ABSTRACT
Aristolochic acid-I (AA-I) is commonly present as a natural product in medicinal plants such as Asarum and Radix aristolochiae. The misuse of some slimming products and dietary, supplements containing AA-I as a regulator has been reported to cause cancer, acute hepatitis and renal failure. Hence, quality control and quantification of AA-I in these products at a trace level are significantly necessary. In this approach, a simple and accurate sequential injection analysis (SIA) chemiluminescence (CL) detection method was employed for AA-I determination. Gold nanoparticles were used to enhance the CL signal of luminol-ferricyanide-AA-I reaction. The results showed that the proposed method displayed linear relationship of 10–20,000 ng mL−1, (r = 0.9992) with 10 and 3 ng mL−1 as the minimum quantification and detection limits, respectively. The possible interferences such as some common metals, additives and related pharmacological action compounds were tested. The recorded SIA-CL results were statistically assessed and compared to those obtained from other published methods.
GRAPHICAL ABSTRACT
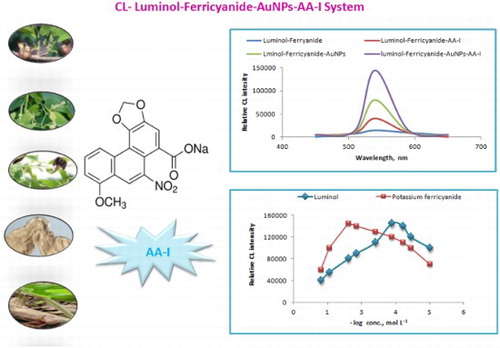
1. Introduction
Aristolochic acid-I (AA-I) is chemically known as 8-methoxy-6-nitrophenanthro [3,4-d]-1,3-dioxole-5-carboxylic acid (). It is one of the substituted 1-phaenanthrene carboxylic acids, a family of carcinogenic, mutagenic and nephrotoxic compounds commonly found in medicinal plants such as Aristolochia and Asarum. Many people believe that the use of natural herbs for treatment is safe and effective. Historically, Aristolochia had been used extensively in various cultures as a popular herbal remedy for different treatments, such as childbirth, joint pain and weight loss ( Citation1). Despite the widespread belief that the herbal remedies are not drugs because of their natural character, extensive research has confirmed that herbal remedies and weight loss products, which are commonly used in several countries in Asia as well as the U.S. contain AA-I. This AA-I not only causes kidney damage ( Citation2) but also, a mean reason for upper urinary tract cancer ( Citation3). These findings encouraged the U.S. Food and Drug Administration (FDA) to warn against using any traditional medicine containing AA-I ( Citation4).
Due to the high risk of consuming these herbal products, several methods have been developed for the determination of AA-I. Wang et al. ( Citation5) described a new high-performance liquid chromatography (HPLC) method coupled with fluorescence detector for sensitive detection of AA-I and aristolochic acid II (AA-II) in Chinese herbal medicine. The limits of detection were found to be 27.1 and 25.4 ng g−1, respectively. Also, Zhang et al. ( Citation6) proposed a simple chromatographic method with electrochemical detection for the determination of AA-I and II in medicinal plants using −0.8 V over the limits of detection 3.7 and 3.4 ng mL−1, respectively. An RP-HPLC method was conducted by Murali et al ( Citation7) to determine the AA-I in plant samples from different species. The highest concentration was found to be 9.9 ± 0.09 µg g−1 for the A. krisagathra species. Furthermore, Yang et al. ( Citation8) developed a simple hollow-fiber liquid phase microextraction coupled with an HPLC system to quantify the AA-I in human urine. The method provided excellent repeatability with a limit of detection 0.01 µg L−1. Other chromatographic methods coupled with mass spectrometry ( Citation9, Citation10) were described to determine AA-I in rat plasma and exfoliated urothelial cells with detection limits of 0.4–600 and 1.0 ng mL−1, respectively. Also, a more recent chromatographic method coupled with mass spectrometry was published by Yu et al. ( Citation11) for the detection of AA-I in different Aristolochia species. In addition to the previously summarized chromatographic methods, a capillary zone electrophoresis (CZE) method using two different types of detectors was reported by Xiaofang et al. ( Citation12). The described CZE method displayed an outstanding detection of AA-I in Chinese medicine with detection limits of 0.6 and 0.05 µg mL−1 using CZE-UV and CZE-MS detectors, respectively. Furthermore, the literature survey reported a spectroscopic technique based on fluorescence analysis for the detection of AA-I derived DNA adducts in renal tissues ( Citation13). Finally, an electrochemical detection method based on square wave voltammetry was introduced to detect AA-I in Chinese medicinal plants with a limit of detection of 1.6 × 10−8 mol L−1 ( Citation14).
