ABSTRACT
In this work, pure MCM-41 mesoporous silica with active mesoporous sites has been successfully applied, as a highly efficient and recoverable catalyst, for the rapid and convenient synthesis of α-aminonitriles and imines. Various imines, as the intermediate of the Strecker reaction, were simply prepared from condensation of a wide range of aldehydes and amines in the presence of low loading of MCM-41 mesoporous silica under solvent-free conditions at room temperature in high to quantitative yields. Furthermore, the corresponding α-aminonitrile derivatives were prepared through the three-component Strecker reaction using trimethylsilylcyanide catalyzed by MCM-41 as a bifunctional heterogeneous mesoporous solid catalyst.
GRAPHICAL ABSTRACT

Introduction
Bifunctional compounds such as α-aminonitriles are extremely useful synthetic intermediates. α-Aminonitriles serve as significantly valuable precursors for the synthesis of natural or synthetic α-amino acids, diamines and various nitrogen containing heterocycles such as thiadiazoles, imidazoles and indoles ( Citation1–7). Indeed, these bifunctional compounds have received considerable attention due to their remarkable biological activities such as anticancer, antibacterial, antifungal, antibiotic and antiviral properties ( Citation5, Citation6, Citation8, Citation9).
The Strecker reaction which was first reported in 1850 ( Citation10) is the simplest and the most efficient method for the formation of carbon–carbon and carbon-heteroatom bonds required for the preparation of non-proteinogenic α-amino acids from corresponding α-aminonitriles in both lab and industrial scales. Furthermore, it has been known as the oldest reaction in the field of multicomponent reactions (MCRs) which is an attractive area of research in the modern organic chemistry ( Citation11, Citation12). Some typical pharmacologically useful compounds which can be prepared through the Strecker reaction, as one of the key steps, include saframycin A ( Citation9, Citation13–15), Phthalascidin ( Citation16–18), (R)-phenylglycinol derivatives ( Citation19), Ecteinascidin 743 ( Citation14, Citation20), (S)-Clopidogrel (Plavix®) ( Citation21, Citation22) and Captopril () ( Citation23, Citation24). Accordingly, different catalytic methods have been developed for synthesis of α-aminonitrile derivatives through the Strecker MCR strategy ( Citation25).
Figure 1. Chemical structure of some pharmaceutically important compounds prepared by the Strecker reaction.
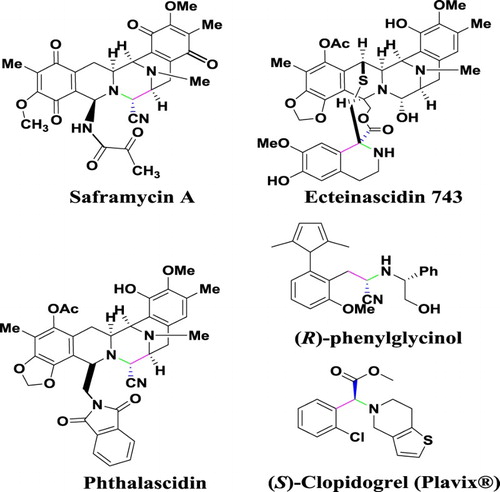
Several modifications of the traditionally Strecker reaction have been reported using a variety of alternative cyanide sources (
Citation10). Indeed, to overcome problems encountered with the use of NaCN, KCN and HCN, other cyanide sources such as trimethylsilylcyanide (TMSCN) (
Citation26), Bu3SnCN (
Citation27), Et2AlCN (
Citation28), Me2C(OH)CN (
Citation29), (EtO)2P(O)CN (
Citation30) and K4[Fe(CN)6] (
Citation31) have been examined. Among these alternative cyanide sources, TMSCN is a safer and more easily handled reagent compared to other cyanide sources (
Citation4). However, TMSCN requires a catalyst to promote the Strecker reaction. Therefore, different homogeneous and heterogeneous Lewis or Bronested acids, metal complexes and biopolymers have been introduced as catalysts for the Strecker reaction. For example, K2PdCl4 or the NHC-amidate palladium(II) complex (
Citation32), NiCl2 (
Citation33), RhI3.H2O (
Citation34), InI3 (
Citation35), NbCl5 (
Citation36), BiCl3 (
Citation4), BiBr3 (
Citation37), Fe3O4 (
Citation38), Fe3O4@ZrO2/ (
Citation39), Fe3O4@SiO2@Me/Et-PhSO3H or Fe3O4@SiO2@PrNHSO3H (
Citation19), Fe(Cp)2PF6 (
Citation40), Cu(OTf)2 (
Citation41), Yb(OTf)3 (
Citation42), Ga-TUD-1 (
Citation43), Ga(OTf)3 (
Citation44), GaCl3 (
Citation45), CeCl3 (
Citation46), Pr(OTf)3 (
Citation47), RuCl3 (
Citation48), La(NO3)3.6H2O (
Citation49), diethylphosphorocyanidated (
Citation30), I2 (
Citation50), FeCl3 (
Citation51), NH2SO3H (
Citation52), l-proline (
Citation53), p-toluenesulfonic acid (
Citation54), nano-sized TiO2 (
Citation55), Sn-montmorillonite (
Citation56), o-benzenedisulfonimide (
Citation57), sulfuric acid-modified PEG-6000 (PEG-OSO3H) (
Citation58), xanthan sulfuric acid (
Citation59), cellulose sulfuric acid (
Citation60), Nafion-SAC-13 and Nafion-H (
Citation61), and chitosan (
Citation62) in conventional organic solvents have been developed in past two decades. Furthermore, a few heterogeneous mesoporous nano-ordered silica modified by transition metal cations or strong Bronsted acid centers such as Co/SBA-15 (
