ABSTRACT
A green and efficient protocol for the synthesis of densely functionalized 2-aminothiophene derivatives is described by the one-pot three-component Gewald reaction from enolizable carbonyl compounds, malononitrile (or ethyl cyanoacetate) and elemental sulfur in polyethylene glycol 600, as an eco-friendly reaction medium, without using any basic compounds under ultrasonic be recovered and reused at least five times without significant loss of its activity. The merits of this protocol are mild reaction conditions, good yields, short reaction time, simple work-up procedure and recyclability of the reaction medium as well as avoiding the use of any basic catalyst at ambient temperature.
GRAPHICAL ABSTRACT
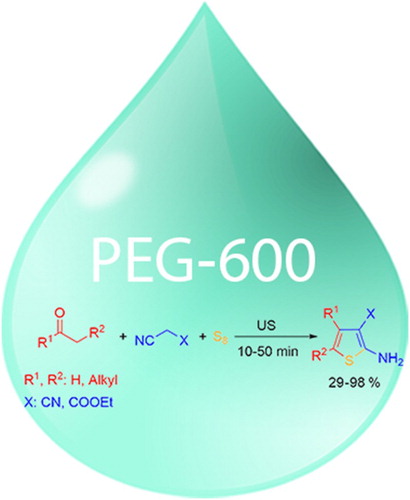
Introduction
Chemical pollution is one of the greatest threats to living organisms including plants, animals and humans. In recent years, the environmental pollution has been increased as one of the consequences of the development of the chemical industry. Therefore, improvement of new chemical processes using more environmentally benign materials and atom-efficient procedures is in high demand. Indeed, the toxic and volatile nature of many organic solvents has posed a serious threat to the environment because such solvents significantly contribute to the chemical wastes. Therefore, avoiding or minimizing the use of volatile organic compounds (VOCs) in chemical reactions is an effective way to reduce the release of toxic compounds to the environment. Hence, development of protocols working under solvent-free conditions or using green and recyclable solvents can be considered as the proper alternatives of VOCs (Citation1–5). In this way, polyethylene glycols (PEGs) have attracted increasing interest in the context of green synthesis during recent years (Citation6, Citation7). Among considerable features of PEGs, thermal stability, non-volatility, non-toxicity, recyclability and commercial availability are more notable (Citation8). Additionally, PEGs can also play the role of phase-transfer catalysts because of their unique ability for cationic coordination (Citation6, Citation9, Citation10). Consequently, PEGs have been used as green solvents in many organic reactions including substitution, oxidation, reduction, Michael addition and even one-pot multicomponent reactions (MCRs) (Citation10, Citation11).
Nowadays, MCRs have been considered as an efficient synthetic strategy among academic and industrial research groups because these reactions afford highly functionalized molecules such as biologically active compounds especially heterocyclic scaffolds in convenient and one-pot procedures. Lower reaction and purification steps, and high atom economy as well as highly convergent and requiring minimum time compared to multi-step alternative synthetic routs make MCRs good candidates for green organic processes (Citation12–19). The Gewald reaction is a popular catalytic and one-pot three-component reaction between elemental sulfur, a cyanomethylene containing an electron-withdrawing group and an enolizable carbonyl compound that affords highly substituted 2-aminothiophene derivatives (Citation12, Citation20). Indeed, 2-aminothiophene scaffold has found wide applications from modern drug design (Citation21, Citation22) to dye chemistry (Citation23, Citation24) ().