Nowadays, many chemiluminescence (CL) methodologies based on flow approaches have been effectively developed ( Citation15, Citation16). Furthermore, the addition of nanomaterials in CL systems impacts the detection performance by enhancing the CL signals ( Citation17–Citation19). One recently developed technique is the CL based Sequential Injection Analysis (SIA-CL). Due to its simplicity, sensitivity, accuracy and reliability in the determination of different substances, researchers have used it extensively, especially for nano-scale detection ( Citation20, Citation21). Because of their chemical stability and high resistance to surface oxidation, gold nanoparticles (AuNPs) are one of the most attractive nanomaterials that can be used to catalyze CL reactions ( Citation22, Citation23). Green analytical chemistry is defined as the collaboration of efforts to develop cleaner or more eco-friendly techniques characterized by eliminating or at least minimizing emission of pollutants into the environment without compromising efficiency. Accordingly, a greener analytical process maybe achieved by avoiding the usage of toxic reagents, reducing the usage of chemical reagents, the miniaturization of the analytical instruments and introducing new technologies such as the nanotechnology ( Citation24). The green synthesis of AuNPs using eco-friendly substances such as natural materials has received great attention in analytical chemistry and pharmaceutical analysis ( Citation25, Citation26). The present study demonstrates employing the green synthesis of AuNPs using natural Mentha longifolia and Mentha pulegium plant extracts as a luminol-ferricyanide CL system enhancer. Based on this point of view, a new and sensitive SIA-CL system using luminol-potassium ferricyanide in the presence of AuNPs was proposed for detecting AA-I in medicinal plant materials as well as commercially sold slimming products.
2. Experimental
2.1. Chemicals and reagents
Sigma-Aldrich (Hamburg, Germany) provided a pure grade of AA-I (97% purity), the standard solution containing AA-II, luminol, hydrogen peroxide, methanol, ethanol, menthol 99%, menthone 90%, pulegone 97%, piperitone 98%, carvacrol acetate 99% and chloroauric acid HAuCl4. Commercial slimming product (Guang Fang Ji) containing AA-I (Aristolochia fangchi) was available from local drug stores. Sodium hydroxide, potassium periodate, potassium permanganate, potassium ferricyanide and sodium citrate dehydrate were purchased from WINLAB (East Midlands, UK). Five different samples of herbal medicines named Aristolochia fangchi (root), Aristolochia contorta (herb), Aristolochia debilis (Radix herb and fruit), Aristolochia mandshuriensis (stem) and Asarum (rhizome) were imported from California, U.S.A. Natural plant leaves (M. longifolia and M. pulegium) were purchased from the local market of Al-Madinah city, Saudi Arabia. A mobile phase composed of 0.1% formic acid and methanol (50:50, v/v) was used for separating AA-I and AA-II from medicinal samples.
2.2. Apparatus
The applied instrument for CL detection was the FIAlab-3500 system. This system is composed of a Vici Valco Cheminer RT® 125-0718 eight-port manifolds, a syringe pump with 2.5 mL volume model-CAVRO XL 3000 (Cavro Scientific Instrument Int., U.S.A.) connected to an ALM-3200 Autosampler, and a photomultiplier tube voltage of 320 V. FIAlab Windows software (version 5.9.321) was used for data collection. The separation of AA-I and AA-II from the natural samples was carried out using silica gel F254 thin layer chromatography (TLC) plate . The characterization of the synthesized AuNPs was performed using transmission electron microscope (TEM) JEOL model 1200EX operated at an accelerating voltage of 100 kV and 20,000× magnification. The ultrospec-2100 pro, ultra-visible spectrophotometer, was used to detect the ultraviolet (UV) absorption spectrum of the prepared nanoparticles.
