ABSTRACT
Green chemistry can strongly attract students to chemistry. We, therefore, developed a green chemistry educational game that motivates students at the undergraduate and advanced high school levels to consider green chemistry and sustainability concerns as they design a hypothetical, chemical product. The game is intended for incorporation into any chemistry course for majors and non-majors that teaches sustainability and/or the Principles of Green Chemistry at the undergraduate level. The game is free of charge and encourages students to think like professional chemical designers and to develop a chemical product with respect to function and improved human and environmental health. This computer simulation has been assessed by educators and can be seamlessly integrated into an existing curriculum.
GRAPHICAL ABSTRACT
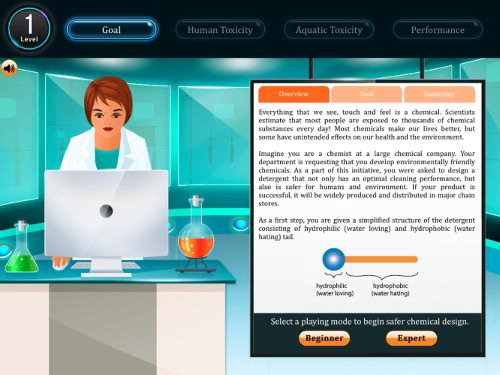
Introduction
Green chemistry can attract students to graduate science and engineering programs, and can provide valuable skills for collaboration with other disciplines ( Citation1,Citation2). The Molecular Design Research Network (MoDRN) is the multi-institutional green chemistry collaboration that was developed to create guidelines for chemists to design safer chemicals in terms of both human and environmental health. MoDRN research resulting from this four-year partnership was used to develop a myriad of educational materials for students, teachers, and professionals to advance green chemistry and the adoption of safer chemical guidelines by practitioners in the field. The educational materials of MoDRN are designed to provide interactive environments where students can develop skills such as critical thinking and problem-solving, or engage in peer-to-peer learning ( Citation3).
In recent years, there has been an increasing interest in using computer simulations with game mechanics to improve pedagogical effectiveness and reinforce information acquisition ( Citation4, Citation5). Since educational simulations can be built to facilitate exploration of different scenarios and guide students through decision-making processes, they present an opportunity to enhance conventional student curriculum. For this reason, we set out to create an educational game for undergraduate and advanced high school students that would not only educate students in safer chemical design, but further their interest in green chemistry. Our goal was to develop an innovative and free of charge, on-line “Safer Chemical Design” simulation that can be seamlessly integrated into an existing curriculum. We partnered with Aptara, a media company specializing in digital content development, in order to construct this unique game. The approach Aptara used was to gamify a digital learning object (DLO) based on safer chemical design that was grounded in experiential learning cycles and styles ( Citation6–8). The principles of experiential learning that were incorporated into the game by Aptara included (1) the reiteration of familiar chemistry concepts by the learner, (2) the presentation of introductory content, examples, or scenarios that mimic real-life instances, (3) the provision for tips, hints and learning aids and (4) the provision to apply and test concepts in a simulated, controlled scenario. Aptara also integrated the important elements of gamification into the overall DLO design, such as scores and rewards to simulate game mechanics. In this letter, we provide an overview of the game and our approach to its creation.
Gameplay
The game was designed to introduce students to safer chemical design concepts and focused on the manipulation of the physico-chemical parameters in order to minimize undesired biological and environmental interactions of a hypothetical commercial chemical. Game scenarios that are presented to the student (player) model the decision-making process used by professionals to design a new chemical and thus the graphic style of the game is realistic. Critically, the computer game simulates various real-world constraints that may affect chemical product development as the student designs a novel product. The students (players) are challenged to design a safer, and more sustainable chemical product using multi-criteria decision analysis. Players can select different combinations of molecular parameters that lead to qualitative outputs related to toxicity, biodegradability, biotransformation, and overall performance (). In doing so, the player must navigate potential tradeoffs as a consequence of their choices. Two levels of complexity are available. The player may choose from beginner and expert levels, which increase in complexity as the player progresses through the game. Each level is divided into three challenges, or tasks, that are related to the attributes of the chemical product, including potential for human toxicity, aquatic toxicity, and performance (function) (). As students progress through different challenges, they are offered “Tips” which include additional information to aid their parameter choices. Both levels require the lower order learning objective of fact memorization as well as the higher order learning objective of knowledge transfer as the student optimizes the chemical product against multiple product requirements across tasks. The design of the chemical product is evaluated on function and avoidance of toxicity. A key feature of the game is the ability of the player to redesign and improve their product based on real-time feedback (). Feedback is included after every task which allows the student to change their selection criteria and improve the design.
