ABSTRACT
A new rust converter, 3,4,5-trihydroxy-2-[(hydroxyimino) methyl] benzoic acid (named as RC-GAO), has been synthesized based on gallic acid by formylation and oximation. The synthesis was confirmed by Fourier transform infrared spectroscopy studies. The nature and morphology of rust and conversion layer on the rusty samples were characterized by scanning electron microscopy and X-ray diffraction. The corrosion resistance of the residual rust coatings containing the rust converter on the rusty sample was measured by the salt spray test. The results showed that the synthesized rust converter possessed excellent chelating capability with rust and significant anticorrosive performance.
The synthesized rust converter revealed excellent chelating capability with rust and significant anticorrosive performance
GRAPHICAL ABSTRACT
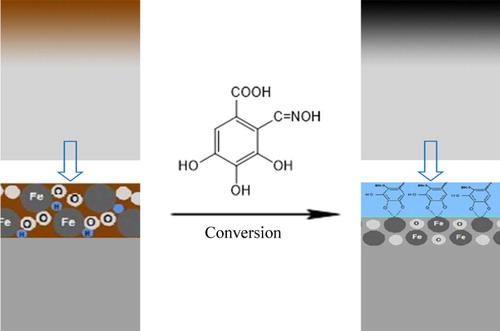
1. Introduction
Metal corrosion is a serious problem all over the world and therefore a number of methods and materials have been developed to minimize the corrosion processes and environment problems (Citation1–6). Among the frequently reported protection methods to prevent metal corrosion, such as organic or inorganic coating (Citation7, Citation8), electrochemical protection (Citation9) and corrosion inhibition (Citation10–13), the most effective and economic technique is the application of coating, particularly anticorrosive coatings (Citation14, Citation15). Metal surfaces require cleaning process such as acid pickling or abrasive blasting before the modification with anticorrosive coating (Citation16). Nevertheless, these cleaning procedures can hardly be performed on irregular surfaces and generate pollution that is harmful to the health of operators. In contrast, rust converters can be directly applied to the corroded surfaces, react with iron oxides and generate new compounds that have a passivation effect on the steel to protect the surfaces against possible further attacks (Citation17–19). Residual rust coatings containing the rust converter without the need of pre-derusting has the advantages over the common anticorrosive coatings (Citation20). Nowadays, most studies regarding residual rust coatings are based on tannic and phosphoric acids (Citation21, Citation22). The use of gallic acid and gallic acid ester as the rust converter for the residual rust coatings have been investigated recently (Citation23).
Gallic acid (3,4,5-trihydroxy benzoic acid, GA) as a natural plant polyphenol is widely distributed in fruits and plants (Citation24–26). Gallic acid and its derivatives have multiple biological and pharmacological properties and are widely used in biology, pharmacy, chemistry and so on (Citation27–30). The structure of gallic acid endorses its powerful chelating capability with metal ions through hydroxyl groups (Citation31–33). Oxime(–C9NOH) and oxime ligands are other compounds that have received considerable attention because of their great applications in various fields including anticorrosive coating due to the chelating capability of the oxime with rust (Citation34–37). Therefore, a compound containing both –O–H and –C9NOH based on GA may present excellent chelating capability with rust.
The aim of this work is to synthesize a new rust converter [3,4,5-trihydroxy-2-[(hydroxyimino) methyl] benzoic acid, named as RC-GAO in this paper] based on gallic acid and to examine its rust converting properties on the rusty samples. The successful synthesis of the rust converter was confirmed by Fourier transform infrared spectroscopy (FT-IR). Converting capability of the rust converter was studied by FT-IR, scanning electron microscopy (SEM) and X-ray diffraction (XRD). The corrosion resistance of the residual rust coatings containing the rust converter was measured by the salt spray test. The results showed that the synthesized new rust converter exhibited good rust-chelating ability and significant corrosion resistance.