2.3. Sample preparation
2.3.1. Preparation of AA-I standard solution
An AA-I stock standard solution (100 µg mL−1) was prepared by dissolving 10 mg of pure AA-I sodium salt in 100 mL distilled water. Serial solutions were prepared daily by appropriate dilution. The employed working solution was in the range of 10–20,000 ng mL−1.
2.3.2. Medicinal plants real samples
For the preparation of the real samples, dried samples of the medicinal plants were finely ground. Approximately 0.5 g of each plant sample was marinated in 10 mL methanol for 16 h. The obtained samples were ultrasonicated for 25 min then centrifuged at 3000 rpm for 5 min. The extracts were combined after 3× extraction, diluted, filtered using 0.45 μm Millipore membrane filter and 20 mL methanol was used to complete the volume. The possible interference of AA-II in the real samples was eliminated by its separation on TLC silica gel F254 using formic acid:methanol (50:50, v/v) and was detected densitometrically at 254 nm. The final pure solution was subjected to SIA-CL analysis in the presence of AuNPs to identify the AA-I content.
2.3.3. Preparation of commercial slimming product samples
For the preparation of the commercial slimming product sample, the herbal product (Guang Fang Ji) was finely ground, and approximately 10 g of the powder was dissolved in 50 mL methanol. The solution was ultrasonicated for 25 min and centrifuged at 3000 rpm for 5 min. The obtained solution was filtered using 0.45 μm Millipore membrane filter, and 50 mL methanol was used to complete the volume. The working solutions in the concentration range of 10–20,000 ng mL−1 were prepared by serial dilutions using the same solvent.
2.4. Synthesis of gold nanoparticles
2.4.1. Synthesis of AuNPs using natural plant extract
The plant extract of natural leaves (M. longifolia and M. pulegium) was obtained by washing the leaves with distilled water and drying them for 2 days at room temperature. The dried leaves were ground to fine powder and sieved carefully to remove coarse particles. Approximately 1 g of the fine leaf powder was marinated in 100 mL ethanol for 1 day and centrifuged at 2500 rpm for 30 min. The extract was filtered, and the filtrate was employed for AuNPs synthesis. Aliquots of the ethanolic water leaf extract were mixed with 1.0 × 10−3 mol L−1 HAuCl4 in ratios equivalent to 2–10% (v/v). The prepared solution was magnetically stirred at ambient temperature 25 ± 1°C, and the color of the solution was changed to pink indicating the formation of AuNPs. The AuNPs were characterized using TEM and UV spectrophotometric detection as shown in (a,b).
2.5. Procedure
To ensure the precise timing and valve movement of the system, the pump was automatically controlled. A mixture of 50 µL of 2.0 × 10−4 moL L−1 luminol, 30 µL of the prepared AuNPs, 50 µL sample solution and 30 µL of potassium ferricyanide (1.0 × 10−2 mol L−1) was aspirated at a 100 µL s−1 flow rate. The contents of the holding coil were flushed to waste by switching the valve to the waste port and operating the pump in the forward direction. The valve was switched to the detector port and the reagent and test solution zones were flushed to the flowthrough cell by the pump operating in the forward mode. The emission from the resultant reaction was monitored from the flowthrough cell by the Photon Counting Photomultiplier Tube (PMT) via the fiber optic cable. Three analysis cycles were carried out per test solution, with the average CL intensity being used for determination or calibration.
2.6. Calibration graph
The calibration graph for the determination of AA-I was constructed under optimum experimental conditions. The graph was plotted using CL intensity against the tested compound concentrations by triplicate sample aspiration and the mean peak heights were calculated. Concentrations were obtained using the conventional linear regression.
3. Results and discussion
3.1. Optimization of the CL conditions
One of the most important parameters that greatly affect the CL reaction is the selection of a suitable oxidizing agent. Different oxidants were tested, including hydrogen peroxide, potassium ferricyanide, potassium periodate and potassium permanganate. The recorded signals indicated that both potassium ferricyanide and hydrogen peroxide showed CL signals. However, potassium ferricyanide provided sharper and higher CL signals than hydrogen peroxide. Poor CL signals were recorded when potassium permanganate and potassium periodate were used (). Therefore, the selected system for further studies was luminol-potassium ferricyanide CL system.