Figure 1. Game screenshot: Optimization of physico-chemical properties of the hypothetical chemical product.
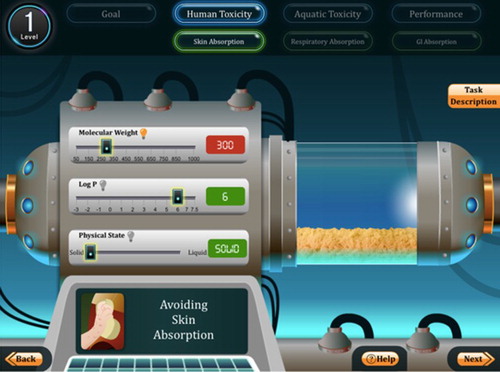
Figure 2. Game structure: The game has two levels and each level is divided into three optimization challenges that are related to human toxicity, aquatic toxicity, and performance. Each challenge is subdivided into tasks related to specific topics such as skin absorption and metabolism.
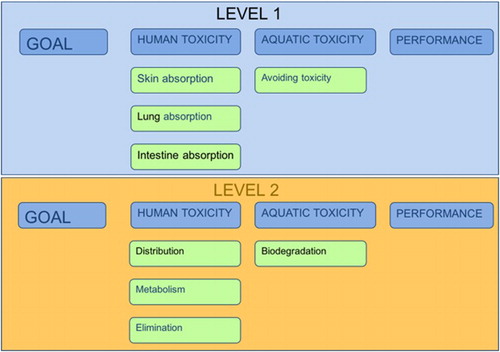
Figure 3. Game screenshot: An example of real-time feedback that allows the player to redesign the product.
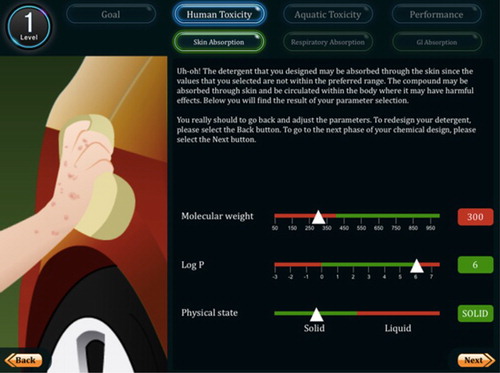
The game concepts are based on the 12 Principles of Green Chemistry ( Citation9) and introduce toxicology and pharmacokinetic concepts such as absorption, distribution, metabolism, and excretion. While the simulation is based on scientific principles and will educate students in real-life challenges, it is also intended to entertain and engage students using game mechanics. The player is rewarded for successfully designing a safer chemical based on information, data and feedback within the game that exhibits principles of gamification, namely goal orientation, achievement (i.e. reward points), reinforcement (looping based on feedback) and fun orientation. Based on this gameplay design, we hoped to achieve the objectives in .
Figure 4. Game Objectives using Bloom’s Taxonomy ( Citation10).