Citation63, Citation64), Zr-MCM-41 (
Citation65), B-MCM-41 (
Citation66), SBA-15-Ph-Pr-SO3H (
Citation67) and MCM-41-SO3H (
Citation68) have been introduced in recent years. However, most of these homogeneous or heterogeneous catalytic systems and modifications require the use of expensive reagents, harsh reaction conditions, lengthy reaction times, the use of toxic organic solvents, low yields as well as tedious work-up leading to the generation of a large amount of toxic waste (
Citation32, Citation34–37, Citation42, Citation43, Citation50, Citation61). Consequently, the use of cost-effective and recyclable catalysts as well as avoiding toxic or volatile organic solvents can be a major effort to improve clean and green synthesis of α-aminonitriles (
Citation62–64, Citation67).
In general, one of the fundamental aspects of development of new instrumental alternative processes is minimizing waste in chemical synthesis leading to environmental protection from pollution. For this purpose, attempts to find alternative and environmentally benign synthetic routes are one of the major aims in the protection of the environment and essential for the development of life and life processes ( Citation69, Citation70). The development of porous materials with large specific surface areas (>1000 m2.g−1) such as silica-based mesoporous has attracted significant attention with regard to their potential applications in the adsorption, chromatography, gas storage, sensor technology and recoverable solid catalysts in organic synthesis ( Citation71). In the case of solid-activated silica-based mesoporous catalysts, mesoporous materials were used as solid support in previous studies performed by our or other research groups ( Citation63–68). Hence, different methods including replacement of Si atoms in the matrix by metal ions such as Al ( Citation72), B or Fe ( Citation66), and Zr ( Citation65) or anchoring acidic organic groups such as 3-sulfonic propyl ( Citation67, Citation73) and inorganic sulfonic acid (−SO3H) ( Citation68) have been described. In continuation of our interest to develop more environmentally benign catalytic systems for different organic transformations ( Citation62, Citation66, Citation74, Citation75), we wish herein to report the pure MCM-41 (1a) as an efficient catalyst for rapid and convenient Strecker reaction of different aldehydes under solvent-free conditions to afford corresponding α-aminonitriles in high to excellent yields (, A). Also, the corresponding imines, as the Strecker reaction intermediates, were easily prepared and isolated under the same reaction conditions in the absence of TMSCN (, B).
Results and discussion
In order to optimize the reaction conditions, condensation of 4-cholorobenzaldehyde (2a, 1 mmol), aniline (3a, 1 mmol) and TMSCN (1.2 mmol), as the model reaction, was investigated in the presence of both silica-based mesoporous materials (MCM-41 and SBA) as well as commercial silica (SiO2) under solvent-free conditions at room temperature. The results are summarized in . It is noteworthy that only a trace amount of 2-(4-chlorophenyl)-2-(phenylamino)acetonitrile (4a) was obtained in the absence of any catalyst. Interestingly, the yield of the desired product 4a was significantly improved in the presence of catalytic amount of MCM-41 (1a), SBA-15 (1b) and the commercial SiO2 (1c). Because of providing higher specific surface area by both MCM-41 (1a) and SBA-15 (1b), they provided a higher yield than the commercial SiO2 (1c) at same catalyst loading. On the other hand, MCM-41 (1a) afforded a higher yield compared to SBA-15. Therefore, the reaction conditions were optimized with regard to various MCM-41 (1a) loading in the next experiments (entries 5 and 6).
Table 1. Three-component Strecker reaction of 4-chlorobenzaldehyde (2a) and aniline (3a) with TMSCN under solvent-free conditions.a
Encouraged by these results, the optimized conditions (MCM- 41, 6 mg; solvent-free conditions; r.t.) were developed to different carbocyclic or heterocyclic aromatic aldehydes 2a–o and different amines 3a–c to afford desired α-aminonitriles 4a–x. The results are summarized in . The desired α-aminonitriles 4a–n were successfully prepared using aniline 3a with a variety of aldehydes (entries 1–14). The reaction also proceeded smoothly using other amines such as p-toluidine 3b and benzylamine 3c to afford the corresponding α-aminonitriles 4o–x in high to excellent yields (entries 15–24). In general, solid products are precipitated out from the initial liquid mixture containing the suspended MCM-41. On the other hand, the oily products afforded viscose mixtures.
