ABSTRACT
A nano-biocatalyst has been successfully prepared via coordination between zinc ion and the nitrogen atoms of the amide groups in the Candida sp. 99-125 lipase. The as-prepared nanoparticle has an excellent hierarchical structure with a large number of separate petals. In regioselective acylation of arbutin, the nano-biocatalyst showed enhanced enzyme activities to about 4.3-folds than the free enzyme. Meanwhile, the prepared nano-biocatalyst exhibited good operational reusability and only about 6% of enzyme activity has lost during 10 continuous reaction-recycles.
GRAPHICAL ABSTRACT
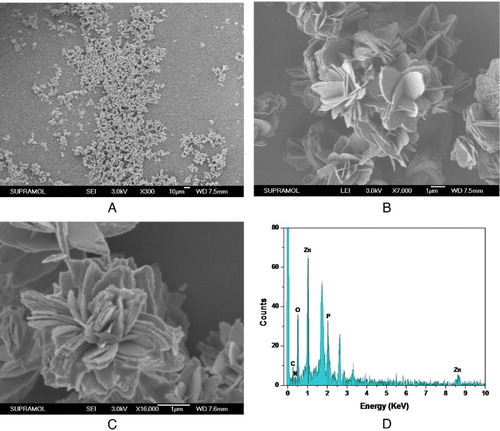
1 Introduction
Enzyme immobilization is a very useful tool in biotechnology ( Citation1). A variety of immobilization techniques (physical adsorption, covalent binding, entrapment and encapsulation, etc.) have been widely applied in the past decades ( Citation2–6). A proper immobilization technique may greatly improve enzyme properties, such as activity, stability, selectivity, specificity and even the purity may be enhanced ( Citation7–13). However, not all immobilization can produce these effects ( Citation14). In 2012, Zare et al. reported a milestone approach for fabricating the organic-inorganic hybrid nanoparticles (hNPs) ( Citation15). They coordinated copper phosphate nanocrystals with the enzymes to form hNPs and found that the hNPs of laccase showed increased enzyme activity for about 6.5-folds. The hNPs of α-chymotrypsin have also been prepared by Lin’s research group ( Citation16) and the enzyme activity was enhanced 266%. They attributed the enhanced enzyme activity to three possible reasons ( Citation8, Citation15–17): (i) synergistic and coupling effects, (ii) allosteric effect and (iii) high surface area of the hNPs. Their inspiring works opened a novel platform for immobilizing enzyme ( Citation18).
Lipase always exhibits high enzyme activity in the organic medium due to its feature of interfacial activation ( Citation19–23). As a widely applied biocatalyst for organic synthesis ( Citation24–28), lipase has also been prepared into hNPs ( Citation15, Citation29,Citation30). However, the existence of Cu2+ might have some negative effects on the conformation of lipase and depress its enzyme activity to a certain extent. To solve this problem, we have prepared a zinc-containing nanoparticle via the coordination between zinc ion and the nitrogen atoms of the amide groups in the Candida sp. 99-125 lipase (CSL). After characterization, the as-prepared nano-biocatalyst by scanning electron micrograph (SEM), FTIR and XRD, the prepared hNPs have been applied for regioselective acylation of arbutin. As a simple natural polyphenol, arbutin has many fascinating bioactivities. Regioselective acetylation can improve its cell membrane penetration and enhance its biological activity. For a kinetically controlled synthesis, the used enzyme and type of enzyme (free enzyme of immobilized enzyme) may alter the enzyme performance and affect the yields ( Citation31). So in this study, regioselective acylation of arbutin was selected as a model reaction to evaluate the effect of immobilization (Scheme 1).
2. Materials and methods
2.1. Materials
Arbutin (98%) was from BC&HC Pharmaceutical Technology Co., Ltd (Changchun, China). All other solvents were purchased from Changchun Chemical Reagent Company (Changchun, China). CSL was from Beijing CTA New Century Biotechnology Co., Ltd. (Beijing, China). KBr was purchased from BDH Co. (Poole, UK). Milli-Q water was used to prepare all the aqueous solutions.