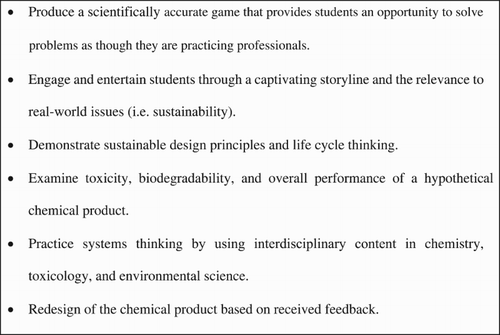
Integration into undergraduate curriculum
Significant advances in integrative, educational programs and novel teaching tools have been made within the past decade. Teachers have integrated toxicology and green chemistry within existing curricula in high school, undergraduate and graduate levels ( Citation11,Citation12). There is a growing demand for related content and tools as more teachers seek ways to integrate toxicology and green chemistry concepts into their courses and programs. We envision that the Safer Chemical Design Game will provide teachers with a tool to integrate toxicology and green chemistry concepts into any chemistry course for majors and non-majors that teaches sustainability and/or the Principles of Green Chemistry at the undergraduate level in a fun and meaningful way.
The game can be integrated in existing curricula in the following ways:
As an in-class exercise, followed by a discussion about the importance of physico-chemical properties;
As a take-home problem followed by an assignment to discuss the advantages and disadvantages of safer chemical design that were explored; and
As additional resource while discussing the chemistry/toxicology nexus.
In-class exercise
Prior to students playing Level 1 of the game, it is recommended that the instructor introduce physico-chemical parameters such as log P and molecular weight, as well as the concepts of dermal, respiratory, and intestinal absorption. Physico-chemical parameters and their influences on absorption are described in ToxTutor ( Citation13) and by MoDRN ( Citation14), and in the Tips embedded within the game. After a discussion of these concepts, students should develop a hypothesis on how these physico-chemical parameters may affect the absorption of a chemical via the three routes of exposure (dermal, respiratory, and intestinal). After completion of Level 1 of the Safer Chemical Design Game, students should reflect on their earlier assumptions and discuss whether their predictions were correct. The discussion can be also framed around finding an optimal chemical design, where the chemical product has a reduced toxicity and increased performance. A similar approach can be used for game Level 2.
Take-home challenge
The Safer Chemical Design Game can be used as a homework assignment, where students are not restricted by the class period and can freely explore different product design scenarios. Therefore, we recommend that the instructor prepare a number of questions which are related to physico-chemical properties and their impact on the function of the chemical. As students play the game, they should record their findings and then provide an explanation of their reasoning. This take-home challenge should test student knowledge retention and understanding of the safer chemical design principles which is based on game content. Examples of questions for the instructor are included in the supplementary material.
Additional resource
Because many institutions have already developed lesson plans around toxicology and green chemistry concepts, the Safer Chemical Design Game may act as an additional resource while discussing topics related to chemistry and toxicology. It can accompany discussions of absorption, distribution, metabolism, and elimination (ADME), molecular transport, physico-chemical properties of chemicals, toxicity, or green chemistry.
Technical aspects
We worked with Aptara, who developed a DLO that aims to provide a gamified learning experience through a device-agnostic course player. The course player was developed using an HTML5 + CSS3 + JavaScript + JSON technology stack, while taking into consideration that the application will be used across varying target devices (desktops, laptops and tablets). The scenarios in the DLO have been in a controlled functional architecture which are based on pre-determined interaction outcomes. The functional architecture of the DLO was developed using an object-oriented JavaScript framework, in a modular architecture based on a Model-View-Presenter design pattern, allowing subsequent versions of the DLO to be easily extended in terms of features, functionalities and overall content. Other frameworks used include EaselJS/CreateJS for building custom data visualization and sprite-sheet based animations, with audio implemented using native HTML5 capabilities.
Game assessment
The game was assessed by MoDRN faculty and staff during the workshop “Toxicology for Chemists” held at the 2017 Green Chemistry & Engineering conference in Reston, VA. Seventeen chemistry educators, government scientists, and chemical industry leaders attended the workshop. Participants were given (1) a 15-minute instruction on the science behind the simulation, learning objectives, and game mechanics; (2) 30 minutes to play the game and discuss it with other workshop attendees; (3) 10 minutes to complete an evaluation survey that included feedback on content, ease of use, and graphics (appearance). The majority (87%) of the workshop participants ranked the game idea and the overall game experience as highly favorable and engaging, and thought that the game instructions were easy to follow. The graphics and illustrations were also rated as being very highly attractive (87%). 100% of participants also agreed that the game content was useful and 62.5% said it contributed to a multidisciplinary learning experience. Critically, most (75%) indicated that they are very likely to include the game in their existing curriculum. We also beta tested the game among various members of MoDRN and Yale Center for Green Chemistry & Green Engineering, including postdoctoral fellows, graduate and undergraduate students, and faculty with backgrounds in Green Chemistry. Their feedback was incorporated into the final version.