2. Experimental
2.1. Material
Methylbenzene, methyl alcohol, and N,N-dimethylformamide (DMF) were purchased from Tanjin Bodi Co. Ltd (Tianjin, China). Formaldehyde and hydroxylamine hydrochloride were provided by Tianjin Benchmark Chemical Reagent Co. Ltd (Tianjin, China). Magnesium was purchased from Shanghai Chemical Reagent Co. Ltd (Shanghai, China). Gallic acid was provided by Tianjin Kermel Chemical Reagent Co. Ltd (Tianjin, China). Texanol (the effective chemical composition is 2,2,4-trimethyl-1,3-pentanediolmono (2-methylpropanoate)) was purchased from Eastman Chemical Company (Shanghai, China). All the chemical reagents used in the experiment were analytical reagents and used without any further purification. Distilled water was used throughout the experiments.
2.2. Synthesis of RC-GAO
The synthesis of RC-GAO is illustrated in Scheme 1. The gallic acid first reacted with magnesium methoxide to form gallate that subsequently reacted with formaldehyde to produce the precursor of the desired product. The RC-GAO was then obtained after the oximation reaction.
For a typical reaction, methenol (32 mL) and toluene (17 mL) were mixed in a four-neck bottle with a condenser and a thermometer. The mixture was stirred at 65°C in a temperature-controlled water bath, and magnesium (1 g, 41.1 mmol) was added by multi-addition. The suspension was stirred at 65°C for 6 h then heated up to 75°C. The solution of gallic acid (2 g, 10.6 mmol) in DMF (20 mL) was added drop by drop through constant pressure funnel. After 1 h, formaldehyde (16 ml) was then slowly added into the reaction vessel. After the addition of the formaldehyde, the stirring was continued for 1 h at 65°C. Then, the aqueous solution of hydroxylamine hydrochloride (34 g, 13%) was added. After 3-h continuous agitation and reaction, the four-neck bottle was naturally cooled down to room temperature and the organic liquid was separated by a separatory funnel. The crude product was obtained as a brown liquid.
According to our experimental results, the optimal mass ratio of methyl alcohol, gallic acid, formaldehyde and hydroxylamine hydrochloride for the synthesis of RC-GAO was 32:1:16:4 by single factor experiments.
2.3. Preparation of samples
The cold-rolled plates (the compositions of the cold-rolled steel plates are listed as follows (%) : C: ≤ 0.10, Si: ≤ 0.05, Mn: ≤ 0.50, P: ≤ 0.035, S: ≤ 0.025, Fe: residual dose) of 6 cm × 7 cm × 0.05 cm were degreased with an alkaline degreasing agent which was made in our lab (to be specific, its main compositions are listed as follows (for example to make 1 g degreasing agent): Na4P2O7•10H2O: 0.03 g, Na2CO3: 0.12 g, NaOH: 0.05 g, NaCl: 0.01 g, sodium gluconate: 0.04 g, triethanolamine: 0.08 g, sodium carboxymethylcellulose: 0.002 g, polyoxyethylene nonylphenol ether (NP-10): 0.02 g, and distilled water: residual dose) and rinsed with distilled water, and then exposed to air for one month to rust. The pre-rusted plates were only roughly cleaned to remove the floating rust and dust on the surface of them using a metallic brush and rinsed with ethanol before the test, since the floating rust and dust may affect the adhesion performance of the coating. Then the rust converter synthesized in the present work was painted on the pre-treated corroded plates when the ethanol had evaporated. The coated samples were allowed to be dried for 72 h at room temperature after the treatment. The average thickness of the rust converter was about 15 μm for all samples measured by a thickness gauge. These samples were studied by SEM and XRD.
The compositions of the residual rust coatings are given in . The residual rust coatings containing RC-GAO was painted on the prepared corroded plates. Then, these samples were exposed in a salt spray chamber to test the corrosion resistance.
Table 1. The composition of residual rust coatings.