The adjustment of potassium ferricyanide and luminol concentrations was carried out using various concentrations in the range of 1.0 × 10−5–1.0 × 10−1 mol L−1 for both reagents. CL intensity showed a significant increase at 1.0 × 10−2 and 2.0 × 10−4 mol L−1 for potassium ferricyanide and luminol, respectively (). The added volume of AuNPs solution can greatly affect the CL intensity of the luminol-ferricyanide system. Thus, AuNPs volume was investigated over the range of 0.5–4 mL. As shown in , the CL intensity was sharply increased when 1.5 mL of AuNPs was used. Hence, this volume was selected to advance the study.
Figure 4. Effect of luminol and potassium ferricyanide concentration on CL intensity, for luminol concentration (1.5 mL of AuNPs solution and potassium ferricyanide 1.0 × 10−2 mol L−1) and for potassium ferricyanide concentration (1.5 mL of prepared AuNPs and luminol 2.0 × 10−4 mol L−1) with AA-I 25 g mL−1.
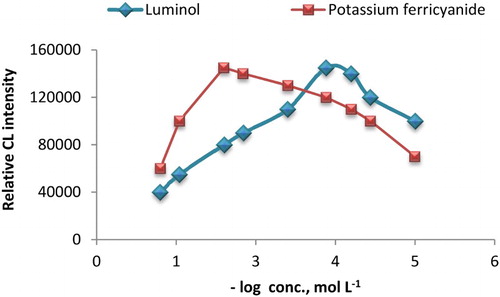
The effect of alkaline media was also investigated. As shown in , it was found that the use of 1.0 × 10−2 mol L−1 sodium hydroxide of pH = 10 gave a sharp CL signal while in the cases of ammonium hydroxide, sodium carbonate and sodium bicarbonate, a significant decrease in CL signal was recorded.
Figure 6. Effect of sodium hydroxide, ammonium hydroxide, sodium carbonate and sodium bicarbonate concentration on CL intensity of luminol-potassium ferricyanide system (1.5 mL of AuNPs, potassium ferricyanide 1.0 × 10−2 mol L−1 and luminol 2.0 × 10−4 mol L−1).
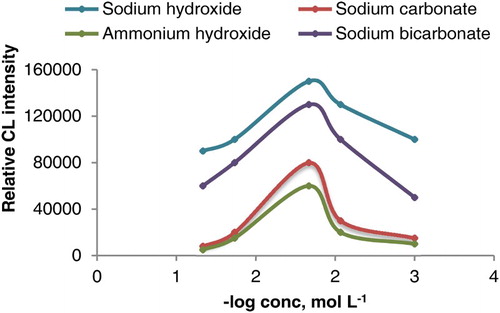
The aspirated volume of samples and reagents was controlled and accurately optimized. The optimum aspirated volume for luminol, AuNPs and potassium ferricyanide was 50, 30 and 30 μL, respectively and 50 μL for the AA-I sample. The time needed to complete flushing through the holding cell with carrier between analysis cycles was 45 s. Also, the effect of flow rate on CL intensity was investigated in the range of 20–140 μL s−1. The optimum flow rate was found to be 100 μL s−1 and it was selected for the further investigation ().
3.2. SIA control program
To utilize the simple and accurate sequential injection analysis (SIA) control program for performing all calibration measurements and experimental analysis of AA-I, the proposed program was employed for the determination of the tested compound in its standard solution, real samples and slimming products. The single cycle takes approximately 45 s. Therefore, the sample throughput of 80 sample h−1 can be recorded ().
Table 1. The control program of AuNPs-luminol-potassium ferricyanide SIA-CL detection of AA-I.
3.3. Analytical characteristics
The enhanced AuNPs-luminol-ferricyanide system was employed to determine AA-I. The presented results in clarified that the measurable linear concentration range was 10–20,000 ng mL−1, (r = 0.9992, n = 12) with a lower limit of detection of 5 ng mL−1 and a quantification limit of 10 ng mL−1. The regression parameters were calculated from the calibration graph, and the reproducibility of the proposed method was tested using 10–20,000 ng mL−1 test solutions. The relative standard deviations were less than 5% indicating that the suggested method was suitable for routine analysis of the investigated compound.