Table 2. Three-component Strecker reaction of various aldehydes 2 and amines 3 with TMSCN catalyzed by pure MCM-41 (1a) under optimal conditions.a
After completion of the reaction, the catalyst 1a can be easily separated from the crude products by suspending the reaction mixture in hot EtOH and subsequent filtration. Indeed, aldehydes containing electron-withdrawing groups (2a,d–f) react faster than those bearing electron-donating groups (2h-k) depending on the used amine. Exceptions are 2-chlorobenzaldehde (2b) and 4-bromobenzaldehde (2c) which require longer reaction times (entries 2, 3). The long reaction times required for 2-chlorobenzaldehde (2b) and 4-bromobenzaldehde (2c) can be related to steric hindrance around the aldehyde functional group and lower electron-withdrawing of the bromo substituent compared to chloro at the p ara- position, respectively ( Citation62, Citation71). Furthermore, acid-sensitive substrates such as cinnamaldehyde (2l), furfural (2m), and thiophene-2-carbaldehyde (2n) reacted smoothly under optimized reaction conditions to provide the corresponding products 4l-4n or 4r-s in high to excellent yields without the formation of any polymerization products (entries 12–14, 18, 19) ( Citation49, Citation68).
To our delight, products such as 4l-v and 4x, which have low melting points and their physical state is generally oil at room temperature, were obtained at optimal reaction conditions in high to excellent yields and short to reasonable times (entries 12–22 and 24). Interestingly, these products and other oily products were not formed when chitosan, as a heterogeneous catalyst and full of hydroxyl groups on its polymer chains, was used ( Citation62).
A proposed mechanism for the Strecker reaction catalyzed by pure MCM-41 (1a) is shown in Scheme 2 based on the obtained results as well as our previous studies about the Strecker reaction ( Citation62, Citation66, Citation68) or cyanosilylation of carbonyl compounds with TMSCN ( Citation79), and protection of the hydroxyl functional group of alcohols and phenols with hexamethyldisilazane (Citation80). The high concentration of free hydroxyl groups distributed on the surface of MCM-41 (1a, > 1000 m2.g−1) and its specific pore size (ranging from 20–30 Å) ( Citation71) activate the carbonyl group of the aldehydes 2 through the hydrogen bonding for the subsequent nucleophilic attack of amines (3) to produce the corresponding imines 5. Furthermore, the hygroscopic property of MCM-41 (1a) to adsorb/absorb the water, as by-product of imine 5 formation, may be considered as a driving force for promoting the imine formation in its equilibrium with the carbonyl compound (2) and amine (3) or even intermediate II. In the next step, the hydroxyl groups on the surface of MCM-41 (1a) can activate both imine and TMSCN to produce α-aminonitriles 4 through the hydrogen bonding to the imine functional group and expanding of the d-orbital of silicon atom in TMSCN (intermediate IV), respectively. In a similar pathway, TMSCN can be activated by the water molecules produced as imine formation by-product (intermediate V). According to the observed results summarized in and , it can be deduced that the rate-determining step is addition of cyanide nucleophile on the activated imines (IV) where amine component 3 has been combined with aldehydes 2 before. Therefore, amines 3a–c with different nucleophilicities do not show significant effects on the reaction rate. On the other hand, it should be noted that the formation of imines (5) from aldehydes (2) and amines (3) and subsequent addition of cyanide anion of complexes IV or V to afford the corresponding α-aminonitriles (4) are faster than direct cyanation of carbonyl compounds. Therefore, MCM-41 (1a) can be considered as a simple and efficient bifunctional inorganic catalyst for the Strecker reaction.
The reusability of the separated MCM-41 (1a) was also investigated for the model reaction in further runs. The recovered catalyst after each run was washed three times with EtOAc and then dried at 50 °C for 1 h. Good conversions for the consecutive model reaction with a little loss of its activity was observed. The loss of reactivity of the catalyst (1a) can be attributed to the blocking of its channels by grafting of the trimethylsilyl group from the TMSCN reagent (see intermediate IV in Scheme 2, ).
The catalytic activity of the pure MCM-41 (1a) has also been compared with other previously reported heterogeneous catalysts to show the advantages of the present protocol. The results are summarized in . Comparison of data in clearly demonstrates that a superior environmentally benign method in terms of the catalyst loading, avoiding the use of active Lewis or Bronsted acid reagents and toxic solvents, and required reaction time has been developed.
Table 3. Comparison of the catalytic efficiency of MCM-41 with other heterogeneous catalysts for the synthesis of α-aminonitrile 4g.
Furthermore, a plethora of catalytic systems have been introduced to promote imines 5 formation from corresponding carbonyl compounds 2 and primary amines 3 through a reversible condensation reaction. Imines (5) are also known as the Strecker reaction intermediates ( Citation58, Citation62, Citation68, Citation77). Therefore, we decided to investigate the imine formation from carbonyl compounds (2) and amines (3) in the presence of MCM-41 (1a) under similar conditions to the Strecker reaction. The results of our experiments for this part of our studies about the synthesis of imines (5) are summarized in .