2.2. Fabrication of the hNPs
CSL powder (1 g) was added in phosphate buffer (100 mL, pH 7.4, 4.0 mM) under stirring for 2 h. After centrifugation (8000 rpm, 5 min), the supernatant was lyophilized to obtain the CSL powder. Enzyme solutions (0.6 mg/mL) were prepared by dissolving lyophilized CSL powder in phosphate buffer (4.0 mM, pH 7.4). Under mechanical stirring, zinc sulfate solution (80 mg/mL, 5 mL) was added into the enzyme solution (100 mL). After being stirred for 1 h and incubated for 2 h under static condition, the prepared hNPs were separated by centrifugation and dried at room temperature.
The protein content (C) in hNPs was calculated by the following equation:where C1 is the N element content in hNPs, C2 is the N element content in zinc phosphate, and C3 is the N element content in lipase.
2.3. Characterization of the prepared hNPs
The SEMs of hNPs were obtained from a JSM-6700F electron microscope (JEOL, Japan). The FTIR spectrums were recorded by Nicolet 5700 FTIR spectrometer (Thermo, USA). Elemental analysis was performed on an elemental analyzer (vario MICRO CUBE, Germany) by using 5 mg of the sample.
2.4. Regioselective acylation of arbutin
Regioselective acylation of arbutin was selected as a model reaction to evaluate the effect of immobilization. The reaction was carried out according to our previous work ( Citation32) with minor modification. Arbutin (200 mg) and vinyl acetate (2.75 mL) were mixed into tetrahydrofuran (THF, 20 mL, aw, 0.63). The reaction was started by adding the hNPs (1.4 g, containing 100 mg CSL) under magnetic stirring (100 rpm/min, 40°C). HPLC was used to monitor the reaction. The enzyme activity (μmol/mg/h) was defined as the amount (μmol) of the arbutin ester produced per hour per milligram of enzyme. The product was purified and characterized by NMR according to our previous research ( Citation32). Each experiment was performed in triplicates to obtain the average values.
2.5. HPLC analytical procedure
A Thermo C18 column (4.6 mm × 250 mm × 5 μm, USA) was equipped for HPLC analytical procedure. The mobile phase (flow rate: 1.0 mL/min) is a mixture of water (A) and methanol (B) with a gradient program of 10–30% (B) for 0–5 min, 30% (B) for 5–25 min and 30–10% (B) for 25–30 min. HPLC was monitored by a UV detector at 282 nm. Arbutin and its 6’-O-acetate could be observed at 5.689 min and 11.426 min, respectively.
3. Results and discussion
3.1. The morphologies of the hNPs
Many nanoparticles were prepared successfully ((A),(B)). The fabricated hNP looked like a beautiful flower in nature and its diameter was about 4–5 μm. It has a large number of separate petals ((C)), which can disperse the enzyme well and then enhance the enzyme performance. EDAX analysis was used to confirm the major components of the fabricated sample. The results ((D)) indicated that the prepared hNPs were mainly composed of zinc phosphate and protein.
3.2. Characterization of the prepared hNPs
FTIR, XRD and elemental analysis were used to characterize the produced hNPs. is the FTIR spectra of hNPs and its raw materials. The vibrations of the amide I and II bands of the protein (
Citation33) could be observed at 1547 cm−1 and 1406 cm−1. The vibrations of (
Citation34) could also be found at 1111 cm−1, 943 cm−1 and 634 cm−1. Thus, the FTIR spectrum of the prepared hNPs (curve b) contained all these characteristic peaks, which verified the existence of protein in the prepared hNPs.
Figure 2. The FTIR spectrum of free CSL (curve a), the prepared hNPs (curve b), and zinc phosphate (curve c).