2.4. Analytical techniques
The as-synthesized rust converter was characterized by FT-IR on a NICOLET 380 spectrometer within the mid-IR range from 4000 to 400 cm−1. Surface morphology of the samples was observed by SEM (Hitachi SU-70, Japan). The type of the rust sample was determined by powder X-ray diffraction patterns equipped with graphite monochromatized Cu Kα radiation (XRD, D/Max2500PC, Rigaku).
The neutral salt spray test was used to evaluate the corrosion resistance of the coatings. The instrument of the neutral salt spray test was a FQY015 pneumatic salt spray corrosion test chamber (Shanghai Experimental Instrument Co. Ltd, (Shanghai, China)) containing sodium chloride (5 ± 0.5) wt.% with a pH value of 6.5–7.2 and a temperature at (47 ± 0.5)°C.
3. Results and discussion
3.1. FT-IR analysis
shows the typical FT-IR spectra of the rust converter, iron rust and iron rust treated with the rust converter. The characteristic absorption spectrum of RC-GAO is shown in (a). A broad peak in the FT-IR spectrum at 3400 cm−1 is attributed to the –O–H stretching vibration. The characteristic peaks at 1656 and 1439 cm−1 are –COO– and –C–N, respectively. The peaks at 1254 and 3025 cm−1 are assigned to –C–H bending vibration and stretching vibration on the benzene ring, respectively. The peaks at 1107 and 692 cm−1 are attributed to the –C–H in plane and –C–H out of plane bending vibrations, respectively. Therefore, the successful synthesis of RC-GAO is confirmed by FT-IR.
Figure 1. IR spectra of (a) iron rust; (b) iron rust treated with rust converter; and (c) the rust converter.
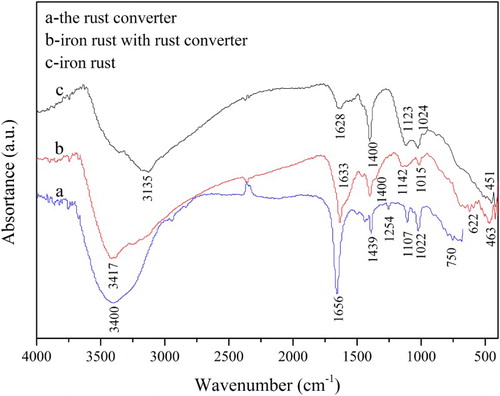
(c) shows the FT-IR spectrum of a typical rusted sample. The broad peak at 3135 cm−1 is related to the –O–H vibrations. The bands at 1024 and 451 cm−1 are the peaks of lepidocrocite and magnetite, respectively. Comparing (b) with 1(c), the peak of lepidocrocite shifts into a broad and noticeable peak at 1015 cm−1. Meanwhile, the peaks at 3135 and 1628 cm−1 in (c) disappeared after treatment. The peaks at 1123 and 1400 cm−1 are weakened and a new peak occurs at 622 cm−1 in (b). The appearance of a new peak at 622 cm−1 is due to the formation of chelation. The change and appearence of peaks suggested that the rust was converted by the rust converter. Comparing (a) with (b), reveals that the strong band of –O–H localized at 3400 cm−1 in (a) seems to be weakened and shifts to 3417 cm−1 in (b), indicating the chelation of –O–H with rust. The comparative analysis proves that iron rust reacted with RC-GAO.
3.2. Morphology analysis
The rusted steel plate surface ((a)) would be transformed into black ((b)) after applying the as-prepared rust converter for about 15 min. This is due to the reaction of the rust and rust converter and the formation of the iron chelate. From (b), we can see that a dense shiny black iron chelate protective film was obtained when the rust converter reacted with rust.
Figure 2. Optical images of the rusty steel sample before (a) and after (b) the treatment with the rust converter. The brown rust in the sample plate is converted into a shiny black protective iron chelate film after the treatment with RC-GAO.