Table 2. Performance data obtained from the determination of AA-I using AuNPs-luminol-potassium ferricyanide system.
3.4. The mechanism of AuNPs-luminol CL enhancement
In the present study, the optimum CL reaction conditions were accomplished using 1.0 × 10−2 mol L−1 potassium ferricyanide with 2.0 × 10−4 mol L−1 luminol in alkaline medium 1.0 × 10−2 mol L−1 sodium hydroxide of pH = 10. It has been previously proven that different CL systems could be enhanced in the presence of AuNPs ( Citation27, Citation28). To investigate the possible reaction mechanism of AuNPs-CL enhancing phenomenon, comparative CL spectra of the luminol-potassium ferricyanide-AuNPs-AA-I reactions were recorded and demonstrated in . The reaction mechanism of oxidation of luminol is believed to involve the superoxide radical O-O•− or hydroxyl radical OH• as important intermediates leading to luminescence. In the absence of AuNPs, the reaction of luminol with potassium ferricyanide in alkaline medium showed weak CL emission. Meanwhile, using AuNPs under the previously mentioned optimum conditions produced a significant sharp CL signal revealing the catalytic activity and enhancing effect of AuNPs. This effect suggests that the active surface of AuNPs facilitates the oxidation process of luminol and the formation of superoxide intermediate radicals, which have an enhancing effect on the CL signal.
Figure 8. Comparative CL signals using luminol-ferricyanide, luminol-ferricyanide-AA-I, luminol-ferricyanide-AuNPs and luminol-ferricyanide-AuNPs-AA-I: Optimum conditions: 50 µL of 2.0 × 10−4 mol L−1 luminol; 30 µL of AuNPs and 30 µL of 1.0 × 10−2 mol L−1 potassium ferricyanide and 50 µL sample AA-I.
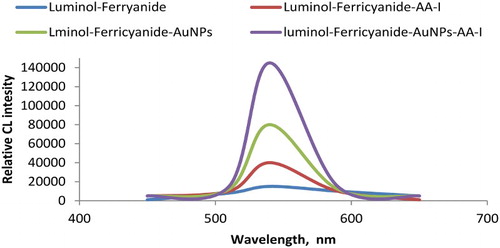
The following mechanism showed the CL reaction of luminol-ferricyanide in the presence of AuNPs (Scheme 1).
To our knowledge, the catalytic effect of AuNPs on the luminol-ferricyanide CL reaction is particle size dependent. If the prepared AuNPs are less than 5 nm, the CL reaction of luminol and ferricyanide will be quenched due to the high surface energy produced from the small particles which will cause high redox activity. Meanwhile, the particle size of AuNPs more than 10–100 nm will enhance the CL reaction due to the change in oxidation state of AuNPs and the catalytic enhancement involved in the process of electron transfer ( Citation29). The CL reaction of luminol with hydrogen peroxide was also enhanced in the presence of AuNPs due to the breaking down of hydrogen peroxide to superoxide. The produced superoxide form activated luminol ions on the AuNPs surface. The light was emitted when the activated luminol ion returned to its ground state. The recorded CL signal in the later reaction was lower than in the case of luminol-ferricyanide. Therefore, we considered the luminol-ferricyanide system to complete our investigation.
3.5. Effect of foreign substances
The selectivity of the method is defined as the ability of the proposed method to discriminate the analytes from all potential interfering species. Some foreign substances such as common cations, some additive excipients, amino acids, sugars and some related pharmacological action compounds such as AA-II were tested. Also, the selectivity of the developed method for detecting AA-I in the presence of some active constituents of M. longifolia and M. pulegium such as menthol, menthone, pulegone, piperitone, carvacrol acetate was investigated. The evaluation of the interferences was carried out using a test solution of 25 ng mL−1of AA-I in the presence of various concentrations of foreign substances. The tolerable level was defined as the amount of foreign species which produce an error that does not exceed 5%. shows the obtained results of tolerated concentration level for the studied interferences. It can be observed that no influence on the determination of AA-I in slimming products was obtained. For real samples, the main possible interference was from the related medicinal compound AA-II. The latter was eliminated using simple separation on a TLC silica gel F254 using formic acid: methanol (50:50, v/v) and was detected densitometrically at 254 nm.