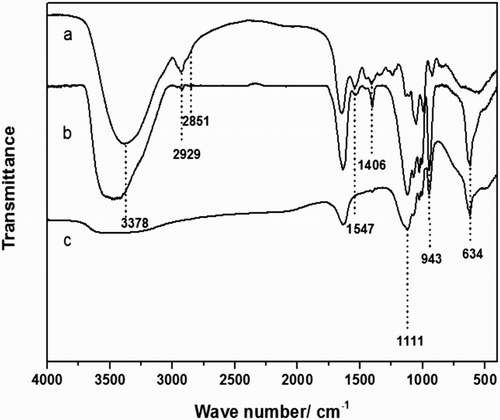
Power XRD analysis was performed to investigate the crystal structure of the prepared hNPs. The XRD patterns of CSL, zinc phosphate and the prepared hNPs were illustrated in . It could be found that the XRD pattern of the prepared hNPs contained the diffraction peaks of protein.
Element content data of the hNPs and its raw materials are compared in . N element content of zinc phosphate, CSL and the prepared nanoparticles were 0.050%, 6.810% and 0.540%, respectively. These results suggested that N element of the nanoparticles mainly come from the protein composition. The protein content in the prepared hNPs has been calculated to be about 7.20%.
Table 1. Element content of the prepared hNPs and its raw materials.
3.3. Effect of CSL concentration
Enzyme concentration during immobilization may affect the enzyme performance via diffusional limitations and also its stability via enzyme-enzyme interactions ( Citation35–37). So, we investigated the effect of CSL concentration. Various concentrations of CSL were used to prepare the hNPs. After separated and dried at room temperature, the morphologies of prepared hNPs were recorded by SEM (). The binding amount of CSL in hNPs and enzyme activity for regioselective acylation of arbutin have also been determined. The results were demonstrated in .
Figure 4. Morphologies of the hNPs prepared with different dosage of CSL (A, 0 mg/mL; B, 0.2 mg/mL; C, 0.4 mg/mL; D, 0.6 mg/mL; E, 0.8 mg/mL; F, 1.0 mg/mL).
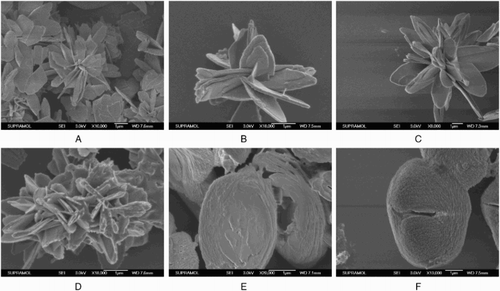
Figure 5. Effect of CSL concentration on the binding amount of CSL in hNPs and the enzyme activity of hNPs for regioselective acylation of arbutin. Reaction condition: THF (20 mL, aw = 0.63), arbutin (200 mg), vinyl acetate (2.75 mL) and hNPs (containing 100 mg of CSL) at 40°C.
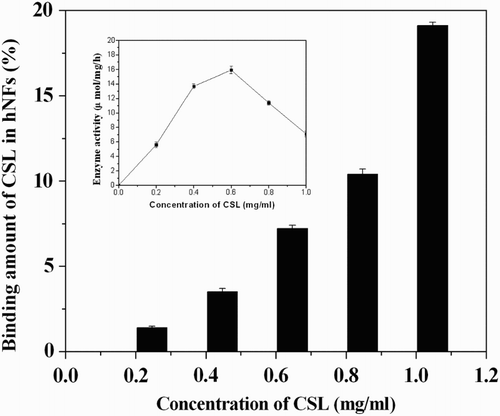
As shown in and , increasing the concentration of CSL could enhance the binding amount of CSL in hNPs. And the hierarchical structure of hNPs became denser simultaneously. However, the highest enzyme activity could be obtained when the prepared concentration of CSL was 0.6 mg/mL. High dosage of CSL (>0.6 mg/mL) will trigger the overloading of enzyme and result in a compact hierarchical structure, which may decrease the mass transfer efficiency and reduce the enzyme activity of hNPs.