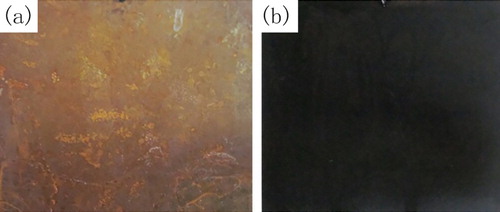
shows the SEM images of rusty samples before and after conversion treatment. (a) shows the original appearance of the rust that is heterogeneous with fine grain and pores of different sizes. Regions of particles with the flakes (lepidocrocite) and globular structure (goethite) can be also observed. The application of the rust converter to the rusty sample surface forms an amorphous compact and cracked layer, with bulging and the presence of bright particles, (b). The bright particles show irregular shapes and different sizes. These particles can correspond to either residual converter or to some amorphous form of converted rust. Compareing (a), the surface of the rusty sample is more homogeneous and smoother after the treatment with the rust converter, (b). It indicates that the RC-GAO can chelate with iron ions.
3.3. XRD analysis
depicts the XRD pattern of the rusted samples before and after treatment with the rust converter. As it can be seen, the main crystal of the rust is lepidocrocite (L, γ-FeOOH). Some peaks of low intensity are also observed, which are goethite (G, α-FeOOH) and magnetite (M, Fe3O4). The surfaces of rusted samples were mainly covered by small crystal of lepidocrocite and magnetite consistent with SEM results. The decrease in peaks corresponding to lepidocrocite, goethite and magnetite observed in testified the reaction between the rust converter and rust.
3.4. Salt spray test analysis
A salt spray corrosion test chamber was utilized for testing the corrosion resistance of the coated samples. The anticorrosion capability of coated samples was evaluated using the cross fork method in terms of corrosion spread around the scratch according to ASTM B117 (Standard Practice for Operating Salt Spray (Fog) Apparatus). The corrosion resistance was evaluated in terms of blistering and degree of rusting according to ASTM D714-02 (Standard Test Method for Evaluating Degree of Blistering of Paints). The blistering is characterized with its size and frequency. The blistering size is graded from 10 to 0, where 10 represents no blistering. Blistering frequency is denoted by D, MD, M, F (dense, medium dense, medium, few) (Citation38, Citation39). The results of the salt spray test are represented in .
Table 2. The result of the salt spray test.
The salt spray corrosion resistance test is the most classic and most widely used accelerated corrosion test method to predict the useful life of the coating by evaluating the corrosion resistance, localized corrosion and pitting of the coating in the world. Only a few blisters and no rust are visible on the surface of samples after 12-h salt spray testing. After 24 h salt spray testing, a small amount of blisters and no rust are observed. Some blisters and little rust can be observed after 120-h salt spray testing as shown in . The results show that the coating film not only can well avoid the pitting corrosion and localized corrosion but also exhibits a significant salt spray resistance
4. Conclusions
We have successfully synthesized a new rust converter, RC-GAO, based on gallic acid. The rust converter presents good rust-chelating ability as confirmed by FT-IR, SEM and XRD. The coatings containing RC-GAO have significant anticorrosive performance confirmed by the salt spray test. The rust converter and its polymer composite coating can be used for metal corrosion protection industries such as petrochemical, power, construction, marine and container manufacturing industries. Moreover, this material after combining with other functional fillers can provide wide potential applications for other fields such as substances separation (Citation40–44) intelligent sensing (Citation45–49), electromagnetic interference (EMI) shielding (Citation50, Citation51), energy harvesting units (Citation52, Citation53), pharmaceutical industry (Citation54), packaging (Citation55) and possible substrate for other functional materials (Citation56) with endured lifetime.
Disclosure statement
No potential conflict of interest was reported by the authors.
Notes on contributors
Yanfei Feng is a teacher in the Resource Environmental Department at Moutai University, received the M.S. degree in Chemical Engineering from Shandong University of Science and Technology. Her research interest focuses on anti-corrosion.
