ABSTRACT
Recently, green synthesis of silver nanoparticles has attracted much curiosity in the field of life science research. In the present study, we have reported a green method for synthesis of silver nanoparticles (AgNPs) using aqueous seed extract of Phoenix sylvestris L. The green synthesized nanoparticles were characterized by aids of dynamic light scattering, scanning electron microscopy, transmission electron microscopy, X-ray diffraction, and Fourier transform-infrared. Further, the study demonstrate the comparative phytochemical analysis as well as antioxidant and antibacterial activity of the extract and Phoenix sylvestris seed generated nanoparticles against acne-causing pathogens, that is, by using a DPPH-scavenging assay and broth microdilution method as well as Kirby–Bauer Disk diffusion method (recommended by CLSI), respectively. Moreover, a concentration-dependent time-kill kinetic studies were also carried out to determine their antimicrobial activity. The seed extract was found a better antioxidant and AgNPs exhibited highly biocidal agent against both the test pathogens, when compared to aqueous extracts. The results obtained indicate that seed extract of P. sylvestris is suitable for synthesizing stable silver nanoparticles, which act as excellent antimicrobial agents with promising treatments for cosmetics embarrassment.
GRAPHICAL ABSTRACT
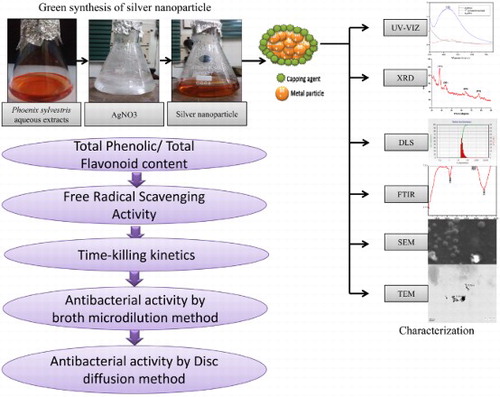
1. Introduction
The biogenic mediated nanotechnology implies the application of green chemistry percepts for nanoparticle fabrication. The biogenic approaches strive to develop the method for nanoparticle synthesis that offers clean, eco-friendly, non-toxic synthesis machinery and compatible for pharmaceutical and cosmeceutical applications. Therefore, use of biogenic approaches for the green synthesis of nanoaparticle is currently a matter of great concern ( Citation1, Citation2).
The use of plant extracts has been extensively examined for metallic nanoparticle synthesis as they improve the monodispersity of nanoparticles. The biomolecules like phenolics, polysaccharides, flavones, terpenoids, alkaloids, proteins, amino acids, enzymes and alcoholic compounds present in plants act as both reducing agents as well as capping agents that stabilize and govern the morphology of NPs ( Citation3, Citation4). Green synthesis of metallic NPs by using plant extracts has extensively been employed in recent research ( Citation5–7). Nanoparticles possess completely novel physical (optical, magnetic and electronic) and chemical properties. Size range, self-assembly and high antimicrobial activity of silver nanoparticles (AgNPs) regarded as important for their potential role in medical devices, optical devices, electronics biotechnologies and catalysis ( Citation8, Citation9). Small size, spherical shape and high surface-area-to-volume ratio of AgNPs facilitate them to interact with the cell walls of pathogens, which gives them better antimicrobial activity ( Citation10).
Phoenix sylvestris L. is a Phoenicoid palm of the family Arecaceae, originated in semiarid regions with high water level ( Citation11). It is accounted for its conventional medicinal use by tribal folk for the treatment of various disorders (memory disturbances, fever, inflammation, paralysis, loss of consciousness and nervous disorders) ( Citation12, Citation13). Phoenix sylvestris is accountable as a virtuous source of immunomodulatory compounds with low cytotoxicity and potent antioxidant activity. We hypothesized that these pharmacological properties of P. sylvestris can prove potent cosmeceutical agent in acne treatments. Therefore, in present study, the biological approach using seed exudates of P. sylvestris has been used as a reducing material as well as surface stabilizing agent for the synthesis of silver nanoparticle, and the comparative effects of P. sylvestris Ag-NPs, aqueous extract and bulk have been evaluated for the first time against Propionibacterium acnes (anaerobic bacteria) and Staphylococcus epidermidis (aerobic bacteria).