Table 3. Tolerable concentration level of interference to 25 ng mL−1 AA-I.
3.6. Method validation
The appropriate validation of analytical methods has become an essential part of any successful method development and characterization. Validation of a method involves using an experimental design to prove that the method can produce accurate and precise results within the scope of its intended use. Understanding the application and limitations of the test method allows for accurate assessment of sample information, ranging from process outputs to commercial release testing and many steps in between ( Citation30). Linearity, the lower limit of detection, quantification limit, accuracy, precision and robustness were performed to validate the method according to International Council for Harmonization (ICH) guidelines ( Citation31).
3.6.1. Linearity
The linearity of the proposed enhanced AuNPs-SIA-CL method for the determination of AA-I using the luminol-ferricyanide system was successfully evaluated. The least square statistical method was applied to calculate the regression line. The obtained results showed that the proposed SIA-CL method exhibited a linear relationship over a concentration range of 10–20,000 ng mL−1.
3.6.2. Limits of detection LOD and quantification LOQ
Signal-to-noise ratio was performed to evaluate the lower limit of detection of the proposed SIA-CL method for determining AA-I. The lower limit of detection was calculated by S/N = 2 as the concentration of AA-I has a CL signal equals two times that of the blank signal. The limit of detection was found to be 3 ng mL−1.
In order to determine the quantification limit of AA-I by the proposed AuNPs-luminol-ferricyanide SIA-CL method, signal-to-noise ratio equals to 10 was performed for such evaluation and the quantification limit was 10 ng mL−1.
3.6.3. Accuracy and precision
The accuracy of the proposed AuNPs-luminol-ferricyanide SIA-CL method was carried out by investigating the tested compound using the standard addition method. It was found to be 99.25 ± 1.4% as mean percentage recovery. To evaluate the precision of the proposed method, the terms intra-day and inter-day were employed. The studies were carried out by repeating the determination to nine replicates. The calculated % RSD values were listed in for AA-I detection. The obtained % RSD value is less than 2% revealing high precision.
Table 4. Intra-day and inter-day precision using enhanced AuNPs-SIA-CL method for detection of AA-I.
3.6.4. Robustness
The robustness of the SIA-CL method for the determination of AA-I was investigated by introducing small changes to method parameters. In the proposed study, the robustness of the method was carried out by changing the flow rate, aspirate rate or reagents and the volume of the sample using 100 ± 10 µL s−1, 50 ± 5 µL and 30 ± 5 µL, respectively. The calculated recovery % for the proposed method was 99.5 ± 0.9% indicating excellent agreement with those obtained from standard solutions.
3.6.5. Analysis of AA-I in slimming products and real samples
It was evident from the obtained results that the proposed method showed excellent results for the determination of AA-I in the standard solution. Thus, its medicinal products, such as the slimming product (Guang Fang Ji), were subjected to the analysis of its AA-I content by the proposed enhanced AuNPs-SIA CL-injection method. The recorded results were presented in and were statistically compared by Student's t-test and F-test ( Citation32) with those obtained from the reported liquid chromatography-mass spectrometry method ( Citation9). This method was carried out using Zorbax Eclipse XDB-C18 by isocratic elution of methanol: ammonium acetate (75:25 v/v, pH = 7.3) at flow rate 0.25 mL min−1 and the detection was mass spectrometry adjusted in positive multiple reaction monitoring mode.
Table 5. Determination of AA-I am using AuNPs-luminol-potassium ferricyanide SIA-injection CL detection in pure solution and medicinal products.
The percentage recovery in pure samples was found to be 99.4 ± 0.6 and did not reveal any significant difference from those obtained by the reference method (98.6 ± 0.9) at 95% confidence level proving similar accuracy and precision. The extremely high sensitivity of the proposed method promoted the check of its applicability to determine AA-I in its real samples. The results were presented as the mean % content and % RSD and were confirmed by the results obtained from the reported HPLC method ( Citation9), which indicated a good agreement and no significant difference as shown in .
Table 6. Detection results of AA-I in five medicinal plants using AuNPs enhanced SIA-CL method (n = 6) and confirmed by reported HPLC method ( Citation9).