3.4. The prepared hNPs vs free CSL
compared the enzymatic performance of the free CSL and hNPs for regioselective acylation of arbutin. The results showed that this kind of immobilization method could not destroy the regioselectivity of CSL. Compared with the free enzyme, the enzyme activity of CSL was enhanced about 4.3-folds after immobilization. The aggregated phenomenon for the free enzyme could be observed in this reaction system. On the contrary, the prepared hNPs dispersed the enzyme well due to its high ratios of surface to volume ( Citation8, Citation15–17), which can increase the catalytic performance of the enzyme.
Table 2. Comparison of the catalytic properties of free CSL and the prepared hNPs for regioselective acylation of arbutin.
3.5. Reusability
The nano-biocatalyst was recovered and reused in the next reaction batch for regioselective acylation of arbutin. After 10 continuous reaction-recycles, the immobilized enzyme lost only 6% of the enzyme activity (), which indicated that the fabricated hNPs have excellent reusability for this reaction.
4. Conclusions
In summary, we reported a facile and rapid method for preparation of the lipase-inorganic hNPs. The prepared nano-biocatalyst exhibited enhanced enzyme activity and excellent reusability in the regioselective acylation of arbutin. The simple fabrication procedure is carried out in aqueous solution under ambient conditions with low operational cost, which is promising for applications in industrial biocatalysis.
Disclosure statement
No potential conflict of interest was reported by the authors.
Notes on contributors
Lu Zheng is a doctoral candidate in Key Laboratory of Molecular Enzymology and Engineering of Ministry of Education, School of Life Sciences (Jilin University, Changchun, China). Her research has focused on the biocatalysis and biotransformation.
Xiaona Xie is working in the first hospital of Jilin University in Changchun City. Now, she is studding in Key Laboratory of Molecular Enzymology and Engineering of Ministry of Education (Jilin University, Changchun, China) for the synthesis and development of chiral drugs.
Zhi Wang was born in 1973. He obtained his Ph.D. degree in 2003. Recently, his research has focused on the enzymatic resolution of chiral compounds, green chemistry and medicinal chemistry.
Yuxin Zhang is studding as a doctoral candidate in School of Life Sciences, Jilin University. Her research has focused on the biocatalysis and biotransformation.
Lei Wang is working in Key Laboratory of Molecular Enzymology and Engineering of Ministry of Education (Jilin University, Changchun, China) as a professor. Recently, his research has focused on the new synthetic methodologies to synthesize bioactive compounds.
Xiuyun Cui is studding as a master candidate in School of Life Sciences, Jilin University. Her research has focused on the synthesis and development of new drugs.
He Huang is studding as a master candidate in School of Life Sciences, Jilin University. Her research has focused on the synthesis of bioactive compounds.
Hong Zhuang, female, born in 1974, professor. She is working in College of Food Science and Engineering, Jilin University, Changchun. Her research interest is food chemistry, mainly engaged in the design, development and detection of functional foods.
