ABSTRACT
In the present study, Lepidium draba root was used to fabricate silver nanoparticles (Ag NPs). The NPs produced with different amounts of this weed root extract were analyzed by Fourier transform-IR spectroscopy. X-ray diffraction analysis and FE-SEM and TEM techniques were also used to morphologically investigate the particles’ crystal structure. Staphyloccocus aureus, Bacillus cereus, Salmonella typhimurium, and Escherichia coli were then utilized to evaluate the antibacterial activity of the Ag NPs. SEM showed the formation of Ag NPs dispersed within the size range of 20–80 nm. X-ray diffraction analysis showed that the average crystalline size of synthesized Ag NPs was 24 nm. The results indicated that by increasing the concentrations of the root extract of L. draba, one can obtain smaller sized Ag NPs. The antimicrobial activity of those Ag NPs that had undergone green synthesis was more effective than that of corresponding silver ions.
GRAPHICAL ABSTRACT
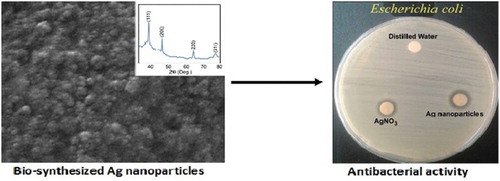
1. Introduction
Owing to the wide application of silver nanoparticles (Ag NPs) in some fields, such as medicine, catalysis, solar energy, and water disinfection, the literature has focused on the synthesis of Ag NPs with minimum hazards and maximum advantage. However, the known chemical, photochemical, electrochemical, radiation, and physical methods are expensive to undertake and associated with risks relating to the natural ecosystem and organisms (Citation1–4). Usually, these methods are toxic and are carried out in several steps. Thus, a process providing benefits such as non-toxicity, fast pace, and eco-friendliness is proposed.
Methods that use biological materials, such as fungi, biomolecules, bacteria, and extracted plants, have been abundantly utilized for Ag NP synthesis (Citation5–8). Among these methods, the use of plant extracts has experienced a rapid advance in the last decade because of advantages such as rapid preparation, eco-friendliness, non-pathogenic effects, non-toxicity, economically feasible procedures, and provision of natural reducing agents of plant extracts for the stabilization of Ag NPs (Citation8–16). Consisting of biomolecules like proteins, amino acids, enzymes, polysaccharides, alkaloids, tannins, phenolics, saponins, terpinoids, and vitamins, plant extracts have been used as reducing and stabilizing agents for converting Ag ions to Ag NPs via a method known as “green synthesis.” The NPs synthesized using the plant system can also be utilized for different commercial applications like pharmaceuticals, drugs, therapeutics, and so on (Citation17). Extracts of different parts of plants such as roots, flowers, seeds, leaves, fruits, latexes, and peels have been used for the biosynthesis of metallic NPs (Citation9, Citation12, Citation15, Citation18). The aqueous extract of Xanthium strumarium (Citation19), Croton bonplandianum (Citation20), and Erigeron bonariensis (Citation21) have been used for the biosynthesis of stable Ag NPs. Ahmed et al. (Citation22) have reviewed the literature released from 2006 to 2015 to demonstrate how extracts of different plants have been applied to fabricate Ag NPs. Since then, more studies have been done on this subject – for example, Allafchian et al. (Citation23) have applied Phlomis extracts to form Ag NPs.
Researchers have explained that metallic-green NPs exhibit antibacterial property (Citation24, Citation25) – for example, Ag NPs with antibacterial properties were synthesized using apple extracts (Citation26). Sedaghat and Afshar (Citation27) have applied Ziziphora tenuior L. water extracts to construct Ag NPs (Citation21). In addition, the green synthesis of Ag NPs using the extract of Clerodendron infortunatum as a reducing and stabilizing agent has been studied (Citation28).