4. Conclusion
The present study introduced a new simple and reliable sequential injection analysis SIA CL technique based on the enhancement effect of AuNPs on luminol-potassium ferricyanide system that has been developed to determine AA-I. The literature survey revealed that CL methods had not been reported for detecting AA-I. Due to the high sensitivity and reproducibility of the proposed method it was successfully applied for the detection of AA-I in different species of medicinal plants and its slimming product.
Acknowledgments
The authors would like to extend their gratitude to Laval University, Zagazig University and King Saud University for providing the working materials as well as suitable, well-equipped and collaborative working environments.
Disclosure statement
The authors declare this work was done by the authors named in this article and all liabilities pertaining to claims relating to the content of this article will be borne by them.
Notes on contributors
Hesham F. Oraby is an Assistant professor of plant biotechnology and molecular biology who received his Ph.D. from Michigan State University, USA in 2006. His research focuses on plant tolerance to biotic and abiotic stresses, and compounds with anticancer and antioxidant activities.
Nawal A. Alarfaj is a professor of Analytical Chemistry at King Saud University, Riyadh, Saudi Arabia. She received her PhD and Master's degree in Analytical Chemistry from King Saud University, KSA in 1999. Her research is concerned with analysis of drugs and environmental samples by luminescence and electrochemical methods.
Maha F. El-Tohamy is currently a Researcher in Department of Chemistry, Faculty of Science, King Saud University. The author received her Ph.D. Pharmaceutical Sciences from Al-Azhar University, Cairo Egypt in 2010, where her analytical research was towards the green chemistry to minimize the formation of hazardous compounds.
References
- Grollman, A.P.; Marcus, D.M. Global Hazards of Herbal Remedies: Lessons from Aristolochia. EMBO Rep. 2016, 17, 619–625. doi: 10.15252/embr.201642375
- Liu, X.; Liu, Y.; Cheng, M.; Xiao, H. Biomarkers. 2016, 21, 233–242. doi: 10.3109/1354750X.2015.1134660
- Margaret, L.; Chen, H.C.; Chen, P.C.; Roberts, N.J.; Dickman, K.G.; Yun, B.H.; Turesky, R.J.; Pu, Y.S.; Vogelstein, B.; Papadopoulos, N. et al. Cancer Epidemiol. Biomarkers Prev. 2016, 25, 1600–1608. doi: 10.1158/1055-9965.EPI-16-0219
- Food and Drug Administration FDA, Aristolochic acid, FDA Concerned about botanical products, including dietary supplements, containing aristolochic acid. U.S. 2000. http://www.fda.gov/Food/DietarySupplements/Alerts/ucm095302.
- Wang, Y.; Chan, W. J. Agric. Food. Chem. 2014, 62, 5859. doi: 10.1021/jf501609j
- Zhang, J.; Wei, Z.; Zhang, A.; Zhao, Y.; Zhao, G.; Cai, X.; Cui, W.; Wang, Y.; Fan, J. Int. J. Electrochem. Sci. 2016, 11, 6830. doi: 10.20964/2016.08.11
- Somya Murali, M.S.; Francis, T.R.; Rashmi, T.R. Indo Am. J. Pharm. Res. 2014, 4, 2449–2454. http://www.scopemed.org/?mno=162237
- Yang, Y.; Juan, C.; Yan-Ping, S. Biomed. Chromatogr. 2010, 24, 1350–1355. doi: 10.1002/bmc.1448
- Yiming, L.; Aihua, L.; Zhifeng, W.; Runmei, O.; Haiding, H. Biomed. Chromatogr. 2010, 24, 174–179. doi: 10.1002/bmc.1267