Table 4. Preparation of different imines 5 by the reaction of aldehydes 2 and amines 3 catalysed by MCM-41 (1a).a
Conclusions
The three-component Strecker reaction catalyzed by the mesoporous MCM-41 without any modification using active Lewis or Bronsted acid reagents, as an efficient bifunctional inorganic catalyst, has been reported for the first time. The most important advantages of the present methodology in the Strecker reaction compared to other methodologies are low catalyst loading, excellent yields, short reaction times, avoiding the use of toxic solvents, clean reaction profile, simple work-up procedure and catalyst recyclability.
Experimental section
General
All chemicals and reagents were purchased from Merck or Aldrich and used without further purification except for benzaldehyde, for which a fresh distilled sample was used. MCM-41 was prepared according to the literature ( Citation68). Tetraethylorthosilicate (TEOS) and cetyltrimethylammonium bromide (CTAB) were used as the source of silicon and structure directing agent, respectively. Analytical TLC was carried out using Merck 0.2 mm silica gel 60 F-254 Al-plates. All yields refer to the isolated products after purification. FTIR spectra were recorded as KBr pellets on a Shimadzu FTIR-8400S spectrometer. 1H NMR (500 MHz) spectra were obtained using a Bruker DRX–500 AVANCE spectrometer in CDCl3 at ambient temperature. Melting points were determined using an Electrothermal 9100 apparatus and are uncorrected. All products are known compounds and their structures were established from their spectral data and melting points as compared with the authentic samples or literature values ( Citation62, Citation68, Citation77).
General procedure for preparation of MCM-41 (1a)
A typical MCM-41 preparation is as follows: Diethylamine (2.7 g) was added to deionized water (42 mL) in a 200 mL beaker while the mixture was stirred at room temperature. After 10 min, CTAB (1.47 g) was added to the above solution under stirring step by step for 30 min until a clear solution was obtained. Then, TEOS (2.1 g) was added drop-wise to the solution. The pH of the reaction mixture was adjusted to 8.5 by the slow addition of hydrochloric acid solution (1 M). At this stage, the precipitate was formed. After 2 h, under slow stirring, the solid product was filtered from the mother liquor and washed with deionized water. The sample was dried at 45 °C for 12 h. The obtained white powder was calcined at 550 °C for 5 h to remove any remaining surfactant ( Citation68).
Typical procedure for the synthesis of α-amino nitriles 4a–x catalyzed by MCM-41 (1a)
A mixture of aldehyde (1 mmol), amine (1 mmol), and TMSCN (1.2 mmol, 0.15 mL) in the presence of the MCM-41 (6 mg) was stirred under solvent-free conditions at room temperature. The progress of the reaction was monitored by TLC. After completion of the reaction, the organic product was dissolved in hot EtOH (3 mL) and the mixture was simply filtered to remove the MCM-41, as a heterogeneous catalyst. The solid products were easily recrystallized from EtOH. In the case of oil products, EtOH was evaporated under reduced pressure to afford the essentially pure products ( Citation62, Citation66, Citation68).
Typical procedure for the synthesis of imine 5a–q catalyzed by MCM-41 (1a)
A mixture of aldehyde (1 mmol) and amine (1 mmol) in the presence of the MCM-41 (6 mg) was stirred under solvent-free conditions at room temperature. The progress of the reaction was monitored by TLC. After completion of the reaction, the organic product was dissolved in hot EtOH (3 mL) and the mixture was simply filtered to remove the MCM-41 as a heterogeneous catalyst. The solid products were easily recrystallized from EtOH. In the case of oil products, EtOH was evaporated under reduced pressure to afford the essentially pure products ( Citation62, Citation81–87).
Supplement_Material.docx
Download MS Word (631.9 KB)Acknowledgements
We would also like to acknowledge the support of Iran Nanotechnology Initiative Council (INIC), Iran.
Disclosure statement
No potential conflict of interest was reported by the authors.
Notes on contributors
Mohammad Eslami was born in Parsabad, Iran, 1985. He studied Applied Chemistry at Mohaghegh Ardabili University, Ardabili, Iran, and received his B.Sc in February, 2010. In September 2010, he started his studies at M.Sc level of Organic Chemistry under the supervision of Dr. Mohammad G. Dekamin in Iran University of Science and Technology (IUST), Tehran, Iran and, was graduated in November 2012. In September 2013, he joined the research group of Prof. Matloubi Moghaddam, as a Ph.D candidate, at Sharif University of Technology (SUT), Tehran, Iran. His research interests are green chemistry, nano-ordered catalysis and multicomponent reactions.
