ABSTRACT
Hydrodesulfurization (HDS) waste catalyst is regulated as a hazardous waste because of its substantial metal content. HDS contains (wt.%) 33.4% Al, 6.8% Mo, 3.6% V, 1.5% Co and 0.7% Ni. Therefore, it must be treated for detoxification or for the recovery of these metals as secondary resources. In this work, recovery of metal ions using calix[4]resorcinarenes hydroxamic acid was investigated. The factors affecting the extraction method such as aqueous phase acidity, type of organic solvent, shaking time, the capacity of calix[4]resorcinarenes to extract vanadium, and the selectivity of V over the other metal ions were studied. The optimal conditions of extraction were found to be as follows: aqueous acidity of 6 M, ethyl acetate as the solvent, and shaking times of 15 min; the extraction of vanadium is effective with most of the vanadium selectively extracted with calix[4]resorcinarenes, whereas all other metal ions remain in the aqueous phase.
GRAPHICAL ABSTRACT
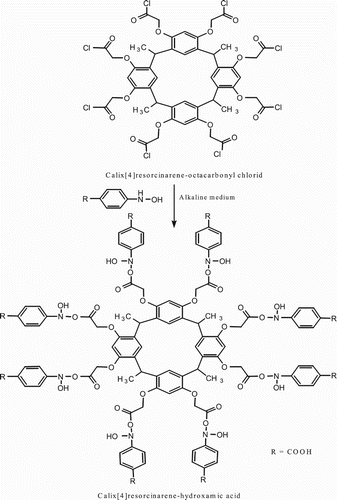
1. Introduction
The recovery of valuable metals from secondary resources has become of great importance. The spent hydrodesulfurization (HDS) waste catalysts are highly important resources for the recovery of metal value because they contain a certain amount of molybdenum, vanadium, cobalt and nickel together with an appreciable amount of aluminum and the concentration of the individual elements varying depending on the source of the HDS catalyst and the crude oil (Citation1). The HDS is a well-known catalyst, and its solid state synthesis and characterization have been reported in (Citation2). Mo and V are strategic elements that are widely used in chemical and aerospace industries. Recovery of these valuable metals from a spent HDS catalyst using various processes has been reported in (Citation3–6). There are two routes for the recovery of these valuable metals, namely the pyro route and the hydrometallurgical route. In the hydrometallurgical route, the spent HDS is leached by either acid or alkali solution after roasting (Citation3–5). On the other hand, in the pyro route, various techniques such as direct smelting (Citation7), calcination and smelting (Citation6), salt roasting (Citation8) and chlorination (Citation9) are used. Nevertheless, both routes suffer from drawbacks such as slow metal recovery kinetics and low recovery percentage of some valuable metals. The eight-armed calix[4]resorcinarene that bears eight hydroxamic acid groups such as p-carboxy-N-phenyl-calix[4]resorcinarene-hydroxamic acid, hereafter abbreviated as (CALIX), has been synthesized. Calix[4]resorcinarenes form an array of complexes with neutral molecules, organic molecules, metal ions, and organometallics depending on the chelating groups on the eight hydroxyl groups present at the extraannular position or on the upper rim (Citation10, Citation11). Usually, calixarenes are used for the identification of metal ions in different media. On the other hand, there have been few reports on the use of calix[4]resorcinarenes for the extraction of metal ions. Some studies have examined the complexation of calixarenes with alkali metal ions, especially potassium ions (Citation12, Citation13). Other studies reported that calixarene with hydroxamic acid functional groups was synthesized and used for the metal complexation studies (Citation14, Citation15). Recently, there also has been some research work on the extraction of the Nd(III) trivalent rare earth metal ions, univalent thallium cation, and americium using deferent derivatives of calix[4]arenes (Citation16–19). The results of these studies showed that calix[4]arene derivatives show excellent extraction ability and can be a potential extractant for disposal of radioactive waste in spent fuel. In this study, the eight-armed p-carboxy-N-phenyl-calix[4]resorcinarene-hydroxamic acid (CALIX) is synthesized and used for extraction of vanadium from spent HDS catalyst leach solution in the presence of a large number of ions. The effects of solvent, acidity and shaking time were studied.