P. acnes is gram-positive, facultative, anaerobic and rod-shaped bacteria that mainly inhabit the human skin with S. epidermidis ( Citation14). Though P. acnes could be considered as chief causative agent of invasive infections of skin, soft tissues, deep organ tissues associated with acnes vulgaris pervasiveness ( Citation15). In this paper, we have used seed extract of P. sylvestris for the synthesis of stable AgNPs, followed by evaluation of its antioxidant and antibacterial activity.
2. Material and method
2.1. Collection of materials
The healthy dates were collected from the local market of Allahabad (Uttar Pradesh), India. Seeds were procured out off the fruits and washed with distal water and shade dried. AgNO3 was purchased from Hi-Media® Pvt. Ltd.
2.2. Test organisms
P. acne (MTCC 1951) and S. epidermidis (MTCC 451) used as test bacteria were procured from microbial type culture collection, IMTECH, Chandigarh, India.
2.3. Preparation of seed extract
The seeds of P. sylvestris were washed several times with tap water followed by double distilled water. 10 gm of seeds were socked in 100 ml of sterilized doubled distilled water, and subjected to magnetic stirrer with 45–45°C with constant stirring for up to 12 h. Crude extract was filtered through muslin cloth and then with Whatman filter paper 1 two times to obtain a clear extract, which was later used for phytochemical analysis and synthesis. Subsequently, the extract solutions followed by evaporation under vacuum and optimal temperature in rotatory evaporator apparatus to obtain crude extracts. Further the extracts had been dried completely and calculated percentage yield, and stored in refrigerator freezer at 4°C for experimental use.
2.4. Phytochemical studies
2.4.1. Total flavonoids content
The seed aqueous extract was tested for flavonoids by the method of Kim et al. ( Citation16). The 5 mL aqueous extract (50 mg/mL concentrated) was added to 0.3 ml of sodium nitrite solution (5%) and aluminum chloride solution (10%). The mixture was incubated at room temperature for 5 minutes followed by addition of 2 ml 1.0 M of NaOH. The obtained mixture was made up to 10 mL with distal water and vortexed followed by Absorbance measurement at 510 nm using a spectrophotometer. Total flavonoid values were expressed in terms of Rutin equivalent (mg/100 g dry weight dates seed).
2.4.2. Total phenols content
The total phenolic content of seed extract was determined by a method demonstrated by the International Organization for Standardization (ISO 14502-1). The 100 µL of aqueous extract was mixed with 500 µl of 1/10 dilution of Folin–Ciocalteu reagent followed by addition of 400 µL of sodium carbonate solution (7.5%w/v). The mixture was incubated at room temperature for 60 min and absorbance at 765 nm was measured by using a spectrophotometer. Total phenol values were expressed in terms of Gallic acid equivalent (mg/100 g dry weight date seed) ( Citation17).
2.4.3. DPPH scavenging assay
The activity of seed extracts and AgNPs were evaluated using 2,2-diphenyl-1-picrylhydrazyl (DPPH). DPPH solutions (100 μg/mL) in methanol were made and the assay was performed in 96 well microtitre plates in triplicates. Both the extracts and Ascorbic acid (standard) were tested at 5, 4.375, 3.75, 3.125, 2.5, 1.875, 1.25, 0.625 mg/mL concentration and serially diluted to 0.625 mg/ml concentrations. After an incubation period of 30 min in dark at 25°C, absorbance was measured at A = 517 nm against blank. The Percentage effect of scavenging ability of the each plant extract on DPPH was calculated using the equation (
Citation18):where Abs control is the absorbance of DPPH + methanol; Abs sample is the absorbance of DPPH radical + sample extract or standard.
2.4.4. Efficient concentration
Efficient concentration (EC50) of DPPH was calculated by a linear equation of the dose inhibition curve obtained by plotting the extract concentrations versus corresponding percent radical scavenging activity using GraphPad Prism 5.01. Results were obtained as Mean ± Standard Deviation (SD) of three independent experiments for each antioxidant; EC50 values were expressed as 95% confidence interval.
2.4.5. The antioxidant radical power
The antioxidant radical power (ARP) was also calculated as follows: [ARP = 1/EC50] ( Citation19).