Shengsong Ge is the professor of College of Chemical and Environmental Engineering, Shandong University of Science and Technology. His research interest focuses on anti-corrosion, polymer synthesis and green chemistry.
Jianfeng Li is a graduate student of College of Chemical and Environmental Engineering, Shandong University of Science and Technology. His research interests are anti-corrosion, polymer synthesis and green chemistry.
Shengyao Li is an undergraduate student of School of Materials Science and Engineering, Central South University, carried out the research in the University of Tennessee, Knoxville. His research interests are mechanical properties of alloy materials, biomaterials and polymers.
Huixin Zhang is the Master of College of Chemical and Environmental Engineering, Shandong University of Science and Technology. His research interests are anti-corrosion, polymer synthesis and green chemistry.
Yong Chen is the associate professor of College of Chemical and Environmental Engineering, Shandong University of Science and Technology. His research interest focuses on organic chemistry.
Dr. Zhanhu Guo, currently an Associate Professor of Chemical and Biomolecular Engineering at University of Tennessee, obtained a Chemical Engineering PhD degree from Louisiana State University (2005) and received three-year (2005-2008) postdoctoral training in Mechanical and Aerospace Engineering Department in University of California Los Angeles. Dr. Guo directs the Integrated Composites Laboratory and chaired the Composite Division of American Institute of Chemical Engineers (AIChE, 2010-2011). His current research focuses on multifunctional nanocomposites for anti-corrosion, energy harvesting, electronic devices, environmental remediation, fire retardancy, and electromagnetic radiation shielding/absorption applications.
References
- Etteyeb, N.; Sanchez, M.; Dhouibi, L.; Alonso, C.; Andrade, C.; Triki, E. Corros. Eng. Sci. Techn. 2006, 41 (4), 336–341. doi: 10.1179/174327806X120775
- Zhang, J.-X.; Liang, Y.-X.; Wang, X.-J.; Zhou, H.-J.; Li, S.-Y.; Zhang, J.; Feng, Y.-N.; Lu, N.; Wang, Q.; Guo, Z.-H. Adv. Compos. Hybrid Mater. 2017. doi:10.1007/s42114-017-0007-0., in press
- Petek, A.; Kovačič, S. Green Chem. Lett. Rev. 2015, 7 (4), 337–341. doi: 10.1080/17518253.2014.952684
- Yang, X.-B.; Jiang, X.; Huang, Y.-D.; Guo, Z.-H.; Shao, L. ACS Appl. Mater. Inter. 2017, 9 (6), 5590–5599 doi: 10.1021/acsami.6b15098
- Xu, Y.-C.; Tang, Y.-P.; Liu, L.-F.Guo, Z.-H.; Shao, L. J. Membrane Sci. 2017, 526, 32–42. doi: 10.1016/j.memsci.2016.12.026