Game construction – challenges and hurdles
With our goal of creating an educational game in mind, we set out to construct our online game with a focus on safer chemical design. The MoDRN team, along with the Center for Green Chemistry & Green Engineering, contributed expertise in safer chemical design; however, we did not have expertise in the gamification of a DLO. Our first approach was to create an ad hoc team of various experts from academic institutions, including experts in areas that we thought were applicable to game design. In practice, this was a challenging task, primarily because of the many experts with different skill sets that are needed to construct an online game. We next turned our focus on outsourcing to a company and brought Aptara, a professional game designing team, to the project. With their assistance, we were able to construct the game at a moderate pace, and we had results in approximately 15 months. Given our limited resources, we pursued a 2-D game with limited animations. The project team also included Information Technology Services (ITS) specialists from Yale that assisted with technical aspects such as transfer of game files to a Yale secure server intended for sharing with the public.
Conclusions and next steps
We successfully created an educational game for undergraduate and advanced high school students that is intended to translate research to practice in the field of safer chemical design. Our game is intended to attract and retain students, including women and underrepresented minorities, to the fields of STEM to pursue careers in STEM fields ( Citation15). Gameplay should help students make more connections between the scientific topics of study and the global, sustainability concerns that they observe in daily life. The game was assessed by chemistry educators and students. The majority of the workshop participants ranked the overall game experience as highly favorable and engaging, and agreed that the game content was informative. Critically, educators indicated that they are very likely to include the game in their existing curriculum.
We will next implement the learning tool in various classroom settings, and make the game available online to the broader educational community. At a future stage, the game may be expanded to explore other aspects of a chemical life cycle such as its manufacturing route, number of production steps, energy usage, etc. In this way, the game can further simulate the way that real-world constraints may affect product development and place a focus on life-cycle-analysis. Modification may also include a chemical editor so that students can construct discrete molecules that can be evaluated in real-time in commercial or proprietary software for various parameters such eco-toxicity. This addition would add considerable complexity to the technical aspects of game construction. The Safer Chemical Design Game can be accessed by http://greenchemistry.yale.edu/education/undergraduate-gra-duate → Safer Chemical Design Game.
Acknowledgements
This work could have not been completed without the help of the following entities: We would like to specially thank Aptara for gamifying the scientific content; Yale ITS for game deployment; Yale Center for Teaching and Learning for the educational input; Yale Center for Green Chemistry and Green Engineering postdoctoral, doctoral and undergraduate students for technical expertise. We would also like to thank reviewers of the beta version: Molecular Design Research Network, Beyond Benign and the green chemistry community.
Supplement_Material.docx
Download MS Word (1 MB)Disclosure statement
No potential conflict of interest was reported by the authors.
Notes on contributors
Dr Karolina E. Mellor manages research and education projects at the Center for Green Chemistry and Green Engineering at Yale on a local, national and international scale. She also actively promotes the Center's activity by leading educational efforts and translating research into teaching tools to educate diverse audiences in green chemistry and sustainability. Dr Mellor is an expert in online/traditional teaching, instructional design and workshop development. Prior to her role as a green chemistry education expert, Karolina received her Ph.D. at the University of Virginia, where she investigated resistance of feedstocks to various biotic and abiotic stresses.
Dr Philip Coish has extensive experience as a project manager in multiple areas of drug discovery and scientific research. He received a Ph.D. in synthetic organic chemistry from the University of British Columbia and was a post-doctoral fellow at the University of Pittsburgh. He started his professional career at Bayer Healthcare as a drug researcher and was involved in project leadership and portfolio management. He then worked at Sepracor (now Sunovion) as an Associate Director where he was a portfolio manager and contributed to the growth and development of the early discovery research portfolio. He is currently a program manager within the Center for Green Chemistry and Green Engineering at Yale.