Lepidium draba (L). Link (Brassicaceae; syn. Cardaria draba), commonly known as whitetop or hoary cress, is a perennial herb of the Brassicaceae family that is reproduced by seeds and horizontal creeping roots (Citation29). It is native to Western Asia including Iran, Armenia, Turkey, Israel, Syria, and Iraq Cripps. Chopra et al. (Citation30) have reported that L. draba is antiscorbutic, and the seeds have been used as a cure for flatulence and fish poison. It is an invasive species and has been introduced by contaminated seeds to every continent (Citation31). This wild-growing weed is extensively found in roadsides, meadows, and fields (Citation32). It can be found in most parts of Iran as a weed plant alongside winter crops in orchards and vegetable fields; it can cause a serious problem (Citation33). Thus, it is very important to see we can use this unwanted plant as a useful plant. The aerial parts of L. draba show the presence of alkaloids, terpenoids, tannins, saponins, Leuco anthocyanins, triterpenoids, and flavonoids. Three known flavonoid glycosides, rhamnocitrin-3-O-β d-glucoside, complanatuside, and genkwanin-4′-O-β d-glucoside were isolated from the all parts of L. draba (Citation34).
In a previous study on L. draba, some organic compounds, such as alkaloids, terpenoids, tannins, saponins, Leuco anthocyanin, triterpenoids, and flavonoids, were recognized (Citation35) that were necessary for reducing and stabilizing Ag ions to Ag NPs. The vegetative and reproductive tissues of L. draba also contain two major types of glucosinolates, namely glucoraphanin and glucosinalbin (Citation36). An important type of isothiocyanate (4-[methylsulfinyl] butyl isothiocyanate) can be produced through glucoraphanin hydrolysis via myrosinase activity (Citation37). This isothiocyanate has antioxidant properties (Citation38) and antibacterial effects on Helicobacter pylori (Citation39). Until now, there has been no report on the biosynthesis of Ag NPs from L. draba. In this paper, we used different amounts of the L. draba root extract to prepare Ag NPs. The bio-prepared Ag NPs characteristics and morphological structures were investigated by Fourier transform infrared (FT-IR) spectroscopy, X-ray diffraction analysis, and FE-SEM and TEM techniques. Furthermore, the antibacterial activity of Ag NPs was tested against Staphyloccocus aureus and Bacillus cereus as gram-positive bacteria, while it was tested against Salmonella typhimurium and Escherichia coli as gram-negative bacteria by applying the disk diffusion assay.
2. Materials and methods
2.1. Materials
AgNO3 was obtained from Merck Co. All the glassworks were washed with double-distilled water and then desiccated in the oven at 80°C. Whatman filter paper No. 1 was used for filtration. The L. draba root of a known identity was available in the herbarium of the Department of Natural Resources, Isfahan University of Technology, Iran, and its taxonomic identity was identified.
2.2. Preparation of the extract
The root parts of L. draba were washed and cleaned several times with normal and then distilled water to remove any contamination. As much as 20 g of the root parts of the plant were boiled in 100 mL of distilled water for 10 min. This mixture was filtered through a normal filter and then through Whatman filter paper No. 1. In this way, the L. draba root extract was separated and stored in the refrigerator at 4°C. This extract was applied as a reducing agent for the synthesis of Ag NPs.
2.3. Apparatus
The FT-IR spectra of various amounts of the L. draba root extract in the presence of AgNO3 for Ag NPs formation were acquired by a JASCO FTIR 680 Plus (Japan) on KBr pellets within a range of 3000–400 cm–1. A Philips-X’Pert Pro MPD with Cu kα radiation (λ = 1.54 Å) was applied to record the XRD patterns of Ag NPs within the 2θ range of 10–80° at a step size of 0.02° at 40 kV and 30 mA. In addition, the Ag NPs were morphologically studied by TEM (CM30-Philips) and SEM techniques (HITACHI S-4160).
2.4. Preparing Ag NPs
As much as 120 mL of the aqueous solution of AgNO3 0.01 M was equally spilled into four backer flasks (30 mL in each backer). Consequently, 2.0, 2.5, 3.0, and 3.5 mL of the prepared L. draba root extract was decanted to each breaker, separately at room temperature. The synthesis of NPs using plants can be visually observed because of the change in coloration from light-green to dark-brown (Citation40). After 15 min, the formation of Ag NPs was confirmed by observing the dark-brown color of the mixed solution. In order to disperse the NPs, the ultrasonic homogenizer (Development of Ultrasonic Technology Co., Iran) was used.