shows some typical biologically active compounds containing 2-aminothiophene scaffold which can be prepared through the Gewald MCR. For instance, compounds A and B enhance the activity of agonists acting at the A1 adenosine receptor (A1AR) (Citation25), and compound C inhibits the binding of L-glucagon to the human glucagon receptor (Citation26). The Gewald reaction is traditionally proceeded in the presence of the excess amount of secondary or tertiary amines such as diethylamine or morpholine (Citation27). Recently, some modifications to the Gewald reaction have been reported in the literature. For example, some basic compounds such as imidazole (Citation28), Mg/La mixed oxide (Citation29), silica-supported piperazine (Citation30), bovine serum albumin (Citation31), ZnO nano-particles (Citation32), saturated NaHCO3 solution in PEG 600 (Citation13) and ionic liquids can be mentioned (Citation33). Furthermore, techniques such as solid phase synthesis (Citation34), microwave irradiation combined with insoluble polymer support (Citation35) or soluble polymer support (Citation36), and electrochemical activation (Citation37) have also been reported. In spite of their merits, most of these methods require the use of basic compounds, high catalyst loading, long reaction times and expensive catalysts or toxic solvents. Therefore, development of eco-friendly protocols using more efficient and safer conditions for the Gewald MCRs is still in high demand. Furthermore, the application of ultrasound irradiation in synthetic organic chemistry has received considerable attention recently (Citation38, Citation39).
Sonochemistry was initially introduced in 1970s and defined as the use of ultrasound waves in various branches of chemistry. The range of ultrasound frequency is between ∼ 20 kHz and 100 MHz which is beyond the frequency that can be detected by the human ear. Ultrasound spectrum can be subdivided into three main regions: Low frequency, high-power ultrasound (20–100 kHz); intermediate frequency, medium power ultrasound (100 kHz–1 MHz); and high frequency, low-power ultrasound (1–10 MHz). It is noteworthy that the range from 20 kHz to ∼ 1 MHz is generally used in sonochemistry (Citation40). Ultrasonic-assisted organic synthesis, as a green synthetic approach, is a powerful technique which is being used to accelerate a wide range of organic reactions. In general, sonication increases the reaction rates and yields without using harsh conditions (Citation41, Citation42). In fact, ultrasound waves through acoustic cavitation phenomenon generate energetic bubbles in the solvent media (Citation43, Citation15). These bubbles possess very high interior pressure and temperature which prepare an intensive condition to promote the organic reactions more effectively (Citation44). In order to describe the regions where sonochemical reactions occur, a three-site model was introduced. According to this model, the reaction can occur either in the bubble’s hot interior gas phase where the temperature is about 5000 K, in the liquid shell surrounding the bubble which can be as high as 1900K, or in the liquid-phase from the injection of liquid droplets into the hot zone during the asymmetric collapse of the bubble as well as the bulk solvent (Citation39).
In this work, a green and highly efficient method for the one-pot synthesis of 2-aminothiophene derivatives is described by the Gewald three-component reaction between alkylketones, activated C–H acids and elemental sulfur in PEG 600 medium under ultrasonic irradiation without using any basic catalyst or toxic solvent at ambient temperature (Scheme 1).
Experimental
General
All commercially available chemicals were obtained from Merck and Sigma-Aldrich, and used without further purifications. The reactions were carried out by Topsonic ultrasonic liquid processors (Iran, Tehran) with the frequency of 20 kHz and power of 360 W. Thin-layer chromatography (TLC) for monitoring of reactions was performed using Merck 0.2 mm silica gel 60 F-254 Al-plates, and EtOAc and n-hexane (1:1, v/v) as eluent. Melting points, which are uncorrected, were determined using an Electrothermal 9100 apparatus. 1H NMR (500 MHz) spectra were recorded on a Bruker DRX-500 Avance spectrometer in CDCl3 at ambient temperature. All chemical shifts are given relative to TMS. Fourier Transfer Infrared (FT-IR) spectra were acquired on a Shimadzu FT-IR-8400S spectrometer. All yields refer to the isolated products.