Dr Bryan W. Brooks is a Distinguished Professor in the Department of Environmental Science and Institute of Biomedical Studies, and Director of Environmental Health Sciences at Baylor University, Waco, Texas. His research focuses on sustainable molecular design, environmental toxicology and chemistry, water resources and urbanization, environmental public health, and harmful algal blooms. Dr Brooks serves as Editor-in-Chief of Environmental Management (Springer Nature).
Dr Evan P. Gallagher is a Professor of Environmental Toxicology in the Department of Environmental and Occupational Health Sciences at the University of Washington, and a holder of the Sheldon D. Murphy Endowed Chair in Toxicology. Dr Gallagher also serves as the Director of the UW NIH Superfund Research Program. He received his B.S. in biology from Virginia Tech, and Ph.D. in biochemical toxicology from Duke University, and completed an NIEHS-funded postdoctoral fellowship at the University of Washington in environmental carcinogenesis. He has published 85 peer-reviewed manuscripts and has presented over 150 scientific research presentations.
Dr Margaret Mills is a postdoctoral researcher in aquatic toxicology, working in the Gallagher laboratory at the University of Washington. Her research focuses on the genetics of antioxidant response in zebrafish (Danio rerio). She completed her Ph.D. in the lab of Dr Catherine Peichel at Fred Hutchinson Cancer Research Center, studying the pleiotropic effects of the Eda gene in threespine stickleback (Gasterosteus aculeatus), and the role of this gene in the evolution and development of different populations of sticklebacks.
Dr Terrance J. Kavanagh received a B.S. in Natural Resources from the University of Michigan, an M.S. in physiology/toxicology, and a Ph.D. in Toxicology and Genetics from Michigan State University. He conducted research in free radical biology and aging as a post-doctoral fellow at the University of Washington. Dr Kavanagh joined the faculty at the University of Washington in the Departments of Medicine and Environmental Health in 1989. He is currently Professor in the Department of Environmental and Occupational Health Sciences and Adjunct Professor of Pulmonary and Critical Care Medicine. Dr Kavanagh is a Diplomate of the American Board of Toxicology and a member of the Society of Toxicology (Vice President Elect of the Mechanisms Specialty Section).
Nancy Simcox, M.S., is the Director of the Continuing Education Programs at the University of Washington, Department of Environmental and Occupational Health Sciences (UW DEOHS) where she develops and delivers research-based education programs for practicing professionals including industrial hygienists, physicians, nurses, safety engineers, and others in the environmental, health and safety field. Ms. Simcox has over 20 years of experience as a research industrial hygienist, where she conducted workplace site visits, designed exposure assessment protocols for evaluation, intervention and research, and performed data reduction and statistical analysis for health-based research projects.
Dr Grace A. Lasker's research focus is in the areas of environmental health and justice, epigenetics, and nutrition. Her research centers on the impact of pesticide bioaccumulation (through environmental and food exposure) on human health. She is also investigating the intersection of toxicology, green chemistry, and educational outreach. Dr Lasker has focused her career on teaching and program development, particularly online programs. She is a certified online developer and instructional designer, working in this capacity for programs and grants across the country.
Dianne Botta is a member of the staff at the Department of Environmental and Occupational Health Sciences, University of Washington, Seattle, WA.
Dr Adelina Voutchkova-Kostal is an Assistant Professor in the Chemistry Department at The George Washington University. She completed her graduate work at Yale University under the supervision of Robert Crabtree in organometallic chemistry and catalysis, prior to which she got her Bachelor's degree in Chemistry and Biochemistry from Middlebury College. Her postgraduate work at the Yale Center for Green Chemistry and Green Engineering with Professor Paul Anastas focused on the rational design of functional commercial chemicals that are safe to humans and the environment. In Dr Voutchkova's independent career, she focuses on the two central research frontiers of green chemistry – development of new chemical methodology with reduced environmental impact through catalysis, and the development of tools that chemists can apply to rationally design new chemicals with minimal biological activity.