Additional information
Funding
References
- Spahn, C.; Minteer, S.D. Enzyme Immobilization in Biotechnology. Recent Pat. Eng. 2008, 2 (3), 195–200. doi: 10.2174/187221208786306333
- Cao, L. Immobilised Enzymes: Science or Art? Curr. Opin. Chem. Biol. 2005, 9 (2), 217–226. doi: 10.1016/j.cbpa.2005.02.014
- Torres-Salas, P.; del Monte-Martinez, A.; Cutiño-Avila, B.; Rodriguez-Colinas, B.; Alcalde, M.; Ballesteros, A.O.; Plou, F.J. Immobilized Biocatalysts: Novel Approaches and Tools for Binding Enzymes to Supports. Adv. Mater. 2011, 23 (44), 5275–5282. doi: 10.1002/adma.201101821
- Datta, S.; Christena, L.R.; Rajaram, Y.R.S. Enzyme Immobilization: An Overview on Techniques and Support Materials. 3 Biotech. 2013, 3 (1), 1–9. doi: 10.1007/s13205-012-0071-7
- Sheldon, R.A.; van Pelt, S. Enzyme Immobilisation in Biocatalysis: Why, What and How. Chem. Soc. Rev. 2013, 42 (15), 6223–6235. doi: 10.1039/C3CS60075K
- Eş, I.; Vieira, J.D.G.; Amaral, A.C. Principles, Techniques, and Applications of Biocatalyst Immobilization for Industrial Application. Appl. Microbiol. Biotechnol. 2015, 99 (5), 2065–2082. doi: 10.1007/s00253-015-6390-y
- Mateo, C.; Palomo, J.M.; Fernandez-Lorente, G.; Guisan, J.M.; Fernandez-Lafuente, R. Improvement of Enzyme Activity, Stability and Selectivity via Immobilization Techniques. Enzyme Microb. Technol. 2007, 40 (6), 1451–1463. doi: 10.1016/j.enzmictec.2007.01.018
- Altinkaynak, C.; Tavlasoglu, S.; ÿzdemir, N.; Ocsoy, I. A New Generation Approach in Enzyme Immobilization: Organic-inorganic Hybrid Nanoflowers with Enhanced Catalytic Activity and Stability. Enzyme Microb. Technol. 2016, 93–94, 105–112. doi: 10.1016/j.enzmictec.2016.06.011
- Palomo, J.M.; Muñoz, G.; Fernández-Lorente, G.; Mateo, C.; Fernández-Lafuente, R.; Guisán, J.M. Interfacial Adsorption of Lipases on Very Hydrophobic Support (octadecyl–Sepabeads): Immobilization, Hyperactivation and Stabilization of the Open Form of Lipases. J. Mol. Catal., B Enzym. 2002, 19–20, 279–286. doi: 10.1016/S1381-1177(02)00178-9
- Fernández-Lafuente, R.; Rodríguez, V.; Mateo, C.; Penzol, G.; Hernández-Justiz, O.; Irazoqui, G.; Villarino, A.; Ovsejevi, K.; Batista, F.; Guisán, J.M. Stabilization of Multimeric Enzymes via Immobilization and Post-immobilization Techniques. J. Mol. Catal., B Enzym. 1999, 7 (1–4), 181–189. doi: 10.1016/S1381-1177(99)00028-4
- Xie, W.; Zang, X. Covalent Immobilization of Lipase Onto Aminopropyl-functionalized Hydroxyapatite-encapsulated-γ-Fe2O3 Nanoparticles: A Magnetic Biocatalyst for Interesterification of Soybean Oil. Food Chem. 2017, 227, 397–403. doi: 10.1016/j.foodchem.2017.01.082
- Xie, W.; Zang, X. Immobilized Lipase on Core–shell Structured Fe3O4–MCM-41 Nanocomposites as a Magnetically Recyclable Biocatalyst for Interesterification of Soybean Oil and Lard. Food Chem. 2016, 194, 1283–1292. doi: 10.1016/j.foodchem.2015.09.009