General procedure for the synthesis of 2-aminothiophenes in PEG 600 under ultrasonication
A mixture of carbonyl compound (1.0 mmol), malononitrile or ethyl cyanoacetate (1.0 mmol) and elemental sulfur (1.0 mmol) in PEG 600 (2 mL) was sonicated by an ultrasonic probe at 20 kHz and power of 360 W for 10–50 min at ambient temperature. The reaction was monitored by TLC in n-hexane/EtOAc (1:1, v/v). After completion of the reaction, crushed ice was added to the reaction mixture and it was filtered off. The filtrate was kept for recycling of PEG 600. The precipitate was washed with distilled water (2 mL) and then recrystallized from EtOH. The separated PEG 600 can be recovered by direct evaporation of excess water under vacuum conditions or extraction by a suitable solvent such as Et2O or EtOAc (2 × 5 mL). For the products with lower yields than 80%, the crude residues were dissolved in EtOAc (15 mL) and PEG 600 was then separated by water (2 × 2 mL). For better separation of the two organic and aqueous phases, NaCl may be used. The organic phase was purified by flash column chromatography on silica gel using n-hexane/EtOAc (1:3, v/v).
Selected spectral data
2-Amino-4,5,6,7-tetrahydrobenzo[b]thiophene-3-carbonitrile (4b)
Light brown solid; M.p.: 139–141°C (reported M.p. (Citation31) 140–142°C); FT-IR (KBr, cm–1): 3444, 3326, 2194; 1H NMR (500 MHz, CDCl3): δ 4.6 (2H, bs, NH2), 2.5–2.4 (4H, m, CH2), 1.8 (4H, m, CH2); 13C NMR (125 MHz, CDCl3): δ 160.1, 131.5, 121.1, 115.3, 87.8, 24.6, 23.9, 23.2, 22.0.
2-Amino-4-methylthiophene-3-carbonitrile (4e)
Light brown solid; M.p.: 118–120°C (reported M.p. 117–119°C (Citation31)); FT-IR (KBr, cm–1): 3415, 3322, 2200; 1H NMR (500 MHz, CDCl3): δ 6.1 (1H, s, CH), 4.7 (2H, bs, NH2), 2.2 (3H, s, CH3); 13C NMR (125 MHz, CDCl3): δ 161.9, 135.4, 115.0, 105.7, 91.2, 15.9.
2-Amino-4-phenylthiophene-3-carbonitrile (4h)
Pale Yellow solid; M.p.: 132–136°C (reported M.p. 136–137°C (Citation32)); FT-IR (KBr, cm–1): 3416, 3316, 3198, 2217, 1627, 1565, 1425; 1H NMR (500 MHz, CDCl3): δ 7.6 (2H, m, CH), 7.3 (2H, m, CH), 7.2 (1H, m, CH), 6.2 (1H, s, CH), 5.0 (2H, bs, NH2); 13C NMR (125 MHz, CDCl3): δ 153.8, 139.3, 136.6, 129.7, 129.1, 128.0, 115.2, 80.1.
Ethyl 2-amino-4,5,6,7-tetrahydrobenzo[b]thiophene-3-carboxylate (4k)
Yellow solid; M.p.: 113–114°C (reported M.p. 114–115°C (Citation31)); FT-IR (KBr, cm–1): 3401, 3296, 1645; 1H NMR (500 MHz, CDCl3): δ 5.9 (2H, bs, NH2), 4.3 (2H, q, J = 7.2 Hz, CH2), 2.7–2.6 (2H, m, CH2), 2.5–2.4 (2H, m, CH2), 1.8–1.7 (4H, m, CH2), 1.3 (3H, t, J = 7.2 Hz, CH3); 13C NMR (125 MHz, CDCl3): δ 167.2, 162.1, 132.7, 118.0, 106.4, 60.6, 27.5, 25.2, 23.9, 23.3, 15.1.
Ethyl 2-amino-4-methylthiophene-3-carboxylate (4n)
Yellow solid; M.p.: 77–79°C (reported M.p. 78°C (Citation31)); FT-IR (KBr, cm–1): 3411, 3300, 1648; 1H NMR (500 MHz, CDCl3): δ 6 (2H, bs, NH2), 5.7 (1H, s, CH), 4.2 (2H, q, J = 7.1 Hz, CH2), 2.3 (3H, s, CH3), 1.3 (3H, t, J = 7.1 Hz, CH3); 13C NMR (125 MHz, CDCl3): δ 172.6, 160.9, 127.1, 114.5, 104.3, 60.6, 20.2, 12.8.