2.5. Antibacterial activity assay
The antibacterial activity of the green-synthesized Ag NPs was evaluated following the disk diffusion procedure (Citation13). The antimicrobial effect of Ag NPs was assessed on different pathogenic bacteria such as S. aureus (ATCC 29213), B. cereus (ATCC 14579), E. coli (ATCC35218), and S. typhimurium (ATCC 14028). The inoculum of bacteria was advanced by growing the organism overnight in the Mueller-Hinton medium at 37°C and then sub-cultured in Mueller-Hinton agar overnight. The bacteria colonies were suspended in 2 mL of sterile saline, and the bacterial suspension’s turbidity was adjusted to 0.5 McFarland standards by diluting the sterile saline. The sterile swabs were dipped into the inoculum tubes. The Mueller-Hinton agar plates were inoculated with bacteria by streaking the swabs. The suspension of Ag NPs, AgNO3, and distilled water as negative control were localized into the disks and incubated for 24 h at 37°C. All the disks were used for the antimicrobial activity evaluation against cited bacterial strains on Mueller-Hinton agar plates. The antibacterial activity of Ag NPs was measured by the standard zone of the inhibition assay.
3. Results and discussion
3.1. XRD characterization of bio-prepared Ag NPs
The XRD pattern of the bio-prepared Ag NPs in different amounts of the L. draba root extract is plotted in . The peaks at 2θ values of 38.08°, 44.27°, 64.44°, and 77.62° correspond to the lattice planes of the face-centered cubic crystal structure of Ag NPs at (111), (200), (220), and (311), respectively; these are observed for all amounts of the root extract. These results are in agreement with the Joint Committee on 250 Powder Diffraction Standards file No. 04-0783. By using the Scherrer equation, the average size of bio-prepared Ag NPs was obtained to be 124 nm.
Figure 1. The XRD profile of bio-synthesized Ag NPs at different values of L. draba root extract: (a) 2.0 mL, (b) 2.5 mL, (c) 3.0 mL and (d) 4.0 mL.
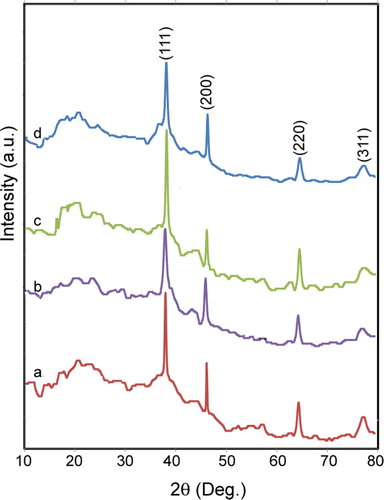
D=Kλ/βcosθ, where K is a constant equal 1, λ is the X-ray source wavelength (1.54 Å), β is the full width half maximum, θ is the corresponding diffraction angle to the lattice plane (111), and finally, D denotes the diameter of Ag NPs.
3.2. FT-IR results
shows the FT-IR spectra of various concentrations of the L. draba root extract in the presence of the AgNO3 solution for Ag NPs fabrication. A phytochemical analysis of the L. draba root extract indicated flavonoids, phenolics, alkaloids, and tannins (Citation35). The L. draba root contains glucoraphanin and glucosinalbin. Glucoraphanin is a group of chemically related compounds known as glucosinolates, which are sulfur- and nitrogen-containing compounds (Citation41).
Figure 2. The FT-IR spectra of different values of L. draba root extract in the presence of Ag solution for Ag NPs making: (a) 2.0 mL, (b) 2.5 mL, (c) 3.0 mL and (d) 4.0 mL.