- Zhang, D.-Q.; Williams, B.L.; Becher, E.M.; Shrestha, S.B.; Nasir, Z.; Lofink, B.J.; Santos, V.H.; Patel, H.; Peng, X.-H.; Sun, L.-Y. Adv. Compos. Hybrid Mater. 2017. doi:10.1007/s42114-017-0006-1., in press
- Huang, M.-X.; Zhang, H.; Yang, J.-L. Corros. Sci. 2012, 65, 561–566. doi: 10.1016/j.corsci.2012.08.020
- Jalili, M.M.; Moradian, S.; Hosseinpour, D. Constr. Build. Mater. 2009, 23(1), 233–238. doi: 10.1016/j.conbuildmat.2007.12.011
- Rahman, H.H.A.; Seleim, S.M.; Hafez, A.M.; Helmy, A.A. Green Chem. Lett. Rev. 2015, 8 (3–4), 88–94. doi: 10.1080/17518253.2015.1111430
- Díaz-Cardenas, M.Y.; Valladares-Cisneros, M.G.; Lagunas-Rivera, S.; Salinas-Bravo, V.M.; Lopez-Sesenes, R.; Gonzalez-Rodríguez, J.G. Green Chem. Lett. Rev. 2017, 10 (4), 257–268. doi: 10.1080/17518253.2017.1369167
- Fouda, A.S.; Elewady, G.; Ali, A.H. Green Chem. Lett. Rev. 2017, 10 (2), 88–100. doi: 10.1080/17518253.2017.1299228
- Torres, A.R.; Cisneros, M.G.V.; Rodríguez, J.G.G. Green Chem. Lett. Rev. 2016, 9 (3), 143–155. doi: 10.1080/17518253.2016.1195017
- Verma, D.K.; Khan, F. Green Chem. Lett. Rev. 2016, 9 (1), 52–60. doi: 10.1080/17518253.2015.1137976
- Ahmed, M.S.; Munroe, P., Jiang, Z.-T.; Zhao, X.-L.; Rickard, W.; Zhou, Z.-F.Kwok, L.; Lie, Y.; Xie, Z.-H. Corros. Sci. 2011, 53 (11), 3678–3687. doi: 10.1016/j.corsci.2011.07.011
- Wang, X.-M.; Zhu, M.-Q.; Lan, S.; Ginder-Vogel, M.; Liu, F.; Feng, X.-H. Chem. Geol. 2015, 415, 37–46. doi: 10.1016/j.chemgeo.2015.09.009
- Viguri, J.; Andres, A.; Ibanez, R.; Puente, C.R.; Irabien, A. J. Hazard. Mater. 2000, 79 (1), 63–75. doi: 10.1016/S0304-3894(00)00248-X
- Devi, A.; Singhal, A.; Gupta, R.; Panzade, P. Clean Technol. Envir. 2014, 16 (8), 1515–1527. doi: 10.1007/s10098-014-0726-7
- Barrero, C.A.; Ocampo, L.M.; Arroyave, C.E. Corros. Sci. 2001, 43 (6), 1003–1018. doi: 10.1016/S0010-938X(00)00139-6
- Collazo, A.; Novoa, X.R.; Perez, C.; Puga, B. Electrochim. Acta 2009, 55 (21), 6156–6162. doi: 10.1016/j.electacta.2009.09.084
- Collazo, A.; Novoa, X.R.; Perez, C.; Puga, B. Electrochim. Acta 2007, 53 (25), 7565–7574. doi: 10.1016/j.electacta.2007.11.078
- Singh, D.D.N.; Yadav, S. Surf. Coat. Tech. 2007, 202 (8), 1526–1542. doi: 10.1016/j.surfcoat.2007.07.013
- Zhang, S.-F.; Zhang, R.-F.; Li, W.-K.; Li, M.-S.; Yang, G.-L. Surf. Coat. Tech. 2012, 207 (25), 170–176. doi: 10.1016/j.surfcoat.2012.06.056
- George, F.O.; Skeldon, P.; Thompson, G.-E. Corros. Sci. 2012, 65, 231–237. doi: 10.1016/j.corsci.2012.08.031
- Kaur, R.; Guru Row, T.N. Cryst. Growth Des. 2012, 12 (6), 2744–2747. doi: 10.1021/cg300536n