Ethyl 2-amino-4-phenylthiophene-3-carboxylate (4q)
Yellow solid; M.p.: 94–96°C (reported M.p. 93°C (Citation29)); FT-IR (KBr, cm–1): 3443, 3341, 1674; 1H NMR (500 MHz, CDCl3): δ 7.5–7.4 (5H, m, CH), 6.8 (2H, bs, NH2), 6.3 (1H, s, CH), 4.3 (2H, q, J = 7.1 Hz, CH2), 1.3 (3H, t, J = 7.1 Hz, CH3); 13C NMR (125 MHz, CDCl3): δ 164.7, 161.2, 143.9, 135.8, 129.8, 129.0, 128.3, 108.4, 105.4, 60.1, 14.6.
Results and discussion
At first, a mixture of cyclohexanone (1a, 1 mmol), malononitrile (2a, 1 mmol) and elemental sulfur (3, 1 mmol), as the model reaction, was heated in PEG 400 (2 mL) at 90°C in the absence of any catalyst. The progress of the reaction was monitored by TLC. To our delight, the desired product of 2-amino-4,5,6,7-tetrahydrobenzo[b]thiophene-3-carbonitrile (4a) was obtained in 85% yield after 40 min (, entry 1). In the next experiments, a systematic study was performed to investigate the efficiency of various polyethers under different conditions. Therefore, the model reaction was examined in PEG 600 and polypropylene glycol 1000 (PPG 1000) to compare the performance of other polyethers as the reaction medium (entries 2 and 3). As it can be deduced from data in , PEG 600 afforded a quantitative yield of the desired product 4a in a short reaction time compared to PEG 400. On the other hand, only the Knoevenagel condensation product of 1a and 2a was obtained in poor yield when PPG 1000 was used as the reaction medium. Hence, PEG 600 was selected as the most efficient reaction medium among the others. In the next optimization experiments, the model reaction was investigated in different volumes of PEG 600 (entries 4–6). According to the obtained results, 2 mL of PEG 600 per 1 mmol of reactants was chosen as an appropriate volume. To obtain the optimum temperature, the model reaction was also studied at different temperatures (entries 7–10). The obtained results showed that 90°C is the optimum temperature under thermal conditions. Moreover, the model reaction was examined under ultrasonic conditions at ambient temperature. Under ultrasonic conditions, the desired product 4a was also obtained in quantitative yield and shorter time when PEG 600 was used as the reaction medium instead of PEG 400 and PPG 1000 (entries 11–13). It can be concluded that during sonication of the reaction mixture in PEG 600, cavitation phenomenon through formation, growth and collapse of microbubbles in the solvent provides a high pressure and temperature conditions to promote the reaction. Indeed, the temperature of the reaction mixture was increased about 90°C during sonication. On the other hand, vigorous agitation of the reaction mixture provides a maximum dispersion of insoluble sulfur in the reaction medium which makes elemental sulfur more accessible to react with the Knoevenagel intermediate and thereby increases the rate of the reaction.
Table 1. Optimization of the Gewald three-component reaction under thermal and ultrasound conditionsa.