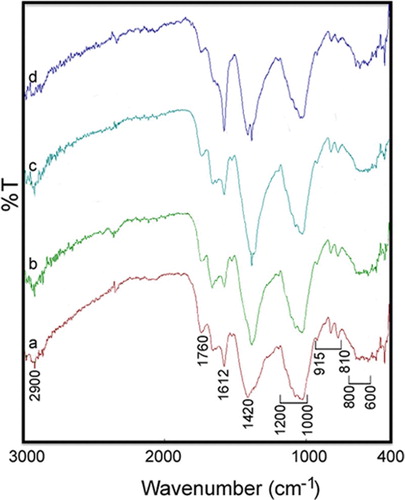
In this figure, peaks are observed at 800–600, 915–810, 1200–1000, 1420, 1612, 1760, and 2900 cm−1. The small band around 2900 cm−1 is attributed to the C−H stretching and bending vibrations. The carbonyl stretch vibration in carboxylic acids, ketones, and aldehydes is observed at around 1760 cm−1 (Citation42–44). The absorption peak at about 1612 cm−1 can be attributed to the asymmetric stretching vibration of COO−, while the band at 1420 cm−1 is a result of the symmetric stretching vibration of COO− and the stretching vibration of C−O (Citation44–46). Moreover, the band within 1200–1000 cm−1 is dominated by ring vibrations overlapped with stretching vibrations of (C−O−H) side groups and the (C−O−C) glycoside band vibration. Further, the band within 810–915 cm−1 is attributed to the presence of polysaccharide. The bands within the range of 800–600 cm−1 are attributed to the C–H out-of-the-plane bend of aromatic phenols. These results show that aldehydes, glycoside, phenolic, and carboxylic acids of the L. draba root extract are mainly involved in the construction of Ag NPs. The presence of protective and reductive biomolecules in the plants can be a major factor for the synthesis of NPs (Citation40).
3.3. The FE-SEM and TEM analysis results
The morphology and crystalline structures of bio-prepared Ag NPs were studied via FE-SEM and TEM methods. In , the spherical NPs were evidently recognized and dispersed within the size range of 20–80 nm. It is interesting that with increases in the amount of the root extract, the SEM and TEM images of Ag NPs vary. Therefore, it is significant that the shapes and sizes of Ag NPs depend on the plant extract concentration, which changes the optical and electronic property of NPs (Citation47).
Figure 3. The FE-SEM patterns of bio-synthesized Ag NPs at different values of L. draba root extract: (a) 2.0 mL, (b) 2.5 mL, (c) 3.0 mL and (d) 4.0 mL. The inserted figures are related to TEM images.
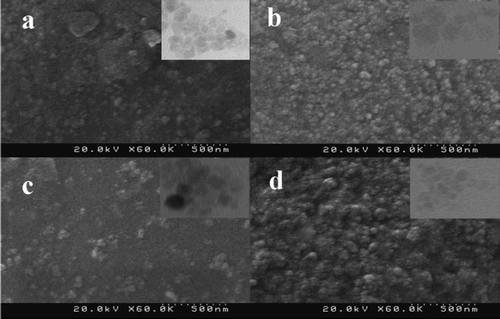
Continuing with this research, the sizes of Ag NPs were measured by Digimizer Software where the corresponding histogram was plotted (). Applying 2.0, 2.5, 3.0, and 4.0 mL of the plant root extract as a reducing agent, the average particle sizes for Ag NPs were obtained to be 42.5, 37.7, 34.4, and 32.5 nm, respectively. The results indicated that the amount of the plant extract is related inversely to the sizes of Ag NPs so that an increase in the amount of the plant extract decreases the sizes of Ag NPs. The metal-reductive process was invigorated in the presence of the larger amounts of biomolecules in such a way that Ag NPs of smaller sizes were obtained.
Figure 4. The histogram of bio-synthesized Ag NPs size by using of different values of L. draba root extract.