- Xie, W.; Wang, J. Enzymatic Production of Biodiesel from Soybean Oil by Using Immobilized Lipase on Fe3O4/poly (Styrene-methacrylic Acid) Magnetic Microsphere as a Biocatalyst. Energy Fuels. 2014, 28 (4), 2624–2631. doi: 10.1021/ef500131s
- Garcia-Galan, C.; Berenguer-Murcia, Á; Fernandez-Lafuente, R.; Rodrigues, R.C. Potential of Different Enzyme Immobilization Strategies to Improve Enzyme Performance. Adv. Synth. Catal. 2011, 353 (16), 2885–2904. doi: 10.1002/adsc.201100534
- Ge, J.; Lei, J.; Zare, R.N. Protein–inorganic Hybrid Nanoflowers. Nat. Nanotechnol. 2012, 7, 428–432. doi: 10.1038/nnano.2012.80
- Yin, Y.; Xiao, Y.; Lin, G.; Xiao, Q.; Lin, Z.; Cai, Z. An Enzyme-inorganic Hybrid Nanoflower Based Immobilized Enzyme Reactor with Enhanced Enzymatic Activity. J. Mater. Chem. B. 2015, 3 (11), 2295–2300. doi: 10.1039/C4TB01697A
- Lin, Z.; Xiao, Y.; Wang, L.; Yin, Y.; Zheng, J.; Yang, H.; Chen, G. Facile Synthesis of Enzyme-inorganic Hybrid Nanoflowers and Their Application as An Immobilized Trypsin Reactor for Highly Efficient Protein Digestion. RSC Adv. 2014, 4 (27), 13888–13891. doi: 10.1039/C4RA00268G
- Cui, J.; Jia, S. Organic–inorganic Hybrid Nanoflowers: A Novel Host Platform for Immobilizing Biomolecules. Coord. Chem. Rev. 2017, 352, 249–263. doi: 10.1016/j.ccr.2017.09.008
- Fernández-Lorente, G.; Palomo, J.M.; Cabrera, Z.; Guisán, J.M.; Fernández-Lafuente, R. Specificity Enhancement Towards Hydrophobic Substrates by Immobilization of Lipases by Interfacial Activation on Hydrophobic Supports. Enzyme Microb. Technol. 2007, 41 (5), 565–569. doi: 10.1016/j.enzmictec.2007.05.004
- Fernandez-Lorente, G.; Cabrera, Z.; Godoy, C.; Fernandez-Lafuente, R.; Palomo, J.M.; Guisan, J.M. Interfacially Activated Lipases Against Hydrophobic Supports: Effect of the Support Nature on the Biocatalytic Properties. Process Biochem. 2008, 43 (10), 1061–1067. doi: 10.1016/j.procbio.2008.05.009
- Suescun, A.; Rueda, N.; dos Santos, J.C.; Castillo, J.J.; Ortiz, C.; Torres, R.; Barbosa, O.; Fernandez-Lafuente, R. Immobilization of Lipases on Glyoxyl–octyl Supports: Improved Stability and Reactivation Strategies. Process Biochem. 2015, 50 (8), 1211–1217. doi: 10.1016/j.procbio.2015.05.010
- Wilson, L.; Palomo, J.M.; Fernández-Lorente, G.; Illanes, A.; Guisán, J.M.; Fernández-Lafuente, R. Effect of Lipase–lipase Interactions in the Activity, Stability and Specificity of a Lipase from Alcaligenes sp. Enzyme Microb. Technol. 2006, 39 (2), 259–264. doi: 10.1016/j.enzmictec.2005.10.015
- Wilson, L.; Palomo, J.M.; Fernández-Lorente, G.; Illanes, A.; Guisán, J.M.; Fernández-Lafuente, R. Improvement of the Functional Properties of a Thermostable Lipase from Alcaligenes sp. via Strong Adsorption on Hydrophobic Supports. Enzyme Microb. Technol. 2006, 38 (7), 975–980. doi: 10.1016/j.enzmictec.2005.08.032
- Choudhury, P. Industrial Application of Lipase: A Review. Biopharm. 2017, 1 (2), 1–47.