2. Experiments
2.1. Supply and analysis of HDS catalyst sample
A sample of HDS waste catalyst utilized during this study was kindly donated by El Nasr Co. for Coke & Chemicals, Egypt. Metal contents were determined using a Siko model SAS7500 atomic absorption spectrophotometer. The chemical analysis of a sample of HDS waste catalyst was carried out as follows: an HDS waste catalyst sample was crushed to powder and roasted for 2 h at 700°C to remove sulfur, hydrocarbons, and carbon deposited on the sample and was then suspended in 62% sulfuric acid solution and heated to 130–200°C for vaporization to dryness. Subsequently, the sample was dissolved in water and heated at 100°C for 1 h. The final solution was filtered to eliminate the small amount of solid silicon dioxide and the filtrate was analyzed. This total dissolution method showed that the HDS catalyst has the following composition (wt.%): 33.4% Al, 6.8% Mo, 3.6% V, 1.5% Co and 0.7% Ni.
2.2. Chemical reagents and preparation of solution
All chemicals used were analytical grade reagents obtained from BHD or E-Merck. The vanadium-containing aqueous phase stock solution (810 ppm V, equivalent to 0.0159 M V) was prepared by dissolving 0.929 g of ammonium metavanadate (NH4VO3) in 500 cc of deionized water. The aqueous phase, which was a synthetic model solution of the leach liquor described above in (2.1), was prepared by dissolving analytical grade NiCl2, CoCl2, (NH4)6Mo7O24·4H2O, NH4VO3 and AlCl3 in deionized water. The acidity of the aqueous solution was adjusted using hydrochloric acid and water. The organic phase (CALIX) was synthesized from resorcinol using the method discussed elsewhere (Citation20). The stock solutions of CALIX (3 × 10−4 M) were prepared in ethyl acetate. Appropriate dilution of the stock solution was carried out when needed for the preparation of the experimental solution.
2.3. Liquid–liquid extraction procedure
Extraction tests were carried out batch-wise by shaking the aqueous solution containing the metal ions and organic phases in 50 cc flasks immersed in a regulated shaking water bath. Once the necessary time elapsed, the aqueous/organic mixture was poured in a 25 cc glass separating funnel. The organic extract was separated and transferred into a 10 cc flask. The metal content in each feed and equilibrium aqueous phase were determined after an appropriate dilution with deionized water. The metal ion concentration extracted into the organic phase as the complex was estimated by
where
is the initial concentration, and
is the equilibrium concentration within the aqueous phase.
The extraction percentage (E%) is calculated as .
2.4. Synthesis and characterization of calix[4]resorcinarene – hydroxamic acid
Synthesis of calix[4]resorcinarene-hydroxamic acids was carried out by coupling of calix[4]resorcinarene-acid chloride with hydroxylamines. This was enabled by the reaction of calix[4]resorcinarene bearing a carboxylic acid functional group with thionyl chloride to give the corresponding acid chloride, which then undergoes a further coupling with hydroxyl amines to give the hydroxamic acids compound denoted as CALIX. The general method for the synthesis of CALIX has been mentioned elsewhere (Citation20) and can be described as follows: Freshly prepared phenyl hydroxylamine (0.8 mol) in diethyl ether was taken in a 100 ml conical flask fitted with a dropping funnel. Then, an aqueous suspension of sodium bicarbonate (42 g, 0.5 mol) in water was added and stirred. After the mixture was cooled to −7°C, calix[4]resorcinarene octacarbonyl chloride compound (0.01 mol) in dioxane was added dropwise during a period of 50 min with the stirring continued for 3 h. The reaction mixture was then brought to room temperature, and the solvents were distilled under a vacuum to obtain the crude product CALIX. The product was then recrystallized from ethyl acetate-hexane medium to yield 50%; its chemical structure is shown in . The chemical composition of CALIX was confirmed by FT-IR and 1H NMR spectroscopy. The 1H NMR spectrum of CALIX showed peaks at δ 1.62 ppm (d, CHCH3), δ 3.16 ppm (q, CHCH3), δ 4.6 ppm (s, –O–CH2–CO–), δ 6.5–7.9 ppm (s, ArH), δ 8.88 ppm (s, N–OH) and δ 9.50 ppm (s, Ar–COOH). The FT-IR spectrum of CALIX showed characteristic absorption bands at 3184 cm−1 due to (O–H) stretching vibration, with the band at 1634 cm−1 assigned to the (C=O) of the hydroxamic acid group. The band at 1360 cm−1 is assigned to (C–N) vibration, and the band at 910 cm−1 is assigned to (N–O) vibration.