Dr. Mohammad G. Dekamin was born in Nahavand, Iran, 1972. He studied Pure Chemistry at Shahid Chamran University (SCU), Ahvaz, Iran and received his B.Sc Degree in July, 1995. Dr. Dekamin obtained his M.Sc Degree in Organic Chemistry under the supervision of Professor Mohammad S. Khajavi at Shahid Beheshti University (SBU), Tehran, Iran, in 1997, and completed his Ph.D. Degree in the same subject under the supervision of Professor Firouz Matloubi Moghaddam at Sharif University of Technology (SUT) Tehran, Iran, in 2002. During the Ph.D. program (2001), Dr. Dekamin spent 8 months in the research group of Professor James H. Clark, Clean Technology Center at The University of York, UK as a visiting scholar. He joined the faculty of the Department of Chemistry at Isfahan University of Technology (IUT) in July 2003. In October 2004, he moved to Iran University of Science and Technology (IUST), Tehran, Iran, where he is currently Associate Professor of Organic Chemistry (Since May, 2011). Dr. Dekamin also took a sabbatical leave for 2 months in the research group of Professor Takeshi Endo (Emeritus Professor at Tokyo Institute of Technology) at the Molecular Engineering Institute (MEI), Kinki University, Iizuka, Fukuoka, Japan from July 2012 to September 2012. Now, 8 Ph.D students and 14 MSc. Students are working under his supervision. He has published more than 65 peer-reviewed papers in the field of organic chemistry. His research interests are green chemistry, heterogeneous catalysis, nanomaterials, natural and synthetic organocatalysts, and medicinal and supramolecular chemistry.
Leila Motlagh was born in Sari, Iran, 1986. He studied Pure Chemistry at Payame Noor University (PNU), Sari, Iran and received her B.Sc in July, 2009. In October 2010, she started her studies at M.Sc level of organic Chemistry under supervision of Dr. Mohammad G. Dekamin in Iran University of Science and Technology (IUST), Tehran, Iran and was graduated in October, 2012. Her research interests are green chemistry, nano-ordered catalysis, nano-absorbents and multicomponent reactions.
Dr. Ali Maleki received his Ph.D. in Organic Chemistry at Shahid Beheshti University in 2009. He started his career as an Assistant Professor in Iran University of Science and Technology, Iran, in 2010, where he is currently Associate Professor. His research interests focus on design and development of novel catalysts, nanochemistry and green chemistry. He has published more than 100 peer-reviewed papers. Dr. Maleki was recently awarded the 2016 IUPAC-CHEMRAWN VII prize for green chemistry.
ORCID
Mohammad G. Dekamin http://orcid.org/0000-0002-7018-7363
Ali Maleki http://orcid.org/0000-0001-5490-3350
Additional information
Funding
References
- Abell, J.P.; Yamamoto, H. J. Am. Chem. Soc. 2009, 131, 15118–15119. doi: 10.1021/ja907268g
- Shafran, Y.M.; Bakulev, V.S.; Mokrushin, V.S. Russ. Chem. Rev. 1989, 58, 148–162. doi: 10.1070/RC1989v058n02ABEH003432
- Smith, M.B.; March, J. March’s Advanced Organic Chemistry: Reactions, Mechanisms, and Structure, 5th ed.; Wiley-Interscience: New York, 2001.
- De, S.K.; Gibbs, R.A. Tetrahedron Lett. 2004, 45, 7407–7408. doi: 10.1016/j.tetlet.2004.08.071
- Weinstock, L.M.; Davis, P.; Handelsman, B.; Tull, R.J. J. Org. Chem. 1967, 32, 2823–2829. doi: 10.1021/jo01284a040
- Matier, W.L.; Owens, D.A.; Comer, W.T.; Deitchman, D.; Ferguson, H.C.; Seidehamel, R.J.; Young, J.R. J. Med. Chem. 1973, 16, 901–908. doi: 10.1021/jm00266a008
- Sigman, M.S.; Vachal, P.; Jacobsen, E.N. Angew. Chem. Int. Ed. 2000, 39, 1279–1281. doi: 10.1002/(SICI)1521-3773(20000403)39:7<1279::AID-ANIE1279>3.0.CO;2-U
- Dyker, G. Angew. Chem. Int. Ed. 1997, 36, 1700–1702. doi: 10.1002/anie.199717001
- Duthaler, R.O. Tetrahedron 1994, 50, 1539–1650. doi: 10.1016/S0040-4020(01)80840-1
- Strecker, A. Ann. Chem. Pharm. 1850, 75, 27–45. doi: 10.1002/jlac.18500750103
- Zhu, J.; Bienayme, H. Multicomponent Reactions, Wiley-VCH: Weinheim, 2005.
- Ganem, B. Accounts Chem. Res. 2009, 42, 463–472. doi: 10.1021/ar800214s
- Mikami, Y.; Takahashi, K.; Yazawa, K.; Arai, T.; Namikoshi, M.; Iwasaki, S.; Okuda, S. J. Biol. Chem. 1985, 260, 344–348.