In the next step, 2 mL of PEG 600 under ultrasonic conditions at ambient temperature, as the optimized reaction conditions, was developed to other derivatives of various enolizable carbonyl compounds (1a–h) and activated nitriles (2a–b). The results have been summarized in . Both cyclohexanone and cycloheptanone afforded higher yields compared to other cycloalkanones under optimized conditions (entries 2, 3, 10, 11). Indeed, cycloalkanones having larger rings than cycloheptanone afforded slightly lower yields of their corresponding products (entries 4, 12), perhaps due to their lower reactivity. Furthermore, when cyclopentanone was used, the corresponding products were obtained in lower yields (entries 1, 9). On the other hand, linear carbonyl compounds were also involved in the optimized reaction conditions to afford corresponding 2-aminothiophene derivatives in good to moderate yields (entries 5–7, 13–15). Moreover, the low yields of acetophenone can be rationalized by the carbonyl group conjugation with the phenyl ring that reduces the reactivity of carbonyl group against nucleophilic attack by the carbanion of the activated nitriles (entries 8 and 16). Also, the ease of carbanion formation can affect the yield of the desired product. Accordingly, when ethyl cyanoacetate (2b) was used instead of malononitrile (2a), the corresponding products were obtained in lower yields.
Table 2. Gewald reaction of various carbonyl compounds under the optimized conditionsa.
A proposed mechanism for the synthesis of 2-aminothiophene derivatives in PEG 600 medium has been shown in Scheme 2. Indeed, the high ability associated with the proper geometry of oxygen atoms in the PEG 600 chain is responsible for such reactivity to promote the Gewald reaction in the absence of any basic compound. Therefore, it can be proposed that chelation ability of PEG 600 is enough to dissociate alpha hydrogens of activated nitriles and later intermediates including the Knoevenagel one and promote the reaction in the next steps to afford desired products 4.
Scheme 2. Proposed mechanism for the three-component Gewald reaction in PEG 600 medium under ultrasonication.
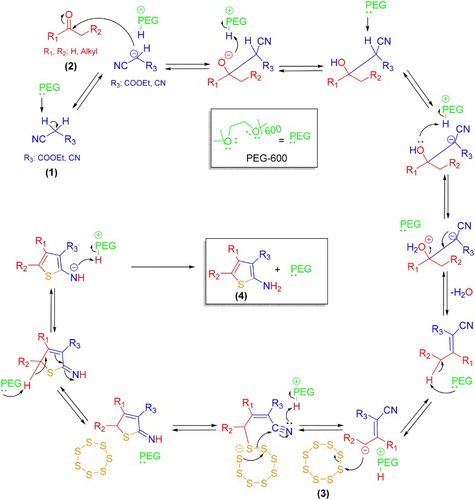
Another important aspect of PEG 600, as a green and efficient reaction medium, is its high potential for recyclability. It has been shown that the PEG 600 could be recovered and reused several times in the subsequent runs without a considerable loss of its efficiency ().
Figure 2. Reusability of PEG 600 in the synthesis of 2-amino-4,5,6,7-tetrahydrobenzo[b]thiophene-3-carbonitrile (4b).
![Figure 2. Reusability of PEG 600 in the synthesis of 2-amino-4,5,6,7-tetrahydrobenzo[b]thiophene-3-carbonitrile (4b).](/cms/asset/5afad1ec-2c5a-41a6-bb93-e22da0ee4325/tgcl_a_1380234_f0002_c.jpg)
In order to clarify the efficiency of suggested method in the synthesis of 2-aminothiophene derivatives, the comparison of previously reported procedures with the present method has been summarized in . As results demonstrate, the present protocol is indeed superior to several of the others in terms of product yield, reaction time, elimination of catalyst and source of energy.
Table 3. Comparative synthesis of compound 4b using the reported methods versus the present method.
Conclusions
In summary, the combination of PEG 600, as an eco-friendly and efficient reaction medium, with ultrasonication, as a green and effective source of energy, provides a high-performance method for the synthesis of densely functionalized 2-aminothiophene derivatives. This new protocol offers several advantages such as short reaction time, avoiding the use of any basic compound, recyclability of the reaction medium and simple work-up procedure which makes this method an instrumental alternative to the previous methodologies for the scale-up of this one-pot three-component reaction.
Disclosure statement
No potential conflict of interest was reported by the authors.