2.5. Synthesis of AgNPs
The aqueous solution of silver nitrate (1 mM) was prepared in 250 mL conical flasks and subjected to a magnetic stirrer with constant stirring, and leaf extract (1.5–2 ml) was added drop by drop (for reduction of Ag+ ions) under continuous stirring till the color changes. The color change of the mixture from faint light to yellowish brown to reddish brown to colloidal brown was supervised periodically (time and color change were noted) for a maximum of 30 min. The reactions were carried out in dark (to prevent photoactivation of AgNO3) at room temperature. Complete reduction of AgNO3 to Ag+ ions was reasserted by the change in color from colorless to brown. The obtained nanoparticle was subjected to confirmation by a spectrophotometric analysis (with peak around 420–430 nm). After confirmation, NPs are stored in dark for further characterization and antibacterial assessment.
2.6. Characterizations of AgNPs
2.6.1. UV–visible
Initially, characterization of the AgNPs (ultra-sonicated) was conducted by UV–visible spectroscopy. UV–visible spectrum of seed extract, bulk AgNO3 and synthesized Ag NPs (10 times diluted with deionized water) were monitored by using Lambda 35 Perkin Elmer spectrophotometer in the wavelength region 250–700 nm operated at a resolution of 1 nm. The UV–visible absorption spectra (numerical data) of these samples were plotted in the ‘Origin 8.1’ ((A)).
Figure 1. Characterization of green-synthesized AgNPs (A) UV–visible absorption spectra of the AgNPs, bulk AgNO3 and seed extract (B) Particle size distribution image of the AgNPs, (C) X-ray diffraction pattern of the Ag NPs (D) FTIR spectrum of the AgNPs, (E) SEM image of AgNPs and (F) TEM image of AgNPs.
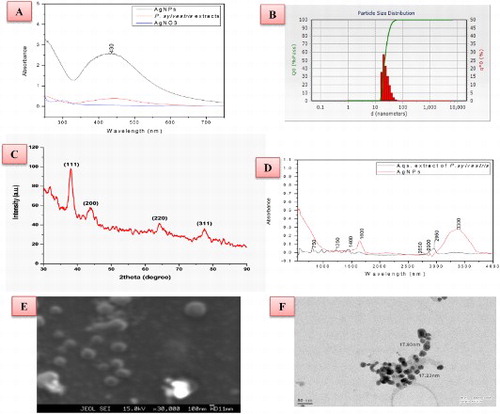
2.6.2. X-ray diffraction
Ag NPs were analyzed by an X-ray diffractometer. The sample was centrifuged and the obtained pallets were transformed to powder form. This metal powder was sticked in the cubes of X-ray diffraction (XRD) and then the result was taken in the XRD equipment (Bruker AXS diffractometer) using CuKα radiation (λ = 1.54056 Å) in the range of 20° ≤ 2θ ≤ 80°. The generator was operated at 40 keV and with a 30 mA current. The scanning range was selected between 15° and 100°. The particle size (D) of the sample was calculated using the Scherrer’s relationship:where λ is the wavelength of the X-ray, B is the broadening of the diffraction line measured as half of its maximum intensity in radians and θ is the Bragg’s diffraction angle ((C)).
2.6.3. DLS particle size analyzer
Dynamic light scattering (DLS) is a technique that can be used to determine the size distribution profile of small particles in suspension or polymers in solution. A laser diffraction method with a multiple scattering technique has been used to ascertain the size range of nanoparticle and distribution of the particle in a sample (Mie-scattering theory). In order to find out the particles size distribution of the Ag NPs, the powder was dispersed in ddw and ultrasonicated. Further experiments were carried out on a computer-based particle size analyzer (Nanotrac wave W3372) to find out the particle size distribution ((B)).
2.6.4. Fourier transforms infrared spectroscopy
The AgNPs were centrifuged at 20,000 rpm for 15 min and then the pellet (sediment) was collected. Fourier transform-infrared (FTIR) spectroscopy used for the analysis of AgNPs, using an IR beam of IR spectrometer (FTIR spectrum RX-1, Perkin Elmer) scanning the spectrum in the range 550–4000 cm−1 at a resolution of 16 cm−1 ((D)).
