore, 15% was selected as the optimal water content for this reaction.
Figure 3. Effect of water content on the three-component reaction.
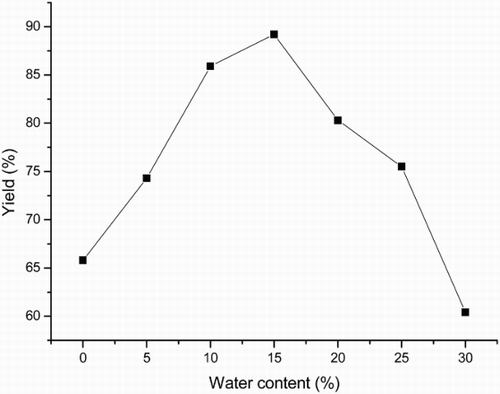
Subsequently, the substrate scope and the generality of the lipase-catalzyed three-component reaction were investigated under the optimal reaction conditions. Various aromatic aldehydes were selected for the synthesis of dihydropyrano[4,3-b]pyranes. As shown in , the reaction worked well (yields: 80.8–96.8%) with different aromatic aldehydes, and the aromatic aldehydes with election-withdrawing groups [1g–1l] gave higher yields than those with electron-donating groups [1b–1f]. It could also be found that the ortho-substituted benzaldehydes showed obviously lower activities than meta- and para-substituted benzaldehydes probably due to the steric hindrances. In addition, several aliphatic aldehydes (such as n-butyraldehyde, n-pentanal or n-hexaldehyde) were also investigated, but no products were detected using these reactants in this reaction.
Table 3. Synthesis of dihydropyrano[4,3-b]pyranes catalyzed by PPL with various aromatic aldehydes.
Experimental
Materials
Porcine pancreas lipase (PPL, lipase type II) was purchased from Sigma (Beijing, China). Pseudomonas sp. lipase (PSL) was purchased from Amano Pharmaceutical Co. Ltd. (Japan). Candida antarctica lipase B (CAL-B) was kindly donated by Novo Nordisk Industries (China). Bacillus subtilis lipase (BSL2) was over expressed from B. subtilis strain IFFI10210 in our laboratory according to the method we have reported [Biotechnol. Appl. Biochem., 2010, 56: 1–6]. Candida sp. lipase (CSL) was purchased from Beijing CTA New Century Biotechnology Co., Ltd (Beijing, China). One unit of the enzyme activity was defined as the amount of enzyme required to hydrolyze 1 μmol of p-nitrophenyl acetate per minute at 30°C. These enzymes were used after lyophilization for enzymatic reaction without further purification. 4-Hydroxy-6-methyl-2H-pyran-2-one and aromatic aldehydes were purchased from J&K Scientific (Beijing, China). Other reagents were purchased from Shanghai Chemical Reagent Company (Shanghai, China). Commercially available reagents and solvents were used without further purification. NMR spectra were recorded on an Inova 500 (500 MHz) spectrometer. ESI-MS was performed on an Agilent 1100 LC/MSD.
General procedures for the synthesis of dihydropyrano[4,3-b]pyranes
PPL (120 U) was added to a round-bottom flask containing aromatic aldehyde (1 mmol), malononitrile (1 mmol), 4-hydroxy-6-methyl-2H-pyran-2-one (1 mmol) and i-propanol (5 mL). The suspension was maintained at 60°C for 10 h. After completion of the reaction (monitored by TLC), the reaction mixture was concentrated under vacuum. The residue was washed with water and cold diethyl ether three times to remove unreacted starting materials and other organic contaminations, and then the filter cake was recrystallized from 95% ethanol to give products 4 with high purity. The experiments were performed triplicate, and all data were obtained based on the average values. The products were characterized by NMR experiments and MS.
Conclusion
In summary, this work illustrated that lipase could be used for the synthesis of dihydropyrano[4,3-b]pyranes via a three-component reaction. PPL is found to exhibit the highest catalytic performance under the optimal reaction conditions. This study provides a green and efficient method for the synthesis of dihydropyrano[4,3-b]pyranes. Moreover, it added a new case of lipase catalytic promiscuity and extended the application of lipase in green MCRs.
Notes on contributors
Xiao Chen is studying as a master candidate in School of Life Sciences, Jilin University. Her research has focused on the synthesis and development of new drugs.
Weian Zhang is studying as a master candidate in School of Life Sciences, Jilin University. His research has focused on the synthesis of bioactive compounds.
Fengjuan Yang is studying as a doctoral candidate in School of Life Sciences, Jilin University. Her research has focused on the biocatalysis and biotransformation.
Chao Guo is an undergraduate in School of Life Sciences, Jilin University. Guo is studying on the enzyme catalytic promiscuity supported by the Undergraduate Innovation Fund of Jilin University.
Ziyuan Zhao is an undergraduate in School of Life Sciences, Jilin University. Zhao is studying on the enzyme catalytic promiscuity supported by the Undergraduate Innovation Fund of Jilin University.
Di Ji is working as a biology teacher in the first middle school in Changchun City. Now, she is studying in Key Laboratory of Molecular Enzymology and Engineering of Ministry of Education (Jilin University, Changchun, China) for the synthesis and development of chiral drugs.