Notes on contributors
Ali Akbarzadeh was born in Tehran, Iran, 1991. He studied Applied Chemistry at Khajeh Nasir Toosi University of Technology (KNTU), Tehran, Iran and received his B.Sc in July, 2013. In October 2013, he started his studies at M.Sc level of organic Chemistry under supervision of Dr. Mohammad G. Dekamin in Iran University of Science and Technology (IUST), Tehran, Iran and was graduated in October, 2015. In the same year, he was joined to the research group of Dr. Reza Zadmard, as a Ph.D candidate, at Chemistry and Chemical Engineering Research Center of Iran (CCERI), Tehran, Iran. His research interests are green chemistry, heterogeneous catalysis and supramolecular chemistry.
Mohammad G. Dekamin was born in Nahavand, Iran, 1972. He studied Chemistry at Shahid Chamran University (SCU), Ahvaz, Iran and received his B.Sc Degree in July, 1995. Dr. Dekamin obtained his M.Sc Degree in Organic Chemistry under the supervision of Professor Mohammad S. Khajavi at Shahid Beheshti University (SBU), Tehran, Iran, in 1997, and completed his Ph.D. Degree in the same subject under the supervision of Professor Firouz Matloubi Moghaddam at Sharif University of Technology (SUT) Tehran, Iran, in 2002. During the Ph.D. program (2001), Dr. Dekamin spent 8 months in the research group of Professor James H. Clark, in the Clean Technology Center at The University York, UK as visiting scholar. He joined the faculty of the Department of Chemistry at Isfahan University of Technology in July 2003. In October 2004, he moved to Iran University of Science and Technology (IUST), Tehran, Iran, where he is currently Associate Professor of Organic Chemistry (Since May, 2011). Dr. Dekamin also took a sabbatical leave for 2 months in the research group of Professor Takeshi Endo (Emeritus Professor at Tokyo Institute of Technology) at the Molecular Engineering Institute (MEI), Kinki University, Iizuka, Fukuoka, Japan from July 2012 to September 2012. Now, 8 Ph.D students and 10 MSc. Students are working under his supervision. He has published more than 60 peer-reviewed papers in the field of organic chemistry. His research interests are green chemistry, heterogeneous catalysis, nanomaterials, natural and synthetic organocatalysts, and supramolecular chemistry.
ORCID
Mohammad G. Dekamin http://orcid.org/0000-0002-7018-7363
Additional information
Funding
References
- Sheldon, R. A. Green Chem. 2005, 7, 267–278. doi: 10.1039/b418069k
- Geiser, K. In Sustainability Science and Engineering;Abraham, M. A., Ed.; Elsevier: Amsterdam, 2006; pp 161–175.
- Dekamin, M. G.; Eslami, M. Green Chem. 2014, 16, 4914–4921. doi: 10.1039/C4GC00411F
- Dekamin, M. G.; Alikhani, M.; Emami, A.; Ghafuri, H.; Javanshir, S. J. Iran. Chem. Soc. 2016, 13, 591–596. doi: 10.1007/s13738-015-0793-7
- Dekamin, M. G.; Eslami, M.; Maleki, A. Tetrahedron 2013, 69, 1074–1085. doi: 10.1016/j.tet.2012.11.068
- Srinivasa Reddy, B.; Naidu, A.; Dubey, P. K. Green Chem. Lett. Rev. 2013, 6, 254–261. doi: 10.1080/17518253.2012.742142
- Zeng, H.; Tian, Q.; Shao, H. Green Chem. Lett. Rev. 2011, 4, 281–287. doi: 10.1080/17518253.2011.571717
- Kidwai, M.; Mishra, N. K.; Bhatnagar, D.; Jahan, A. Green Chem. Lett. Rev. 2011, 4, 109–115. doi: 10.1080/17518253.2010.512565
- Zarei, M.; Ameri, M. A.; Jamaleddini, A. J. Sulfur Chem. 2013, 34, 259–263. doi: 10.1080/17415993.2012.733005
- Chen, J.; Spear, S. K.; Huddleston, J. G.; Rogers, R. D. Green Chem. 2005, 7, 64–82. doi: 10.1039/b413546f
- Karnakar, K.; Narayana Murthy, S.; Ramesh, K.; Satish, G.; Nanubolu, J. B.; Nageswar, Y. V. D. Tetrahedron Lett. 2012, 53, 2897–2903. doi: 10.1016/j.tetlet.2012.03.135
- Marqués-López, E.; Herrera, R. P. Multicomponent Reactions: Concepts and Applications for Design and Synthesis, John Wiley & Sons, Inc: Hoboken, New Jersey, 2015; pp 382–415.