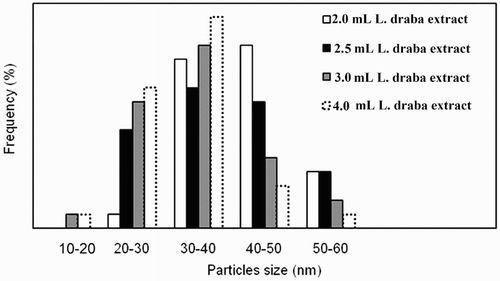
The synthesis of NPs using plants is relatively fast compared with the other systems and can be visually observed because of the change in coloration from light-green to dark-brown. The shapes and sizes of the NPs can be modulated by a slight change in the pH and temperature of the NPs (Citation48).
3.4. Antibacterial activity results
In , the disc diffusion results of Ag NPs, the AgNO3 solution, and the distilled water (as negative control) are shown, with the corresponding zone of inhibition presented in against gram-positive (S. aureus and B. cereus) and gram-negative (S. typhimurium and E. coli) bacteria. Regarding these results, it can be concluded that the bio-synthesized Ag NPs by the L. draba root extract (4 mL) have higher antimicrobial activity than AgNO3, as measured against all the cited bacteria. This may be because of the bacterial cell death due to accumulation of metallic NPs inside the cell membrane and the release of cellular compounds (Citation24). Since smaller sized NPs had a larger surface area for interaction with bacteria, their antibacterial activity was higher than that of larger sized NPs (Citation49).
Figure 5. The antibacterial activity of Ag NPs against microorganisms: (1) Ag NPs, (2) distilled water and (3) AgNO3.
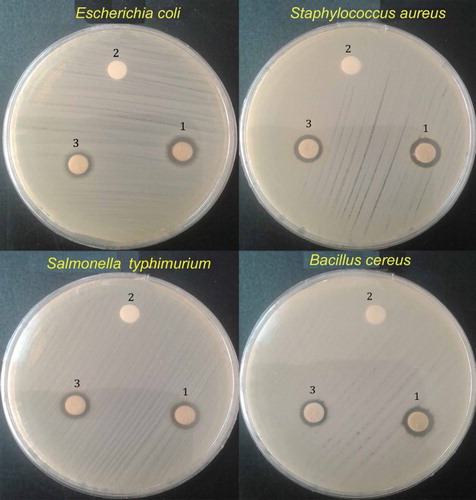
Table 1. The zone of inhibition (mm) of Ag NPs, AgNO3 and distilled water against tested bacteria.
4. Conclusion
This study demonstrated a green synthesis procedure for Ag NPs by applying the L. draba root extract as a reducing agent. By using XRD, FT-IR, FE-SEM, and TEM techniques, the fabrication, structure, and morphology of Ag NPs were investigated. Corresponding FT-IR to the functional groups of carbonyl (–C9O), glyosidic (C–O–C), and carboxylic acid (–COO) showed that aldehydes, carboxylic acids, glycosidic, and phenolics-components of the L. draba root extract play an important role in Ag NPs fabrication. In the XRD pattern of Ag NPs, four characteristic peaks were detected and their spherical shape was obviously observed in SEM and TEM images. The size distribution with different amounts of the L. draba root extract showed that the smallest Ag NPs were achieved by applying the highest amount of the plant extract. The zone inhibition results showed that in comparison with Ag ions, the Ag NPs have a larger potential in terms of antimicrobial activity. The demonstrated fast synthesizing method and superior antimicrobial activity of the bio-synthesized Ag NPs may suggest the use of plant extracts for the preparation of Ag NPs. This outcome could be used as an application of the unwanted plants, especially in crop fields, to produce eco-friendly NPs.
Disclosure statement
No potential conflict of interest was reported by the authors.
Notes on contributors
Fatemeh Benakashani was born in Tehran, Iran, in 1979. He received his Ph.D. degree from University of Tehran (UT) in 2011. She is an assistant professor of Weed Science (Since April 2016), University of Tehran, Tehran, Iran. Assistant professor of Weed Science, Institute of Isfahan University of Technology, Isfahan, Iran. (2012–2016). Weed Researcher at Iranian Research Institute of Plant Protection (IRIPP), Tehran, Iran. (2005–2012). She is working on nonchemical management of weeds and practical usage of unexpected plants as a safe material to biosynthesis of nanoparticles. She also researched on herbicides nano-capsulation. Dr. Benakashani has more than 4 items awards. Her research project was selected at the 21th Kharazmi Festival and she was successful in obtaining a scholarship from the National Elite Foundation.