- Lin, G.; Hanzhi, W.; Hao, Y.; Shuhai, L.; Yongquan, L.; Zongwei, C. J. Chromatogr. B 2010, 879, 153–158. doi: 10.1016/j.jchromb.2010.11.035
- Yu, J.; Ma, C.M.; Wang, X.; Shang, M.Y.; Hattori, M.; Xu, F.; Jing, Y.; Dong, S.W.; Xu, Y.Q.; Zhang C.Y.; Cai, S.Q. Chin. J. Nat. Med. 2016, 14, 626–640. http://doi.org/10.1016/S1875-5364(16)30074-7
- Xiaofang, F.; Yi, L.; Wei, L.; Yu, B.; Yiping, L.; Huwei, L. Talanta. 2011, 85, 813–815. doi: 10.1016/j.talanta.2011.03.088
- Victor, R.; Victoria, S.; Rosenquist, T.A.; Terry, W.; Grollman, A.P. Anal. Biochem. 2012, 427, 49–51. doi: 10.1016/j.ab.2012.03.027
- MiSun, K.; Younhee, H. Arch. Pharm. Res. 2007, 30, 255–259. doi: 10.1007/BF02977702
- Vakh, C.; Koronkiewicz, S.; Kalinowski S.; Moskvin, L.; Bulatov, A. Talanta. 2017, 167, 725–732. doi: 10.1016/j.talanta.2017.02.009
- Asghar, M.; Yagoob, M.; Munawar, N.; Nabi, A. Anal. Sci. 2016, 32, 337–342. doi: 10.2116/analsci.32.337
- Li, Q.; Zhang, L.; Li, J.; Lu, C. TrAC Trends Anal. Chem. 2011, 30, 401–413. doi:10.1021/jp200711a doi: 10.1016/j.trac.2010.11.008
- Li, J.; Li, Q.; Lu, C.; Zhao, L. Analyst. 2011, 136, 2379–2387. doi: 10.1039/c0an00918k
- Li, Q.; Liu, F.; Lu, C.; Lin, J.M. J. Phys. Chem. C 2011, 115, 10964–10970. doi: 10.1021/jp200711a
- Oliveira, H.M.; Grand, M.M.; Ruzicka, J.; Measures, C.I. Talanta. 2015, 133, 107–111. doi: 10.1016/j.talanta.2014.06.076
- Fassoula, E.; Economou, A.; Calokerinos, A. Talanta. 2011, 85, 1412–1418. doi: 10.1016/j.talanta.2011.06.037
- Zhang, Z.F.; Cui, H.; Lai, C.Z.; Liu, L.J. Anal. Chem. 2005, 77, 3324–3329. doi: 10.1021/ac050036f
- Hasanpour, F.; Khayamian, T.; Ensafi, A.A.; Rahmani, H.; Razaei, B. Lumin. 2013, 28, 780–784. doi: 10.1002/bio.2438
- Anastas, P.T.; Warner, J.C. Green Chemistry: Theory and Practice; Oxford University Press: New York, 1998; p 30.
- Soshnikova, V.; Kim, Y.J.; Singh, P.; Huo, Y.; Markus, J.; Ahn, S.; Castro-Aceituno, V.; Kang, J.; Chokkalingam, M.; Mathiyalagan, R. ; et al. Artif Cells Nanomed Biotechnol. 2017, 3, 1–10. doi: 10.1080/21691401.2017.1296849
- Cabrera, G.F.; Balbin, M.M.; Eugenio, P.J.; Zapanta, C.S.; Monserate, J.J.; Salazar, J.R.; Mingala, C.N. Biochem Biophys Res Commun. 2017, 484, 774–780. doi: 10.1016/j.bbrc.2017.01.164
- Abideen, S.U. Chemiluminescent Reactions Catalyzed by Nanoparticles of Gold, Silver, and Gold/Silver Alloys. Master's Theses and Doctoral Dissertations. Eastern Michigan University, 2012, pp. 37, 40.
- Dong, U.P.; Gao, T.T.; Chu, X.F.; Wang, C. J. Lumin. 2014, 154, 350–355. doi: 10.1016/j.jlumin.2014.05.011
- Duan, C.; Cui, H.; Zhang, Z.; Liu, B.; Guo, J.; Wang, W. J. Phys. Chem. 2007, 111, 4561–4566.
- Bridwell, H.; Dhingra, V.; Peckman, D.; Roark, J.; Lehman, T. Qual. Assur. J. 2010, 13, 72–77. doi: 10.1002/qaj.473
- International Council for Harmonization (ICH). Technical Requirements for Registration of Pharmaceuticals for human use, complementary guidelines on methodology. Jones & Bartlett: Washington, DC, 1996, 13 p 281.
- Miller, J.C.; Miller, J.N. Statistics for Analytical Chemistry, 3rd ed.; Ellis Horwood-Prentice Hall: Chichester, 1996. http://trove.nla.gov.au/version/45464904