- Paema, V.V.; Sowmya, P.; Felix, T.A.; Baskaran, R.; Poornima, P. Food Chem.Toxicol. 2011, 49 (4), 991–998. doi: 10.1016/j.fct.2011.01.005
- Parihar, S.; Gupta, A.; Chaturvedi, A.K.; Agarwal, J.; Luqman, S.; Changkija, B.; Manohar, M.; Chanda, D.; Chanotiya, C.S.; Shanker, K.; Dwivedi, A.; Konwar, R.; Negi, A.S. Steroids 2012, 77 (8), 878–886. doi: 10.1016/j.steroids.2012.03.012
- Garcia-Rivera, D.; Delgado, R.; Bougarne, N.; Haegeman, G.; Berghe, W.V. Cancer Lett. 2011, 305 (1), 21–31. doi: 10.1016/j.canlet.2011.02.011
- Latha, R.C.R.; Daisy, P. Chem-Bio. Interact. 2011, 189 (1), 112–118. doi: 10.1016/j.cbi.2010.11.005
- Nabavi, S.F.; Habtemariam, S.; Jafari, M.; Sureda, A.; Nabavi , S.M.B. Environ. Contam. Tox. 2012, 89 (1), 73–77. doi: 10.1007/s00128-012-0645-4
- Tang, B.; Yuan, H.; Cheng, L.; Zhou, X.-D.; Huang, X.-L.; Li, J.-Y. Arch. Oral Biol. 2015, 60 (1), 167–173. doi: 10.1016/j.archoralbio.2014.09.011
- Liang, Z.-X.; Zhai, H.-Y.; Chen, Z.G.; Wang, H.-H.; Zhou, Q.; Huang, X.-T. Sensor. Actuat. B-Chem. 2016, 224, 915–925. doi: 10.1016/j.snb.2015.10.101
- Chandrappa, S.; Umashankara, M.; Vinaya, K.; Ananda Kumar, C.S.; Rangappa, K.S. Tetrahedron Lett. 2012, 53 (21), 2632–2635. doi: 10.1016/j.tetlet.2012.03.052
- Zhang, Q.-A.; Shen, H.; Fan, X.-H.; Shen, Y.; Wang, X.; Song, Y. Ultrason. Sonochem. 2015, 22, 149–154. doi: 10.1016/j.ultsonch.2014.06.010
- Criado, P.; Fraschini, C.; Salmieri, S.; Becher, D.; Safrany, A.; Lacroix, M. Radiat. Phys. Chem. 2016, 118, 61–69. doi: 10.1016/j.radphyschem.2015.05.030
- Müller, B.; Kubitzki, G.; Kinet, G. Corros. Sci. 1998, 40, 1469–1477. doi: 10.1016/S0010-938X(98)00058-4
- Takács, M.; Bubenyák, M.; Váradi, A.; Blazics, B.; Horváth, P.; Kökösi, J. Tetrahedron Lett. 2011, 52(16), 1863–1865. doi: 10.1016/j.tetlet.2011.02.024
- Lin, M.-H.; Lin, L.-Z.; Chuang T.-H.; Liu, H.-J. Tetrahedron. 2012, 68 (12), 2630–2635. doi: 10.1016/j.tet.2012.01.074
- Zhang, K.; Song, Y.-S.; Zhong, D.-H.Shao, Z.-H.; Zhou, L.-Q. Adv. Mater. Res. 2011, 311, 1847–1851.
- Wang, S.-W.; Morelos-Gomez, A.; Lei, Z.-W.; Terrones, M.; Takeuchi, K.; Sugimoto, W.; Endo, M.; Kaneko, K. Carbon 2016, 96, 174–183. doi: 10.1016/j.carbon.2015.09.017
- Cheng, X.-Q.; Ding, S.-G.; Guo, J.; Zhang, C.; Guo, Z.-H.; Shao, L. J. Membrane Sci. 2017, 536, 19–27. doi: 10.1016/j.memsci.2017.04.057
- Li, Y.-C.; Wu, X.-L.; Song, J.-F.; Li, J.-F.; Shao, Q.; Cao . N.; Lu, N.; Guo, Z.-H. Polymer 2017, 124, 41–47. doi: 10.1016/j.polymer.2017.07.042
- Yu, G.-Q.; Lu, Y.; Guo, J.; Patel, M.; Bafana, A.; Wang, X.-F.; Qiu, B.; Jeffrtes, C.; Wei, S.-Y.; Guo, Z.-H. Adv. Compos. Hybrid Mater. 2017. doi:10.1007/s42114-017-0004-3., in press.