3. Results and discussions
3.1. Effect of aqueous acidity
The effect of aqueous phase acidity on the vanadium extraction from its individual synthetic solution using CALIX was examined. A series of experiments was performed at different hydrochloric acid concentrations ranging from 2 to 7.5 M. The results are shown in . The results reveal that the V extraction percentage was increased with increasing acidity of the aqueous phase up to 6–6.5 M and then started to decrease. This suggested that the optimum acidity is 6 M, and this acidity was used for all subsequent experiments.
3.2. Effect of solvent
The V-CALIX complex was examined in various solvents, such as ethyl acetate, chloroform, toluene and benzene. The results are shown in . Inspection of reveals that the optimal V extraction is obtained using ethyl acetate as solvent. This may be attributed to the fact that ethyl acetate exhibits the highest dielectric constant among the solvents listed in .
Table 1. Effect of different solvents on vanadium extraction %.
3.3. Effect of shaking time
The shaking time was changed in the range from 2 to 30 min. The optimum shaking time was found to be 15 min, and further shaking did not affect the quantitative extraction of vanadium as shown in .
3.4. Vanadium extraction capacity of CALIX
To measure the vanadium extraction capacity of CALIX, a series of experiments was carried out using the following solutions: an aqueous phase containing 810 ppm of V equivalent to 0.0159 M with the organic phase (CALIX) containing 3 × 10−4 M. By changing the O/A phase ratio, the number of moles of vanadium present can also be changed so that for the constant organic phase volume of 10 ml, the number of moles of CALIX in these experiments is 3 × 10−6 mol. The number of moles of V for different O/A phase ratios is listed in . shows that the V extraction percentage increases with increasing O/A phase ratio, and the optimum V extraction is obtained at 1 O/A phase ratio, which is equivalent to the CALIX to V mole ratio of 1.89 × 10−2.
Table 2. Study of the vanadium extraction capacity of CALIX.
3.5. Vanadium selectivity relative to other metal ions
The vanadium selectivity of CALIX can be practically determined by the extraction of vanadium from the solution containing other metal ions under the optimum extraction conditions. To evaluate the vanadium extraction selectivity of CALIX, the extract was carried out in the presence of the metal ions present in the solution produced from the HDS waste catalyst dissolution, as listed in . Oxalic acid (0.25 M C2H2O4) was added as a masking agent for molybdenum to prevent its extraction with the vanadium by CALIX. The complex produced between Mo and oxalic acid was reported in (Citation21). The extraction percentage is calculated as the difference between the concentration of the metal ion in the aqueous phase before and after shaking with CALIX. The results listed in show that all vanadium is selectively extracted with CALIX, while all other metal ions remain in the aqueous phase. The small extraction percentage of the other metal ions may be attributed to an experimental error in the measurements.
Table 3. Effect of metal ions on the extraction of vanadium (V-CALIX complex).