- Srikrishna, D.; Dubey, P. K. Tetrahedron Lett. 2014, 55, 6561–6566. doi: 10.1016/j.tetlet.2014.10.021
- Ghanbari, M. M. Green Chem. Lett. Rev. 2016, 9, 203–207. doi: 10.1080/17518253.2016.1231841
- Ghasemzadeh, M. A.; Abdollahi-Basir, M. H. Green Chem. Lett. Rev. 2016, 9, 156–165. doi: 10.1080/17518253.2016.1204013
- Edjlali, L.; Vessally, E.; Jafari, Z.; Esrafili, M. D. Green Chem. Lett. Rev. 2016, 9, 13–19. doi: 10.1080/17518253.2015.1116617
- Dekamin, M. G.; Azimoshan, M.; Ramezani, L. Green Chem. 2013, 15, 811–820. doi: 10.1039/c3gc36901c
- Dekamin, M. G.; Ilkhanizadeh, S.; Latifidoost, Z.; Daemi, H.; Karimi, Z.; Barikani, M. RSC Adv. 2014, 4, 56658–56664. doi: 10.1039/C4RA11801D
- Dekamin, M. G.; Mokhtari, Z. Tetrahedron 2012, 68, 922–930. doi: 10.1016/j.tet.2011.10.087
- Huang, Y.; Dömling, A. Mol. Diversity 2010, 15, 3–33. doi: 10.1007/s11030-010-9229-6
- Wu, C.; Decker, E. R.; Blok, N.; Bui, H.; You, T. J.; Wang, J.; Bourgoyne, A. R.; Knowles, V.; Berens, K. L.; Holland, G. W.; Brock, T. A.; Dixon, R. A. F. J. Med. Chem. 2004, 47, 1969–1986. doi: 10.1021/jm030528p
- Ghanbari Pirbasti, F.; Mahmoodi, N. O.; Abbasi Shiran, J. J. Sulfur Chem. 2016, 37, 196–210. doi: 10.1080/17415993.2015.1122009
- Shien Yen, M.; Wang, I. J. Dyes Pigm. 2004, 61, 243–250. doi: 10.1016/j.dyepig.2003.10.015
- Zali-Boeini, H.; Pourjafarian, F. J. Sulfur Chem. 2012, 33, 639–645. doi: 10.1080/17415993.2012.733006
- Jacobson, K. A.; Gao, Z. G. Nat. Rev. Drug Discovery 2006, 5, 247–264. doi: 10.1038/nrd1983
- Duffy, J. L.; Kirk, B. A.; Konteatis, Z.; Campbell, E. L.; Liang, R.; Brady, E. J.; Candelore, M. R.; Ding, V. D. H.; Jiang, G.; Liu, F.; Qureshi, S. A.; Saperstein, R.; Szalkowski, D.; Tong, S.; Tota, L. M.; Xie, D.; Yang, X.; Zafian, P.; Zheng, S.; Chapman, K. T.; Zhang, B. B.; Tata, J. R. Bioorg. Med. Chem. Lett. 2005, 15, 1401–1405. doi: 10.1016/j.bmcl.2005.01.003
- Sabnis, R. W.; Rangnekar, D. W.; Sonawane, N. D. J. Heterocycl. Chem. 1999, 36, 333–345. doi: 10.1002/jhet.5570360203
- Huang, X.; Liu, J.; Ren, J.; Wang, T.; Chen, W.; Zeng, B. Tetrahedron 2011, 67, 6202–6205. doi: 10.1016/j.tet.2011.06.061
- Moeinpour, F.; Dorostkar, N.; Vafaei, M. Synth. Commun. 2012, 42, 2367–2374. doi: 10.1080/00397911.2011.557175