- Ghanem, A.; Aboul-Enein, H.Y. Application of Lipases in Kinetic Resolution of Racemates. Chirality. 2005, 17 (1), 1–15. doi: 10.1002/chir.20089
- Zhang, J.; Shi, H.; Wu, D.; Xing, Z.; Zhang, A.; Yang, Y.; Li, Q. Recent Developments in Lipase-catalyzed Synthesis of Polymeric Materials. Process Biochem. 2014, 49 (5), 797–806. doi: 10.1016/j.procbio.2014.02.006
- Aransiola, E.F.; Ojumu, T.V.; Oyekola, O.O.; Madzimbamuto, T.F.; Ikhu-Omoregbe, D.I.O. A Review of Current Technology for Biodiesel Production: State of the Art. Biomass Bioenergy. 2014, 61, 276–297. doi: 10.1016/j.biombioe.2013.11.014
- Stergiou, P.-Y.; Foukis, A.; Filippou, M.; Koukouritaki, M.; Parapouli, M.; Theodorou, L.G.; Hatziloukas, E.; Afendra, A.; Pandey, A.; Papamichael, E.M. Advances in Lipase-catalyzed Esterification Reactions. Biotechnol. Adv. 2013, 31 (8), 1846–1859. doi: 10.1016/j.biotechadv.2013.08.006
- Wu, Z.; Li, X.; Li, F.; Yue, H.; He, C.; Xie, F.; Wang, Z. Enantioselective Transesterification of (R,S)-2-pentanol Catalyzed by a New Flower-like Nanobioreactor. RSC Adv. 2014, 64 (4), 33998–34002. doi: 10.1039/C4RA04431B
- An, B.; Fan, H.; Wu, Z.; Zheng, L.; Wang, L.; Wang, Z.; Chen, G. Ultrasound-assisted Enantioselective Esterification of Ibuprofen Catalyzed by a Flower-like Nanobioreactor. Molecules. 2016, 21 (5), 565. doi: 10.3390/molecules21050565
- Rodrigues, R.C.; Ortiz, C.; Berenguer-Murcia, Á.; Torres, R.; Fernández-Lafuente, R. Modifying Enzyme Activity and Selectivity by Immobilization. Chem. Soc. Rev. 2013, 42 (15), 6290–6307. doi: 10.1039/C2CS35231A
- Jiang, L.; Xie, X.; Yue, H.; Wu, Z.; Wang, H.; Yang, F.; Wang, L.; Wang, Z. Highly Efficient and Regioselective Acylation of Arbutin Catalyzed by Lipase from Candida sp. Process Biochem. 2015, 50 (5), 789–792. doi: 10.1016/j.procbio.2015.02.014
- Yang, W.-J.; Griffiths, P.R.; Byler, D.M.; Susi, H. Protein Conformation by Infrared Spectroscopy: Resolution Enhancement by Fourier Self-deconvolution. Appl. Spectrosc. 1985, 39 (2), 282–287. doi: 10.1366/0003702854248917
- Yu, Y.; Fei, X.; Tian, J.; Xu, L.; Wang, X.; Wang, Y. Self-assembled Enzyme–inorganic Hybrid Nanoflowers and Their Application to Enzyme Purification. Colloids Surf., B. 2015, 130, 299–304. doi: 10.1016/j.colsurfb.2015.04.033
- Fernandez-Lopez, L.; Pedrero, S.G.; Lopez-Carrobles, N.; Gorines, B.C.; Virgen-Ortíz, J.J.; Fernandez-Lafuente, R. Effect of Protein Load on Stability of Immobilized Enzymes. Enzyme Microb. Technol. 2017, 98, 18–25. doi: 10.1016/j.enzmictec.2016.12.002
- Rueda, N.; dos Santos, J.; Ortiz, C.; Torres, R.; Barbosa, O.; Rodrigues, R.C.; Berenguer-Murcia, Á.; Fernandez-Lafuente, R. Chemical Modification in the Design of Immobilized Enzyme Biocatalysts: Drawbacks and Opportunities. Chem. Rec. 2016, 16 (3), 1436–1455. doi: 10.1002/tcr.201600007
- Zaak, H.; Siar, E.-H.; Kornecki, J.F.; Fernandez-Lopez, L.; Pedrero, S.G.; Virgen-Ortíz, J.J.; Fernandez-Lafuente, R. Effect of Immobilization Rate and Enzyme Crowding on Enzyme Stability Under Different Conditions. The Case of Lipase from Thermomyces Lanuginosus Immobilized on Octyl Agarose Beads. Process Biochem. 2017, 56, 117–123. doi: 10.1016/j.procbio.2017.02.024