- Martinez, E.J.; Corey, E. J. Org. Lett. 1999, 1, 75–78. doi: 10.1021/ol990553i
- Myers, A.G.; Kung, D.W. Org. Lett. 2000, 2, 3019–3022. doi: 10.1021/ol0063398
- Rinehart, K.L.; Holt, T.G.; Fregeau, N.L.; Keifer, P.A.; Wilson, G.R.; Perun, T.J.; Sakai, R.; Thompson, A.G.; Stroh, J.G.; Shield, L.S.; Seigler, D.S.; Li, L.H.; Martin, D.G.; Grimmelikhuijzen, C.J.P.; Gäde, G. J. Nat. Prod. 1990, 53, 771–792. doi: 10.1021/np50070a001
- Rinehart, K.L.; Holt, T.G.; Fregeau, N.L.; Stroh, J.G.; Keifer, P.A.; Sun, F.; Li, L.H.; Martin, D.G. J. Org. Chem. 1990, 55, 4512–4515. doi: 10.1021/jo00302a007
- Corey, E.J.; Gin, D.Y.; Kania, R.S. J. Am. Chem. Soc. 1996, 118, 9202–9203. doi: 10.1021/ja962480t
- Mobaraki, A.; Movassagh, B.; Karimi, B. ACS Comb. Sci. 2014, 16, 352–358. doi: 10.1021/co500022g
- Razafindrabe, C.R.; Aubry, S.; Bourdon, B.; Andriantsiferana, M.; Pellet-Rostaing, S.; Lemaire, M. Tetrahedron 2010, 66, 9061–9066. doi: 10.1016/j.tet.2010.08.053
- Meijden, M.W.; Leeman, M.; Gelens, E.; Noorduin, W.L.; Meekes, H.; Enckevort, W.J.P.; Kaptein, B.; Vlieg, E.; Kellogg, R.M. Org. Process Res. Dev. 2009, 13, 1195–1198. doi: 10.1021/op900243c
- Noorduin, W.L.; Asdonk, P.; Bode, A.A.C.; Meekes, H.; Enckevort, W.J.P.; Vlieg, E.; Kaptein, B.; Meijden, M.W.; Kellogg, R.M.; Deroover, G. Org. Process Res. Dev. 2010, 14, 908–911. doi: 10.1021/op1001116
- Chirumamilla, R.R.; Marchant, R.; Nigam, P. J. Chem. Technol. Biot. 2001, 76, 123–127. doi: 10.1002/jctb.337
- Nam, D.H.; Lee, C.S.; Ryu, D.D.Y. J. Pharm. Sci. 1984, 73, 1843–1844. doi: 10.1002/jps.2600731251
- Enders, D.; Shilvock, J.P. Chem. Soc. Rev. 2000, 29, 359–373. doi: 10.1039/a908290e
- Prasad, B.A.B.; Bisai, A.; Singh, V.K. Tetrahedron Lett. 2004, 45, 9565–9567. doi: 10.1016/j.tetlet.2004.11.015
- Zuend, S.J.; Coughlin, M.P.; Lalonde, M.P.; Jacobsen, E.N. Nature 2009, 461, 968–470. doi: 10.1038/nature08484
- Nakamura, S.; Sato, N.; Sugimoto, M.; Toru, T. Tetrahedron: Asym. 2004, 15, 1513–1516. doi: 10.1016/j.tetasy.2004.03.040
- Sipos, S.; Jablonkai, I. Tetrahedron Lett. 2009, 50, 1844–1846. doi: 10.1016/j.tetlet.2009.02.004
- Harusawa, S.; Hamada, Y.; Shioiri, T. Tetrahedron Lett. 1979, 20, 4663–4666. doi: 10.1016/S0040-4039(01)86677-6
- Zheng, L.; Yuanhong, M.; Jun, X.; Jinghong, S.; Hongfang, C. Tetrahedron Lett. 2010, 51, 3922–3926. doi: 10.1016/j.tetlet.2010.05.088
- Karmakar, B.; Banerji, J. Tetrahedron Lett. 2010, 51, 2748–2750. doi: 10.1016/j.tetlet.2010.03.059
- De, S.K. J. Mol. Catal. A: Chem. 2005, 225, 169–171. doi: 10.1016/j.molcata.2004.09.005
- Majhi, A.; Kim, S.S.; Kadam, S.T. Tetrahedron 2008, 64, 5509–5514. doi: 10.1016/j.tet.2008.03.106
- Shen, Z.L.; Ji, S.J.; Loh, T.P. Tetrahedron Lett. 2008, 64, 8159–8163. doi: 10.1016/j.tet.2008.06.047
- Majhi, A.; Kim, S.S.; Kim, H.S. Appl. Organomet. Chem. 2008, 22, 466–470. doi: 10.1002/aoc.1427
- Santosh, P.T.; Kadam, T.; Kim, S.S. Tetrahedron Lett. 2010, 66, 1684–1688. doi: 10.1016/j.tet.2010.01.010
- Mojtahedi, M.M.; Abaee, S.M.; Alishiri, T. Tetrahedron Lett. 2009, 50, 2322–2325. doi: 10.1016/j.tetlet.2009.02.199
- Ghafuri, H.; Rashidizadeh, A.; Ghorbani, B.; Talebi, M. New J. Chem. 2015, 39, 4821–4829. doi: 10.1039/C5NJ00314H