4. Conclusion
This work examined the recovery of vanadium as a valuable element from HDS waste catalyst. The different factors that affect the extraction process were studied. The obtained results reveal that
Calix[4]resorcinarenes are synthesized from resorcinol in good yield and act as a good extractant of vanadium from an HDS waste catalyst.
The presence of valuable elements (wt.%) 33.4% Al, 6.8% Mo, 3.6% V, 1.5% Co and 0.7% Ni in HDS waste catalyst makes it an attractive material for simultaneous metal recovery and detoxification.
Ethyl acetate is the optimal solvent for vanadium extraction. This may be due to the fact that the dielectric constant of ethyl acetate is higher than those of the other solvents investigated in this study.
The optimum shaking time for extraction was found to be 13–15 min.
All vanadium is selectively extracted with CALIX, while all other metal ions remain in the aqueous phase.
Acknowledgements
This work has been financially supported by the Deanship of Scientific Research, Hail University, KSA.(Project number 0150082). The authors extend their appreciation to the Deanship of Scientific Research, and to the Chemistry Department, Faculty of Science, Hail University for technical support.
Disclosure statement
No potential conflict of interest was reported by the authors.
Notes on contributors
Seham Nagib graduated with Ph.D. degree in applied chemistry from Saga University, Japan. She has carried out research projects in recycling of waste catalyst, and metal recovery. She has more than 25 years of teaching experience as a lecturer and associate professor in Egypt and the KSA. Seham has more than 35 research papers in various national and international journals. She is currently working as an associate professor of inorganic Chemistry at Hail University.
Reda S. Abdel Hameed graduated with a degree in chemistry from Al-Azhar University, Cairo, Egypt. He has carried out research projects in applied organic chemistry, physical chemistry, and green chemistry. He has more than 20 years of teaching experience as a lecturer and associate professor in Egypt and the KSA. Reda has more than 43 research papers in various national and international journals. He is currently working as an associate professor of applied Physical Chemistry at Al-Azhar University.
Additional information
Funding
References
- Zeng, I.; Cheng, C.Y. A Literature Review of the Recovery of Mo and V from Spent Hydrodesuphurization Catalysts: Part-1: Metallurgical Processes. Hydrometallurgy. 2009, 98, 1–9. doi: 10.1016/j.hydromet.2009.03.010
- Villa Garcia, M.A.; Lindner, J.; Sachdev, A.; Schwank, J. Solid State Synthesis and Characterization of Model Hydrodesulfurization Catalysts. J. Catal. 1989, 119, 388–399. https://deepblue.lib.umich.edu/bitstream/handle/2027.42/27741/0000133.pdf?sequence=1 doi: 10.1016/0021-9517(89)90169-3
- Seham, N.; Inoue, K. Recovery Of Metal Values From Man-made Recourses; Spent Catalyst And Fly Ash Issued From Municipal Incineration Plants. Doctor Thesis, Dept. of Applied Chemistry, Saga University, Japan, 2000.
- Park, K.H.; Mohapatra, D.; Nam, C.W. Two-stage Leaching of Activated Spent HDS Catalyst and Solvent Extraction of Al Using Organo-phoshinic Extractant, Cyanex-272. J. Hazard. Mater. 2007, 148, 287–295. doi: 10.1016/j.jhazmat.2007.02.034
- Kar, D.J.; Dutta, P.; Misra, V.N. Spent Catalyst: Secondary Source for Mo Recovery. Hydrometallurgy. 2004, 72, 87–92. doi: 10.1016/S0304-386X(03)00122-1
- Medvedev, A.S.; Malochkina, N.V. Sublimation of Molybdenum Trioxide from Exhausted Catalysts Employed for the Purification of Oil Products. Russ. J. Non Ferr. Met. 2007, 48, 114–117. doi: 10.3103/S1067821207020071
- Howard, R.A.; Branes, W.R. Process for Recovering Valuable Metals from Spent Catalysts. US Patent No. 5013533, 1991.