Alireza Allafchian was born in Isfahan, Iran, in 1983. He received his Ph.D. degree from Isfahan University of Technology (IUT) in 2012. His Ph.D. thesis was devoted to the preparation of electrochemical sensors and coupling of this method with the extraction method based on nanoparticles. Later on, he joined the Research Institute for Nanotechnology and Advanced Materials, Isfahan University of Technology (IUT), Iran, serving as an assistance professor since 2012. He opened new fields of researches in this Institute, such as preparation and characterization of nanocomposites and nano particles for advanced applications in the field of sensor and novel antibacterial materials. Dr. Allafchian has published more than 44 ISI journal papers, more than 72 national and international conference papers and 11 Iranian patents. He has more than 8 items awards. The most important ones were foundation award and outstanding student award in the country and IUT.
Seyed Amir Hossein Jalali is assistant professor at Isfahan University of Technology (IUT). He was born in Isfahan, Iran, in 1980. He earned DVM at Shahrekord University, Faculty of Veterinary Medicine, in 2005. His Ph.D. degree in Biotechnology from the University of Tehran, in 2011. In his thesis work he produced antibodies specific to bovine gamma interferon by genetic immunization. After completing Ph.D. he joined the Research Institute for Biotechnology and Bioengineering, Isfahan University of Technology (IUT), Iran, since 2012. In 2016 he accepted a position in Department of Natural Resources. During that time he focused and developed nanomaterial applications in the field of biotechnology. Dr. Jalali has published more than 16 ISI journal papers, more than 35 national and international conference papers.
ORCID
Fatemeh Benakashani http://orcid.org/0000-0002-9123-5168
Alireza Allafchian http://orcid.org/0000-0002-6728-6221
References
- Dimitrijevic, N.M.; Bartels, D.M.; Jonah, C.D.; Takahashi, K.; Rajh, T. J. Phy. Chem. B. 2001, 105 (5), 954–959. doi: 10.1021/jp0028296
- Sun, Y.; Yin, Y.; Mayers, B.T.; Herricks, T.; Xia, Y. Chem. Mat. 2002, 14 (11), 4736–4745. doi: 10.1021/cm020587b
- Callegari, A.; Tonti, D.; Chergui, M. Nano Lett. 2003, 3 (11), 1565–1568. doi: 10.1021/nl034757a
- Yin, B.; Ma, H.; Wang, S.; Chen, S. J. Phy. Chem. B. 2003, 107 (34), 8898–8904. doi: 10.1021/jp0349031
- Moghaddam, A.B.; Namvar, F.; Moniri, M.; Tahir, P.M.; Azizi, S.; Mohamad, R. Molecules. 2015, 20 (9), 16540–16565. doi: 10.3390/molecules200916540
- Venkatpurwar, V.; Pokharkar, V. Mat. Lett. 2011, 65 (6), 999–1002. doi: 10.1016/j.matlet.2010.12.057
- Shivaji, S.; Madhu, S.; Singh, S. Proc. Biochem. 2011, 46, 1800–1807. doi: 10.1016/j.procbio.2011.06.008
- Kaviya, S.; Santhanalakshmi, J.; Viswanathan, B.; Muthumary, J.; Srinivasan, K. Spectrochimica Acta Part A: Mol Biomol Spect. 2011, 79, 594–598. doi: 10.1016/j.saa.2011.03.040
- Harekrishna, B.; Dipak, K.B.; Gobinda, P.S.; Priyanka, S.; Santanu, P.M.Ajay, M. Colloids Surf. A Physicochem.Eng Asp. 2009, 348, 212–216. doi: 10.1016/j.colsurfa.2009.07.021
- Jha, A.K.; Prasad, K. Digest J. Nanomat. Biostruc. 2011, 6 (4), 1717–1723.