- Khan, N.H.; Agrawal, S.; Kureshy, R.I.; Abdi, S.H.R.; Singh, S.; Suresh, E.; Jasra, R.V. Tetrahedron Lett. 2008, 49, 640–644. doi: 10.1016/j.tetlet.2007.11.136
- Paraskar, A.S.; Sudalai, A. Tetrahedron Lett. 2006, 47, 5759–5762. doi: 10.1016/j.tetlet.2006.06.008
- Karimi, B.; Maleki, A.; Elhamifar, D.; Clark, J.H.; Hunt, A. J. Chem. Commun. 2010, 46, 6947–6949. doi: 10.1039/c0cc01426e
- Karmakar, B.; Sinhamahapatra, A.; Panda, A.B.; Banerji, J.; Chowdhury, B. Appl. Catal.: A 2010, 392, 111–117. doi: 10.1016/j.apcata.2010.10.030
- Prakash, G.K.S.; Mathew, T.; Panja, C.; Alconcel, S.; Vaghoo, H.; Do, C.; Olah, G.A. Proc. Natl. Acad. Sci. USA 2007, 104, 3703–3706. doi: 10.1073/pnas.0611316104
- Kumar, S.D.; Saini, A.; Sandhu, J.S. Russ. J. Chem. 2008, 1, 684–688.
- Pasha, M.A.; Nanjundaswamy, H.M.; Jayashankara, V.P. Synth. Commun. 2007, 37, 4371–4380. doi: 10.1080/00397910701578180
- De, S.K.; Gibbs, R.A. Synth. Commun. 2005, 35, 961–966. doi: 10.1081/SCC-200051702
- De, S.K. Synth. Commun. 2005, 35, 653–656. doi: 10.1081/SCC-200050347
- Narasimhulu, M.; Reddy, T.S.K.; Mahesh, C.; Reddy, S.M.; Reddy, A.V.; Venkateswarlu, Y. J. Mol. Catal. A: Chem. 2007, 264, 288–292. doi: 10.1016/j.molcata.2006.09.036
- Royer, L.; De, S.K.; Gibbs, R.A. Tetrahedron Lett. 2005, 46, 4595–4597. doi: 10.1016/j.tetlet.2005.05.005
- Heravi, M.M.; Ebrahimzadeh, M.; Oskooie, H.A.; Baghernejad, B. Chin. J. Chem. 2010, 28, 480–482. doi: 10.1002/cjoc.201090100
- Heydari, A.; Khaksar, S.; Pourayoubi, M.; Mahjoub, A.R. Tetrahedron Lett. 2007, 48, 4059–4060. doi: 10.1016/j.tetlet.2007.04.018
- Ghafuri, H.; Roshani, M. RSC Adv. 2014, 4, 58280–58286. doi: 10.1039/C4RA11957F
- Reddy, C.S.; Raghu, M. Indian J. Chem. Sect. B 2008, 47, 1572–1577.
- Baghbanian, S.M.; Farhang, M.; Baharfar, R. Chin. Chem. Lett. 2011, 22, 555–558. doi: 10.1016/j.cclet.2010.12.003
- Wang, J.C.; Masui, Y.; Onaka, M. Eur. J. Org. Chem. 2010, 2010, 1763–1771. doi: 10.1002/ejoc.200901323
- Barbero, M.; Cadamuro, S.; Dughera, S.; Ghigo, G. Org. Biomol. Chem. 2012, 10, 4058–4068. doi: 10.1039/c2ob25584g
- Shekouhy, M. Catal. Sci. Technol. 2012, 2, 1010–1020. doi: 10.1039/c2cy00493c
- Shaabani, A.; Maleki, A.; Soudi, M.R.; Mofakham, H. Catal. Commun. 2009, 10, 945–949. doi: 10.1016/j.catcom.2008.12.035
- Shaabani, A.; Maleki, A. Appl. Catal.: A 2007, 331, 149–151. doi: 10.1016/j.apcata.2007.07.021
- Prakash, G.K.S.; Thomas, T.E.; Bychinskaya, I.; Prakash, A.G.; Panja, C.; Vaghoo, H.; Olah, G.A. Green Chem. 2008, 10, 1105–1110. doi: 10.1039/b803152e
- Dekamin, M.G.; Azimoshan M.; Ramezani, L. Green Chem. 2013, 15, 811–820. doi: 10.1039/c3gc36901c
- Rajabi, F.; Nourian, S.; Ghiassian, S.; Balu, A.M.; Saidi, M.R.; Serrano-Ruiz, J.C.; Luque, R. Green Chem. 2011, 13, 3282–3289. doi: 10.1039/c1gc15741h
- Rajabi, F.; Ghiassianb, S.; Saidi, M.R. Green Chem. 2010, 12, 1349–1352. doi: 10.1039/c0gc00047g
- Derikvand, Z.; Derikvand, F. Chin. J. Catal. 2011, 32, 532–535. doi: 10.1016/S1872-2067(10)60189-1
- Dekamin, M.G.; Karimi, Z. Sci. Iran 2011, 18, 1356–1364. doi: 10.1016/j.scient.2011.11.005
- Karimi, B.; Zareyee, D. J. Mater. Chem. 2009, 19, 8665–8670. doi: 10.1039/b911388f
- Dekamin, M.G.; Mokhtari, Z. Tetrahedron 2012, 68, 922–930. doi: 10.1016/j.tet.2011.10.087
- Paul, T.A.; Tracy, C.W. J. Am. Chem. Soc. 1996, 626, 1–17.