Dr Jakub Kostal is an Assistant Professor of Chemistry at The George Washington University and co-Founder of DOT Consulting, a company that develops and maintains the ToxFix platform of predictive tools and design guidelines for reduced toxicity of commercial chemicals. Dr Kostal holds B.A. from Middlebury College and Ph.D. in Theoretical and Biophysical Chemistry from Yale University, where he studied under the auspices of Professor Bill Jorgensen. During his postdoctoral research, Dr Kostal worked with Professors Julie Zimmerman and Paul Anastas on developing in silico tools for toxicity testing. Dr Kostal's group at The George Washington University researches the utility of computational chemistry, particularly classical and quantum-mechanics simulations of biochemical systems, in designing safer chemicals.
Melissa L. Mullins, M.S., coordinates education and outreach at the Center for Reservoir and Aquatic Systems Research at Baylor University. Her work involves connecting people of all ages and backgrounds to the center's research and its researchers, and focuses on water education, teacher professional development, increasing girls' involvement in STEM, and Citizen Science. She currently serves on the Board of Directors of the Informal Science Education Association of Texas and is a Certified Environmental Educator.
Dr Suzanne M. Nesmith has been involved in science education for over 30 years at every educational level. She currently serves as an Associate Professor of Science Education at Baylor University. Her educational background in science curriculum and pedagogy alongside her experiences with preservice and in-service science educators have given her a broad base from which to approach many topics. Her specializations, research interests, and numerous publications include topics related to environmental education, inquiry-based science, discipline- and genre-specific literature integration, science efficacy, and STEM for young learners.
Dr Jone Corrales received her Ph.D. in Comparative Biomedical Sciences at North Carolina State University in 2008. She is currently a research scientist in the Department of Environmental Science at Baylor University, Waco, TX.
Dr Lauren Kristofco received her Ph.D. in Environmental Science at Baylor University in 2017. She is currently an environmental toxicologist for Chevron in Houston, TX.
Gavin Saari is a Ph.D. candidate at Baylor University in the Department of Environmental Science. He received his B.S. in biology from the University of Wisconsin-Superior with an emphasis in aquatic ecology and fisheries. His current research focuses on environmental toxicology and physiology, water resources, and urbanization.
W. Baylor Steele is a doctorate candidate in Biomedical Studies at Baylor University. His dissertation research is aimed at leveraging behavioral endpoints in fish bioassays to provide diagnostic screening of aquatic contaminants. He received his M.S. in Environmental Science from the University of North Texas in Denton, TX and his undergraduate degree in Business Administration from the University of Mississippi in Oxford, MS. His recent research has focused on behavioral ecotoxicology, comparative pharmacology, and toxicology, bioconcentration/bioaccumulation of aquatic contaminants, alternative animal models in toxicity testing, and sustainable molecular design.
Fjodor Melnikov is a graduate researcher at the Yale School of Forestry and Environmental Studies working on biological modeling and molecular design guidelines. His research bridges the disciplines of chemistry and toxicology to understand the relationship between chemical properties and the biological pathways the chemicals affect. Fjodor is also developing statistical tools for robust inference from large, high throughput datasets to enable broader data use in modeling and molecular design work. He is an EPA Science to Achieve Results (STAR) fellow. Previously, Fjodor worked as a Toxicologist and a Risk Assessor, completed an M.S. in Environmental Health during a collaboration between Cyprus University of Technology and Harvard School of Public Health, and a B.S. in Biochemisty & Economics at Brandeis University.
Dr Julie B. Zimmerman is a Professor jointly appointed to the Chemical & Environmental Engineering Department of the School of Engineering and Applied Sciences and the School of Forestry and Environmental Studies. She is the Senior Associate Dean of Academic Affairs and Deputy Director at the Yale Center for Green Chemistry & Green Engineering. Her research interests broadly focus on green chemistry and engineering with specific emphasis on green downstream processing and life cycle assessment of algal biomass for fuels and value-added chemicals as well as novel biobased sorbents for purification of drinking water and remediation of industrial wastewater.