2.6.5. Scanning electron microscopy
For the morphological characters (shape and size), analyses of AgNPs scanning electron microscopy (SEM) were done by JEOL JXA-8230. The synthesized AgNPs sample was sonicated for 30 min; small drop of this sample was allowed to dry on a glass slide. NPs were thin layer coated on the copper grid and allowed to magnify the grid to record size and shape of NPs ((E)).
2.6.6. Transmission electron microscopy
Transmission electron microscopy (TEM) analysis was done by using TECNAI 200 kV instrument (SAIF AIIMS ID N-099). For TEM analysis, nanoparticles were diluted with DDW and dispersed by the ultrasonic bath. Further, one drop was placed on a carbon-coated copper grid to make a thin film and kept in the grid box sequentially for TEM analysis ((F)).
2.7. Antibacterial assay
The antibacterial susceptibility test of the P. sylvestris L. seed Ag NPs, aqueous extract and bulk was analyzed against pathogenic bacteria of acne (P. acnes and S. epidermidis) using the broth microdilution method recommended by the Clinical and Laboratory Standards Institute (CLSI) (NCCLS; 2003). Freshly prepared Mullur Hinton Broth medium was used for the assay. The Stock solutions of aqueous extract were prepared in 50 mg/ml of dimethyl sulfoxide (DMSO) and homogenized by using vortex for 4–5 min. Bacterial inocula were prepared as per 0.5 McFarland standard ( Citation20). The bacterial suspension was inoculated in two-fold serially diluted Ag NPs; Aqueous extract, bulk and Tetracycline as a standard were used to estimate antibacterial susceptibility. The experiment was performed in flat-bottom sterile 96-well microtitre plates. Initial dispensing of 100 μL medium (MHB) was followed by the addition of 90 and 80 μL of MHB in columns 3 and 4, respectively. Further, 10 and 20 μl of drugs (AgNPs, Aqueous extract, bulk and standard) were added in two-fold to each well of columns 3 (sample control) and 4 (dilution well), respectively. Followed by serial dilution from 4th column wells (2.5 mg/ml) to 11th column wells (0.02 mg/ml) and cast off from the last column.
Instantaneously, bacterial inoculum (100 μL) was added in each well of column 4 to column 12 that will maintain final volume of 200 μL. Column 1 serves as negative control that comprises media and formaldehyde. Column 12 was considered as the positive control (O. D. Control), which contains 100 μL medium and 100 μL inocula (). This 96 well plate was then transferred to the anaerobic jar of Anaxomate to provide an anaerobic environment. The jar was then incubated in a CO2 incubator (Galaxy 170 S New Brunswick, USA) for 24–48 hr (
Citation21).
2.7.1. Time killing kinetics
The time killing of P. sylvestris seed aqueous extracts, and green synthesized AgNPs, bulk and standard were determined by measuring the absorbance of the prepared 96 well plates at an interval of 2.5, 5, 7.5, 10, 15, 20, 25 h. The data obtained show the concentration-dependent killing rate in terms of percent inhibition based on absorbance ( and ).
2.7.2. Determination of minimum inhibitory concentrations and IC50
The minimum inhibitory concentrations (MICs) and IC50 were obtained by measuring absorbance using a spectrophotometer. The tests were implemented in a twofold manner. For AgNPs, aqueous extracts, bulk and standard, the MIC was resolute as the lowest drug concentration showing the absence of growth visually or 80% growth inhibition compared with the growth in the drug-free well. IC 50 is defined as the drug concentration that produces 50% of growth inhibition compared to the growth in the drug-free well. Comparative inhibition percent of bacteria inocula in medium treated with herbal extracts and oil was calculated by using the following formula:
2.8. Antibacterial activity by Kirby–Bauer disk diffusion method
The Kirby–Bauer disk diffusion methods are used for antimicrobial susceptibility testing recommended by the CLSI. The bacterial inocula of each microorganism were inoculated by streaking the swab over the entire sterile agar surface separately. The sterile disks (6 mm of Whatman No 1) were dipped in Ag NPs, AgNO3 and plants extract (50 μg/ml). These disks were air-dried under sterile conditions, and placed onto the seeded top layer of the MHA plates, and incubated at 37°C for 24 h. Diameters (mm) of the zones of bacterial inhibition minus the disks diameter were recorded ( Citation22). Tetracycline disks were used as positive controls.