- Arokiyaraj, S.; Saravanan, M.; Vijayakumar, B. South Indian J. Biol. Sci. 2015, 1 (2), 115–118. doi: 10.22205/sijbs/2015/v1/i2/100433
- Ibrahim, H.M.M. J. Radiation Res. App. Sci. 2015, 8 (3), 265–275. doi: 10.1016/j.jrras.2015.01.007
- Kathiravan, V.; Ravi, S.; Ashokkumar, S.; Velmurugan, S.; Elumalai, K.; Chandra, P.K. Spectrochim Acta A Mol. Biomol. Spectrosc. 2015, 139, 200–205. doi: 10.1016/j.saa.2014.12.022
- Mohammed, A.E. Asian Pacific J. Trop. Biomed. 2015, 5 (5), 382–386. doi: 10.1016/S2221-1691(15)30373-7
- Padalia, H.; Moteriya, P.; Chanda, S. Arabian J. Chem. 2015, 8 (5), 732–741. doi: 10.1016/j.arabjc.2014.11.015
- Ponvel, K.M.; Narayanaraja, T.; Prabakaran, J. Int. J. Nano Dimension. 2015, 6 (4), 339–349.
- Mahendra, R.; Yadav, Alka. IET Nanobiotechnol, Special Issue: Recent Advances in Biosynthesis of Nanoparticles and their Applications. 2013, 7 (3), 117–124. doi: 10.1049/iet-nbt.2012.0031
- Guidelli, E.J.; Ramos, A.P.; Zaniquelli, M.E.D.; Baffa, O. Spectrochimica Acta Part A: Mol. Biomol. Spectroscopy. 2011, 82 (1), 140–145. doi: 10.1016/j.saa.2011.07.024
- Kumar, V.; Kumar Gundampati, R.; Kumar Singh, D.; Jagannadham, M.V.; Sundar, S.h.; Hasan, S.H. J. Ind. Eng. Chem. 2016, 37, 224–236. doi: 10.1016/j.jiec.2016.03.032
- Kumar, V.; Mohan, S.; Singh, D.K.; Verma, D.K.; Kumar Singh, Vika; Hasan, S.H. Mat. Sci. Eng. 2017, 71, 1004–1019. doi: 10.1016/j.msec.2016.11.013
- Kumar, V.; Kumar Singh, D.; Mohan, S.; Hasan, S.H. J. Photochem. Photobiol. B: Biol. 2016,39–50. doi: 10.1016/j.jphotobiol.2015.12.011
- Ahmed, S.; Ahmad, M.; Swami, B.L.; Ikram, S. J. Adv. Res. 2016, 7 (1), 17–28. doi: 10.1016/j.jare.2015.02.007
- Allafchian, A.; Mirahmadi-Zare, S.Z.; Jalali, S.A.H.; Hashemi, S.S.; Vahabi, M.R. J. Nanostruc. Chem. 2016,1–7.
- Groiss, S.; Selvaraj, R.; Varadavenkatesan, T.h.; Vinayagam, R. J. Mol. Struct. 2017, 1128, 572–578. doi: 10.1016/j.molstruc.2016.09.031
- Selvaraj, R.; Vinayagam, R.; Varadavenkatesan, T.h. Arabian J.Chem. 2017, 10, 253–261. doi: 10.1016/j.arabjc.2015.06.023
- Ali, Z.A.; Yahya, R.; Sekaran, S.D.; Puteh, R. Adv. Mat. Sci. Eng. 2016,1–6.
- Sedaghat, S.; Afshar, P. J. Appl. Chem. Res. 2016, 10 (1), 103–109.
- Jha, A.K.; Prasad, K. Adv. Mater. Lett. 2016, 7 (1), 42–46. doi: 10.5185/amlett.2016.6083
- Dixon, G.R. Vegetable brassicas and related crucifers, CABI2007, 327 pp.
- Chopra, R.N.; Nayar, S.L.; Chopra, I.C. Glossary of Indian Medicinal Plants (Including the Supplement); Council of Scientific and Industrial Research: New Delhi, 1986.