- Ma, Y.-L.; Lv, L.; Guo, Y.-R.; Fu, Y.-J.; Shao, Q.; Wu, T.-T.; Guo, S.-J.Sun, K.; Guo, X.-K.; Wujcik, E.K.; Guo, Z.-H. Polymer 2017, 128, 12–23. doi: 10.1016/j.polymer.2017.09.009
- Zhang, Y.-Q.; Yang, X.-B.; Wang, Z.-X.; Long, J.; Shao, L. 2017,J. Mater. Chem. A 2017, 5 (16), 7316–7325. doi: 10.1039/C6TA11252H
- Alippi, C. CAAI Trans. Intellig. Technol. 2016, 1, 1–3. doi: 10.1016/j.trit.2016.03.002
- Jin, H.-Y.; Chen, Q.; Chen, Z.-X.; Hu, Y.; Zhang, J.W. CAAI Trans. Intellig. Technol. 2016, 1, 104–113. doi: 10.1016/j.trit.2016.03.010
- Sun, K.; Xie, P.-T.; Wang, Z.-Y.; Su, T.-M.; Shao, Q.; Ryu, J.E.; Zhang, X.H.; Guo, J.; Shankar, A.; Li, J.-F.; Fan, R.-H.; Cao, D.-P.; Guo, Z.-H. Polymer 2017, 125, 50–57. doi: 10.1016/j.polymer.2017.07.083
- Zhang, X.-Y.; Gao, H.-B.; Guo, M.; Li, G.-P.; Liu, Y.-C.; Li, D.Y. CAAI Trans. Intellig. Technol. 2016, 1, 4–13. doi: 10.1016/j.trit.2016.03.003
- Padhy, S.; Panda, S. CAAI Trans. Intellig. Technol. 2017, 2, 12–25. doi: 10.1016/j.trit.2017.01.002
- Cheng, C.-B.; Fan, R.-H.; Wang, Z.-Y.; Shao, Q.; Guo, X.-K.; Xie, P.-T.; Yin, Y.-S.; Zhang, Y.-Z.; An, L.-Q.; Lei, Y.-H.; Ryu, J.-E.; Shankar, A.Guo, Z.-H. Carbon 2017, 125, 103–112. doi: 10.1016/j.carbon.2017.09.037
- Zhang, K.; Li, G.-H.; Feng, L.-M.; Wang, N.; Guo, J.; Sun, K.; Yu, K.-X.; Zeng, J.-B.; Li, T.-X.; Guo, Z.-H.; Wang, M. J. Mater. Chem. C 2017, 5, 9359–9369. doi: 10.1039/C7TC02948A
- Liu, T.; Yu, K.; Gao, L.-N.; Chen, H.; Wang, N.; Hao, L.-H.; Li, T.-X.; He, H.-C.; Guo, Z.-H. J. Mater. Chem. A 2017, 5, 17848–17855. doi: 10.1039/C7TA05123A
- Sun, Z.-Y.; Zhang, L.; Dang, F.; Liu, Y.; Fei, Z.-Y.; Shao, Q.; Lin, H.; Guo, J.; Xiang, L.-C.; Yerrd, N. Cryst Eng Comm. 2017, 19, 3288–3298. doi: 10.1039/C7CE00279C
- Zheng, Y.; Zheng, Y.; Yang, S.; Guo, Z.; Zhang, T.; Song, H.; and Shao, Q. Green Chem. Lett. Rev. 2017, 10, 202–209. doi: 10.1080/17518253.2017.1342001
- Yang, W.; Wang, X.; Li, J.; Yan, X.; Ge, S.; Tadakamalla, S. Polym. Eng. Sci., 2017, in press, doi:10.1002/pen.24675.
- P. Zhou, S. Wang, C. Tao, X. Guo, L. Hao, Q. Shao, L. Liu, Y. Wang, W. Chu, B. Wang, S. Luo and Z. Guo, Adv. Polym. Technol., 2017, in press, doi:10.1002/adv.21908.