3. Results and discussion
The present study explains the green synthesis of AgNPs from seed extract of P. sylvestris and evaluation of their phytochemical, antioxidant and antibacterial activity. NPs are generally characterized by their size, shape, surface area, and disparity as homogeneity of nanoproperties is important in its applications aspects ( Citation6). When 1.5–2 ml of the seed extract was added to the 1 mM AgNO3 solution with continuous stirring on a magnetic stirrer within 5–6 min of the reaction, its color changed from colorless to dark golden brown, indicating aqueous Ag+ ions isbio-reduced by aqueous seed extract to generate extremely stable silver nanoparticles in water ( Citation23, Citation24). The bioreduction might be done by the phytochemicals of the plant extracts. Among the phytochemicals, phenolic contents serve as strong reducing agents by donating electrons to Ag+ ions and reduced to form Ag° nanoparticles, hence color changes ( Citation25, Citation26). The UV–visible spectra of AgNPs, bulk AgNO3 and seed extracts are shown in (A). The particles of the sample absorb light at the visible and adjacent range and get excited due to charge density at the interface between conductor and insulator of a UV–visible spectroscope to give a respective peak (known as surface plasmon resonance). The absorption peak obtained at 440 nm is a characteristic peak for metallic nanoparticles, which further confirms that the reduced nanoparticles are silver ( Citation27, Citation28), and for bulk AgNO3, the absorption maximum peak usually falls around 278 nm. The absorption or reflectance in the visible range directly affects the apparent color of the chemical involved ( Citation29).
The XRD pattern of the green-synthesized AgNPs was carried out to affirm the crystalline nature of nanoparticles. The XRD pattern shows four peaks on 20° of X-axis like 38°, 44°, 64° and 78° analogous to 111, 200, 220 and 311 Bragg reflections of Y-axis, respectively ((C)). These Bragg reflections proposed that X-ray scattering face-centered cubic structure of NPs could serve as a capping agent. The peaks coincide with Joint Committee on Power Diffraction Standards (JCPDS pdf no: 89-3722). The average crystalline size of NPs is measured at 43 nm from corresponding XRD peaks according to the Debye–Scherrer equation ((D = 0.9λ/(Bcos θ) ( Citation30, Citation31).
DLS can be defined as the size distribution profile of small particles in suspension. The dimension depends on the size of the particle core, the size of the surface structure, particle concentration and the type of ions in the medium. DLS study demonstrates that the maximum particles in the solution are in the range of 50–60 nm. Since it predominantly measures variations in scattered light intensity due to diffusing particles, the diffusion coefficient of the particles can be ascertained ((B)).
FT-IR spectrum of synthesized NPs was carried out to know the probable bio-molecules functional group of P. sylvestris seed extract responsible for the capping and stabilization of nanoparticles. The FTIR spectrum of green synthesized AgNPs are shown in (D). The sample was examined in the scan range from 4000 to 500 cm−1 of near IR spectra. According to the IR spectrum, the broad peak at 3300 cm−1 is due to stretching vibration of O-H of phenols and peak at 1600 cm−1 is due to N–H bond of primary amines, whereas two minor peaks at 2900 and 2850 cm−1 can be due to the –O–H– and C = O stretching vibrations. Proposing that the O–H group of phenols, amide groups of proteins aromatic and carbonyl groups of the protein forming a layer of the NPs and these act as potential capping agents to prevent accumulation of the particle and provide stability to the medium ( Citation32, Citation33).
SEM delivers insight of the morphology and size of the AgNPs. The SEM image of green synthesized NPs is shown in (E). The morphology of particles was found to be nearly irregular to spherical. A higher magnification study was carried out with TEM analysis to know the size and shape of the nanoparticles along with crystalline nature. 100 nm resolution studies of synthesized NPs show 40–50 nm size with predominant spherical shape and they do not have any agglomeration ((E)). The size determined by DLS is hydrodynamic diameter of NPs (includes the Brownian movement of the liquid film), which seems larger than the real particle size while XRD-defined crystalline size seems smaller than the real particle size. In contrast, SEM/TEM measures real diameter, which is greater than crystalline diameter (XRD) ( Citation29).