Fan Zhou is an undergraduate in School of Life Sciences, Jilin University. Zhou is studying on the enzyme catalytic promiscuity supported by the Undergraduate Innovation Fund of Jilin University.
Zhi Wang was born in 1973. He was obtained his Ph. D degree in 2003. Recently, her research has focused on the enzymatic resolution of chiral compounds.
Rui Zhao is an associate professor in-charge of department of urology, China-Japan Union Hospital of Jilin University, China. His research has focused on the synthesis and biological evaluation of bioactive compounds, green chemistry and medicinal chemistry.
Lei Wang is working in Key Laboratory of Molecular Enzymology and Engineering of Ministry of Education (Jilin University, Changchun, China) as a professor. Recently, his research has focused on the new synthetic methodologies to synthesize bioactive compounds.
Supplement_Material.docx
Download MS Word (1.8 MB)Disclosure statement
No potential conflict of interest was reported by the authors.
Additional information
Funding
References
- Dömling, A.; Ugi, I. Angew. Chem. Int. Ed. 2000, 39, 3168–3210.
- Tietze, L.F.; Modi, A. Med. Res. Rev. 2000, 20, 304–322.
- Wessjohann, L.A.; Rivera, D.G.; Vercillo, O.E. Chem. Rev. 2009, 109, 796–814.
- Li, C.J. Chem. Rev. 2005, 105, 3095–3166.
- Ranu, B.C.; Banerjee, S. Tetrahedron Lett. 2007, 48, 141–143.
- Banerjee, S. New J. Chem. 2015, 39, 5350–5353.
- Banerjee, S.; Santra, S. Tetrahedron Lett. 2009, 50, 2037–2040.
- Yang, F.J.; Wang, H.R.; Jiang, L.Y.; Yue, H.; Zhang, H.; Wang, Z.; Wang, L. RSC Adv. 2015, 5, 5213–5216.
- Xu, J.C.; Li, W.M.; Zheng, H.; Lai, Y.F.; Zhang, P.F. Tetrahedron 2011, 67, 9582–9587.
- Yang, F.J.; Wang, Z.; Zhang, X.W.; Li, Y.Z.; Wang, L. Chem. Cat. Chem. 2015, 7, 3450–3453.
- Li, K.; He, T.; Li, C.; Feng, X.W.; Wang, N.; Yu, X.Q. Green Chem. 2009, 11, 777–779.
- Yang, F.J.; Zhang, X.W.; Li, F.X.; Wang, Z.; Wang, L. Green Chem. 2016, 18, 3518–3521.
- Bora, P.P.; Bihani, M.; Bez, G. J. Mol. Catal. B-Enzym. 2013, 92, 24–33.
- Bora, P.P.; Bihani, M.; Bez, G. RSC Adv. 2015, 5, 50597–50603.
- Bihani, M.; Bora, P.P.; Verma, A.K.; Baruah, R.; Boruah, H.P.D.; Bez, G. Bioorg. Med. Chem. Lett. 2015, 25, 5732–5736.
- Kłossowski, S.; Wiraszka, B.; Berłożecki, S.; Ostaszewski, R. Org. Lett. 2013, 15, 566–569.
- Wang, J.L.; Liu, B.K.; Yin, C.; Wu, Q.; Lin, X.F. Tetrahedron 2011, 67, 2689–2692.
- Kostova, I.; Manolov, I.; Nicolova, I.; Konstantonov, S.; Karaivanova, M. Eur. J. Med. Chem. 2001, 36, 339–347.
- Zhao, H.; Neamati, N.; Hong, H.; Mazumdar, A.; Wang, S.; Sunder, S.; Milne, G.W.A.; Pommier, Y.; Burke, T.R. J. Med. Chem. 1997, 40, 242–249.
- Manolov, I.; Maichle-Moessmer, C; Danchev, N. Eur. J. Med. Chem. 2006, 41, 882–890.
- Ballini, R.; Bosica, G.; Conforti, M.L.; Maggi, R.; Mazzacani, A.; Righi, P.; Sartori, G. Tetrahedron 2001, 57, 1395–1398.
- Saha, A.; Payra, S.; Verma, S.K.; Mandal, M.; Thareja, S.; Banerjee, S. RSC Adv. 2015, 5, 100978–100983.
- Jin, T.S.; Xiao, J.C.; Wang, S.J.; Li, T.S.; Song, X.R. Syn Lett 2003, 35, 2001–2004.
- Ren, Y.; Cai, C. Catal. Commun. 2008, 9, 1017–1020.
- Kumar, B.S.; Srinivasulu, N.; Udupi, R.H.; Rajitha, B.; Reddy, Y.T.; Reddy, P.N.; Kumar, P.S. J. Heterocycl. Chem. 2006, 43, 1691–1693.
- Yang, F.J.; Zhang, X.W.; Li, F.X.; Wang, Z.; Wang, L. Eur. J. Org. Chem. 2016, 2016, 1251–1254.
- Liu, S.Y.; Xiong, G.H.; Gao, J.L.; Li, F.X.; Wei, S.S.; Wang, Z.; Wang, L. Green Chem. Lett. Rev. 2016, 9, 190–195.
- Ungcharoenwiwat, P.; H-Kittikun, A. J. Mol. Catal. B: Enzym. 2015, 115, 96–104.