- Radonić, A.; Blažević, I.; Mastelić, J.; Zekić, M.; Skočibušić, M.; Maravić, A. Chem. Biodivers. 2011, 8(6): 1170–1181. doi: 10.1002/cbdv.201000370
- Graves-Medley, M.; Jane, M. Biology, Ecology and Management of Whitetop (Cardaria spp.);Montana State University Extension: Bozeman, 2011.
- Rezvani, M.; Zaefarian, F. Plant Species Biol. 2015, 31 (4), 280–287. doi: 10.1111/1442-1984.12113
- Bichaa, S.; Benmekhebib, L.; Boubekria, N.; Khellafc, R.; Brouardd, I.; Zamaa, D.; Benayachea, Samir.; Benayachea, F. RJPBCS. 2016, 7 (2), 283–287.
- Mojab, F.; Mohammad, K.; Naysaneh, G.; Reza, V.H. Iran. J. Pharm. Res. 2003,77–82.
- Powell, E.E.; Hill, G.A.; Juurlink, B.H.J.; Carrier, D.J. J. Chem. Technol. Biotechnol. 2005, 80 (9), 985–991. doi: 10.1002/jctb.1273
- Fahey, J.W.; Zalcmann, A.T.; Talalay, P. Phytochemistry. 2001, 56 (1), 5–51. doi: 10.1016/S0031-9422(00)00316-2
- Fahey, J.W.; Talalay, P. Food Chem. Toxicol. 1999, 37 (9), 973–979. doi: 10.1016/S0278-6915(99)00082-4
- Fahey, J.W.; Haristoy, X.; Dolan, P.M.; Kensler, T.W.; Scholtus, I.; Stephenson, K.K.; Talalay, P.; Lozniewski, A. Proc. Nat. Acad. Sci. 2002, 99 (11), 7610–7615. doi: 10.1073/pnas.112203099
- Thakkar, K.N.; Mhatre, S.S.; Parikh, R.Y. Nanomedicine. 2011, 6 (2), 257–262. doi: 10.1016/j.nano.2009.07.002
- Miri, A.; Sharifi-Rad . J.; Sharifi-Rad . M.; Teixeira da Silva, J.A. Ann. Biol. Res. 2013, 4 (6), 76–79.
- Mohan, J. Alpha science international;CRC Press Reference: Harrow, 2004, 563.
- Philip, D. Spectrochimica Acta. Part A. 2010, 77, 807–810. doi: 10.1016/j.saa.2010.08.008
- Zhao, S.-Y.; Chen, S.-H.; Li, D.-G.; Yang, X.-G.; Ma, H.-Y.; Zhao, S.-Y.; Chen, S.-H. Physica E: Low-dimensional Syst. Nanostruct. 2004, 23, 92–96. doi: 10.1016/j.physe.2004.01.008
- Zhao, T.; Mao, G.; Feng, W.; Mao, R.; Gu, X.; Li, T.; Li, Q.; Bao, Y.; Yang, L.; Wu, X. Carbohydr. Polym. 2014, 105, 26–33. doi: 10.1016/j.carbpol.2014.01.059
- Jiang, Y.-y.; Wang, L.; Zhang, L.; Wang, T.; Yu, L.; Ding, C.-b.; Yang, R.-w.; Wang, X.-l.; Zhou, Y.-h. Int. J. Biol. Macromol. 2014, 70, 92–99. doi: 10.1016/j.ijbiomac.2014.06.036
- Xu, H.; Käll, M. J Nanosci Nanotechnol. 2002, 4, 254–259.
- Rai, M.; Yadav, Alka. IET Nanobiotechnol. 2013, 7 (3), 117–124. doi: 10.1049/iet-nbt.2012.0031
- Paná cek, A.; Kvítek, L.; Prucek, R.; Kolá r, M.; Vecerová, R.; Pizúrová, N.; Sharma, V.K.; Neve cná, T.j.; Zboril, R. J. Phys.Chem. B. 2006, 110, 16248–16253. doi: 10.1021/jp063826h