The total phenolic content and flavonoid content of aqueous extracts along with radicals scavenging activity by DPPH assay of aqueous extracts and AgNPs has also been determined. Our data have validated that the mean phenolic content (PC) in P. sylvestris seed aqueous extracts is considerably good, compared with the standard (Gallic acid) 68.03–89.27 mg and flavonoid content (FC) from 27.44 to 48.06 (). Phenolic content present in plant extracts exhibited high antioxidant activity and serves as a reducing agent, which are important for the synthesis of AgNPs ( Citation34). The higher total phenolic content of P. sylvestris seed extract, finner the aasemly of silver ions (Ag+) to AgNPs because donation of electrons by these phenolic compunds ( Citation26). The Free radical scavenging activity of seed extracts and AgNPs are depicted in compared with the standard (ascorbic acid), showing a more free radical scavenging activity of AgNPs than P. sylvestris seed extracts. Further efficient concentrations (EC50:concentration of a drug that gives half-maximal response) of both the sample and ascorbic acid is listed in . The efficient concentration of ascorbic acid was 1.78 mg/mL. AgNPs scavenged 50% of DPPH at 3.00 mg/mL, whereas P. sylvestris seed aqueous extracts scavenged its 50% of DPPH at 2.28 mg/mL, as depicted in . The obtained data showed a statistically significant correlation between phytoconstituents analyses, that is, total phenols and total flavonoids and DPPH assay (). Moreover, seed extracts having higher scavenging activity might be due to the higher phenolic content ( Citation35). The phenolic contents having antioxidant potentiality, essentially due to its ability to improve radical stability through the formation of an intramolecular hydrogen bond between the free hydrogen of the hydroxyl group and its phenoxyl radicals, which interprets the fact that phenolic phytoconstituents are less reactive and possess lower electron reduction potential than the oxygen radicals ( Citation36).
Figure 3. Free radical scavenging activity of green-synthesized silver nanoparticles, P. sylvestris seed extracts and Standard.
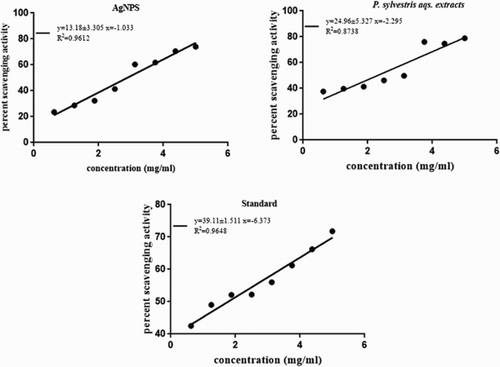
Table 1. Total phenolic content, flavonoid content and 50% scavenging activity (EC50) and ARP estimation of P. sylvestris seed aqueous extracts, silver nanoparticle along with standard.
Regarding the antibacterial activity of AgNPs and aqueous extract of P. sylvestris, our data clearly portray significant antibacterial properties with reference to the MICs as well as IC50 (mg/mL) values through 96 microtitre well plate (CLSI recommended broth micro-dilution method). The AgNPs were found to be highly biocidal against both the test pathogen, anaerobic bacteria P. acnes with MIC (mg/mL): 0.687, IC50:0.650 and aerobic bacteria S. epidermidis with MIC: 0.693, IC50:0.662, but still more active toward anaerobic pathogen.The biocidal activity of P. sylvestris aqueous extracts reveals a high activity against P. acnes MIC: 1.344, IC50: 1.298 than S. epidermidis MIC: 1.345, IC50: 1.301(). These quantitative value were compared by control (tetracycline) reading against P. acnes (MIC: 0.355, IC50: 0.344) and S. epidermidis (MIC: 0.372, IC50:0.362) (). The time-kill kinetics profiles of AgNPs along with concentration (against P. acnes and S. epidermidis test organisms) were observed to be bacteriostatic and concentration dependent. The time-kill kinetics profile of AgNPs showed a gradual reduction in the number of viable cells over the first 5–7 hours at all the given concentration, followed by an impulsive reduction up to the 20th hour and steady reduction of cells. When compared to the extract, it shows gradual and steady reduction up to 10–12 h and considerable viable cell reduction till 24 h. The time-killing profile of bulk and control is comparable to the profile of NPs. However, the Killing profile of NPs is higher when compared in lower concentration and much earlier. It might be due to its unique size, which facilitates its permeation into the cell easily and hence causes cell damage. From a clinical point of view, it is very interesting to note that the susceptibility of P. acnes () appeared to be significantly higher than that of S. epidermidis () ( Citation37). Both species belong to the bacterial flora of the skin, but only P. acnes is reflected to be chief the pathogenetic agent of the chronic skin inflammation in acne vulgaris ( Citation38–40). Comparatively, susceptibility of P. acnes is higher than that of S. epidermidis.