- Rezaei-Seresht, E.; Tayebee, R.; Yasemi, M. Synth. Commun. 2013, 43, 1859–1864. doi: 10.1080/00397911.2012.674607
- Zhao, D. D.; Li, L.; Xu, F.; Wu, Q.; Lin, X. F. J. Mol. Catal. B: Enzym. 2013, 95, 29–35. doi: 10.1016/j.molcatb.2013.05.014
- Tayebee, R.; Javadi, F.; Argi, G. J. Mol. Catal. A: Chem. 2013, 368–369, 16–23. doi: 10.1016/j.molcata.2012.11.011
- Hu, Y.; Wei, P.; Huang, H.; Han, S. Q.; Ouyang, P. K. Synth. Commun. 2006, 36, 1543–1548. doi: 10.1080/00397910600588819
- Castanedo, G. M.; Sutherlin, D. P. Tetrahedron Lett. 2001, 42, 7181–7184. doi: 10.1016/S0040-4039(01)01470-8
- Frutos Hoener, A. P.; Henkel, B.; Gauvin, J. C. Synlett 2003,63–66.
- Zhang, H.; Yang, G.; Chen, J.; Chen, Z. Synthesis 2004,3055–3059.
- Feroci, M.; Chiarotto, I.; Rossi, L.; Inesi, A. Adv. Synth. Catal. 2008, 350, 2740–2746. doi: 10.1002/adsc.200800503
- Tabassum, S.; Govindaraju, S.; Khan, R. U. R.; Pasha, M. A. Ultrason. Sonochem. 2015, 24, 1–7. doi: 10.1016/j.ultsonch.2014.12.006
- Cravotto, G.; Cintas, P. Chem. Soc. Rev. 2006, 35, 180–196. doi: 10.1039/B503848K
- Ashokkumar, M.; Mason, T. J. Kirk-Othmer Encyclopedia of Chemical Technology, John Wiley & Sons, Inc: New York, 2000.
- Reddy, M. B. M.; Jayashankara, V. P.; Pasha, M. A. Green Chem. Lett. Rev. 2013, 6, 107–112. doi: 10.1080/17518253.2012.710650
- Cella, R.; Stefani, H. A. Tetrahedron 2009, 65, 2619–2641. doi: 10.1016/j.tet.2008.12.027
- Santos, H. M.; Lodeiro, C.; Capelo-Martínez, J. L. The Power of Ultrasound, Wiley-VCH Verlag GmbH & Co. KGaA: Weinheim, 2009. Vol. 171.
- Kumar, V. B.; Porat, Z. e.; Gedanken, A. Ultrason. Sonochem. 2016, 28, 367–375. doi: 10.1016/j.ultsonch.2015.08.005
- Bai, R.; Liu, P.; Yang, J.; Liu, C.; Gu, Y. ACS Sustainable Chem. Eng. 2015, 3, 1292–1297. doi: 10.1021/sc500763q
- Kaki, V. R.; Akkinepalli, R. R.; Deb, P. K.; Pichika, M. R. Synth. Commun. 2015, 45, 119–126. doi: 10.1080/00397911.2014.951898
- Abaee, M. S.; Cheraghi, S. J. Sulfur Chem. 2014, 35, 261–269. doi: 10.1080/17415993.2013.860141
- Mojtahedi, M. M.; Abaee, M. S.; Mahmoodi, P.; Adib, M. Synth. Commun. 2010, 40, 2067–2074. doi: 10.1080/00397910903219435
- Shaabani, A.; Hooshmand, S. E.; Afaridoun, H. Monatsh. Chem. 2017, 148, 711–716. doi: 10.1007/s00706-016-1787-6