- Anastas, P.T.; Kirchhoff, M.M. Account. Chem. Res. 2002, 35, 686–694. doi: 10.1021/ar010065m
- Hoffmann, F.; Cornelius, M.; Morell, J.; Fröba, M. Angew. Chem. Int. Ed. 2006, 45, 3216–3251. doi: 10.1002/anie.200503075
- Iwanami, K.; Seo, H.; Choi, J.C.; Sakakura, T.; Yasuda, H. Tetrahedron 2010, 66, 1898–1901. doi: 10.1016/j.tet.2010.01.001
- Karimi, B.; Khalkhali, M. J. Mol. Catal. A: Chem. 2007, 271, 75–79. doi: 10.1016/j.molcata.2007.02.018
- Yaghoubi, A.; Dekamin, M.G. Green and Facile Synthesis of 4H-Pyran Scaffold Catalyzed by Pure Nano-Ordered Periodic Mesoporous Organosilica with Isocyanurate Framework (PMO-ICS). ChemistrySelect. 2017, 2, 9236–9243. doi: 10.1002/slct.201700717
- Dekamin, M.G.; Arefi, E.; Yaghoubi, A. Isocyanurate-based periodic mesoporous organosilica (PMO-ICS): a highly efficient and recoverable nanocatalyst for the one-pot synthesis of substituted imidazoles and benzimidazoles. RSC Adv. 2016, 6, 86982–86988. doi: 10.1039/C6RA14550G
- Lei, Q.; Wei, Y.; Talwar, D.; Wang, C.; Xue, D.; Xiao, J. Chem. Eur J. 2013, 19, 4021–4029. doi: 10.1002/chem.201204194
- Olah, G.A.; Mathew, T.; Panja, C.; Smith, K.; Prakash, G.S. Catal. Lett. 2007, 114, 1–7. doi: 10.1007/s10562-007-9031-y
- El-Ahl, A.A.S. Synth. Commun. 2003, 33, 989–998. doi: 10.1081/SCC-120016363
- Dekamin, M.G.; Karimi, Z.; Farahmand, M. Tetraethylammonium 2-(N-hydroxycarbamoyl)benzoate: a powerful bifunctional metal-free catalyst for efficient and rapid cyanosilylation of carbonyl compounds under mild conditions. Catalysis Science & Technology. 2012, 2, 1375–1381. doi: 10.1039/c2cy20037f
- Dekamin, M.G.; Yazdaninia, N.; Mokhtari, J.; Naimi-Jamal, M.R. Tetrabutylammonium phthalimide-N-oxyl: An efficient organocatalyst for trimethylsilylation of alcohols and phenols with hexamethyldisilazane. J. Iran. Chem. Soc. 2011, 8, 537–544. doi: 10.1007/BF03249088
- Iovel, I.; Golomba, L.; Fleisher, M.; Popelis, J.; Grinberga, S.; Lukevics, E. Chem. Heterocycl. Comp. 2004, 40, 701–714. doi: 10.1023/B:COHC.0000040765.61681.f0
- Al-Kahraman, Y.M.S.A.; Madkour, H.M.F.; Ali, D.; Yasinzai, M. Molecules 2010, 15, 660–671. doi: 10.3390/molecules15020660
- Tan, D.W.; Li, H.X.; Young, D.J.; Lang, J.P. Tetrahedron 2016, 72, 4169–4176. doi: 10.1016/j.tet.2016.05.036
- Imhof, W. J. Organomet. Chem. 1997, 533, 31–43. doi: 10.1016/S0022-328X(96)06824-6
- Xu, W.; Zhang, S.; Yang, S.; Jin, L.H.; Bhadury, P.S.; Hu, D.Y.; Zhang, Y. Molecules 2010, 15, 5782–5796. doi: 10.3390/molecules15085782
- Yasser, M.S.A.; Girija, S.S.; Masoom, Y. Lett. Drug Des. Dis. 2011, 8, 491–495. doi: 10.2174/157018011795514221
- Wang, R.; Xu, J. Arkivoc 2010, 9, 293–299.