- Parkinson, G.; Isho, S. Recyclers Try New Ways to Process Spent Catalyst. Chem. Eng. 1987, 94, 25–31.
- Gaballah, I.; Diona, M. Valuable Metals Recovery from Spent Catalysts by Selective Chlorination. Resour. Conserv. Recycl. 1994, 10, 87–96. doi: 10.1016/0921-3449(94)90041-8
- Pillai, S.G. The Journey of a Molecule to a Macromolecule;Lambert Academic Publishing: Saarbrücken, 2010.
- Sliwa, W.; Zujewska, T.; Bachowska, Resorcinarenes, B. Polish. J. Chem. 2003, 77, 1079.
- Wright, A.J.; Matthews, S.E.; Fischer, W.B.; Beer, P.D. Novel Resorcin[4]arenes as Potassium Selective Ion Channel and Transporter Mimics. Chem. Eur. J. 2001, 7, 3474. doi: 10.1002/1521-3765(20010817)7:16<3474::AID-CHEM3474>3.0.CO;2-6
- Fox, O.D.; Dalley, N.K.; Harrison, R.G. A Metal-assembled pH-dependent Resorcinarene-based Cage Molecule. J. Am. Chem. Soc. 1998, 120, 7111. doi: 10.1021/ja980150y
- Dasaradhi, L.; Stark, P.C.; Huber, V.J.; Smith, P.H.; Jarvinen, G.D.; Gopalan, A.S. 4-tert Butylcalix[4]arene Tetrahydroxamate Chelators for the Selective Extraction of Actinide Ions: Synthesis and Preliminary Metal Ion Extraction Studies. J. Chem. Soc. Perkin. Trans. 1997, 2, 1187. doi: 10.1039/a607354i
- Pillai, S.G.; Dwivedi, A.H.; Patni, N. Synthesis and Characterization of p-carboxy-N-phenyl-calix[4]resorcinarene, NUiCONE-2011. Proceedings of the 2nd International Conference on Engineering, Chemical Engineering Track; 2011, pp 22–1.
- Zhang, P.; Dong, L.; Zhang, D.; Lei, S.; Hongmei, Y.; Jinying, L.; Wang, Y.; Tarasov, V.V. Efficient Extraction of Nd(III) by Calix[4]arene Derivatives Containing Diethyl Phosphite. Hydrometallurgy. 2017, 169, 47–58. doi: 10.1016/j.hydromet.2016.12.005
- Lu, Y.; Liao, W. Extraction and Separation of Trivalent Rare Earth Metal Ions from Nitrate Medium by p-phosphonic Acid Calix[4]arene. Hydrometallurgy. 2016, 165(Part 2), 300–305. doi: 10.1016/j.hydromet.2016.01.019
- Makrlík, E.; Bureš, M.; Vaňura, P.; Asfari, Z. Extraction and DFT Study on Interaction of the Univalent Thallium Cation with Calix[4]arene-(1,2-phenylene-crown-6,crown-6). J. Mol. Liq. 2016, 218, 473–477. doi: 10.1016/j.molliq.2016.02.030
- Smirnov, I.V.; Stepanova, E.S. Extraction of Americium with Substituted Calix[4]Arenes from Alkaline Solutions. Procedia Chem. 2016, 21, 203–210. doi: 10.1016/j.proche.2016.10.029
- Roberts, B.A.; Cave, G.W.V.; Raston, C.L.; Scott, J.L. Green Chem. 2001, 3, 280. doi: 10.1039/b104430n
- Cindric, M.; Strukan, N.; Vrdoljak, V. Synthesis and Characterization of New Molybdenum(VI) Oxalate Complexes. Crystal Structure of Tetramethylammonium Salt of m-Oxo-diaquadioxalatotetraoxodimolybdenum(VI). Croat. Chem. Acta. 1999, 72(2–3), 501–509. ISSN-0011–1643,CCA-2592, CCACAA.