Dr Paul T. Anastas holds the Teresa and H. John Heinz III Chair in Chemistry for the Environment at Yale University and is the founding Director of the Center for Green Chemistry & Green Engineering at Yale. Dr Anastas has appointments in the School of Forestry & Environmental Studies, Department of Chemistry, School of Management, School of Public Health and the School of Engineering and Applied Sciences. Dr Anastas has served in three Presidential Administrations including the appointment by President Barack Obama as the Assistant Administrator for Research and Development at the U.S. Environmental Protection Agency. Dr Anastas has published over 150 research papers and 14 books, is known as the “Father of Green Chemistry”, and has been internationally recognized for his advancement of the field.
Additional information
Funding
References
- National Research Council (US) Chemical Sciences Roundtable. In Exploring Opportunities in Green Chemistry and Engineering Education: A Workshop Summary to the Chemical Sciences Roundtable, Anastas P, e. a., Ed; National Academies Press, 2007.
- Haack, J.A.; Hutchison, J.E. Green Chemistry Education: 25 Years of Progress and 25 Years Ahead. Acs. Sustain. Chem. Eng. 2016, 4 (11), 5889–5896. doi: 10.1021/acssuschemeng.6b02069
- NRC. Evaluating and Improving Undergraduate Education in Science, Technology, Engineering, and Mathematics; National Academies Press: Washington, DC, 2003.
- Mayo, M.J. Video Games: A Route to Large-Scale STEM Education? Science. 2009, 323 (5910), 79–82. doi: 10.1126/science.1166900
- Barab, S.A.; Scott, B.; Siyahhan, S.; Goldstone, R.; Ingram-Goble, A.; Zuiker, S.J.; Warren, S. Transformational Play as a Curricular Scaffold: Using Videogames to Support Science Education. J. Sci. Edu. Technol. 2009, 18 (4), 305–320. doi: 10.1007/s10956-009-9171-5
- Shaffer, D.W. Epistemic Frames for Epistemic Games. Comput. Edu. 2006, 46 (3), 223–234. doi: 10.1016/j.compedu.2005.11.003
- Shaffer, D.W. Pedagogical Praxis: The Professions as Models for Post-Industrial Education. Teachers College Record. 2004, 106 (7), 1401–1421. doi: 10.1111/j.1467-9620.2004.00383.x
- APTARA: Instructional Design. http://www.instructionaldesign.org/theories/constructivist.html (accessed Oct 12, 2017).
- Anastas, P.T.; Warner, J.C. Green Chemistry: Theory and Practice; Oxford University Press: New York, 1998.
- Bloom, B.S.; Engelhart, M.D.; Furst, E.J.; Hill, W.H.; Krathwohl, D.R. Taxonomy of Educational Objectives: The Classification of Educational Goals. Handbook I: Cognitive domain; David McKay Company: New York, 1956.
- Purcell, S.C.; Pande, P.; Lin, Y.X.; Rivera, E.J.; Latisha, P.U.; Smallwood, L.M.; Kerstiens, G.A.; Armstrong, L.B.; Robak, M.T.; Baranger, A.M.; Douskey, M.C. Extraction and Antibacterial Properties of Thyme Leaf Extracts: Authentic Practice of Green Chemistry. J. Chem. Educ. 2016, 93 (8), 1422–1427. doi: 10.1021/acs.jchemed.5b00891
- Anastas, P.T.; Levy, I.J.; Parent, K.E. Green Chemistry Education: Changing the Course of Chemistry; American Chemical Society: Washington, 2009.
- ToxTutor. https://toxtutor.nlm.nih.gov/ (accessed Oct 18, 2017).
- Molecular Design Research Network-MoDRN http://modrn.yale.edu/ (accessed Oct 18, 2017).
- Zimmerman, J.B.; Vanegas, J. Using Sustainability Education to Enable the Increase of Diversity in Science, Engineering and Technology-Related Disciplines. Int. J. Eng. Educ. 2007, 23 (2), 242–253.