Figure 4. Broth microdilution Antibacterial assay of P. sylvestris L. seed extracts and Green synthesized AgNPs against P. acnes and S. epidermidis.
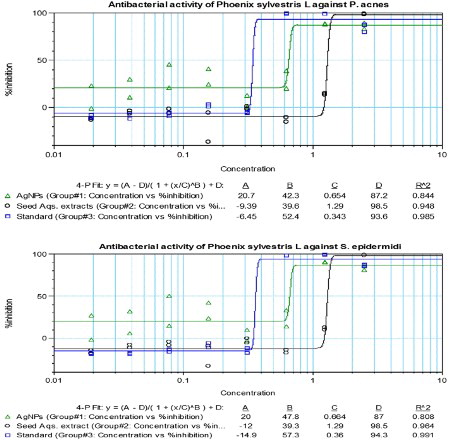
Figure 5. The concentration-dependent time-kill kinetics on P. acnes of (A) P. sylvestris extracts, (B) AgNPs, (C) Bulk and (D) Standard.
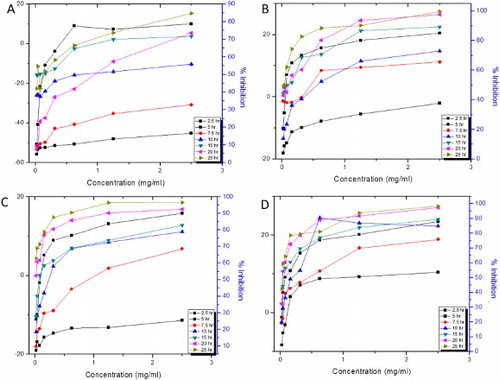
Figure 6. The concentration-dependent time-kill kinetics on S. epidermidis of (A) P. sylvestris extracts, (B) AgNPs, (C) Bulk and (D) Standard.
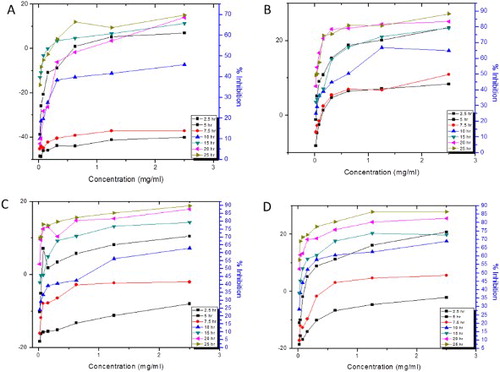
Table 2. Broth microdilution Antibacterial assay of P. sylvestris L. seed extracts and green-synthesized AgNPs against acne vulgaris bacterial pathogens.
Moreover, antimicrobial activity assay by disk diffusion methods (in terms of zone of inhibition) reveals the high antimicrobial activity of AgNPs, which is comparable to the activity of bulk (AgNO3), whereas seed extract shows comparatively low antibacterial activity against both the pathogen compared with standard (, ). The high antibacterial activity of AgNPs has been reported in series of studies ( Citation31). High antimicrobial activity may be attributed to the finner AgNPs which penetrate through flexible cell walls of bacteria, where the cell walls are made up of peptidoglycan (a carbohydrate polymer) linked by amino acids and cross-linked by tetrapeptides ( Citation41). Silver cations released from AgNPs cause damage by interacting with the electrons of phosphorous- and sulphur-containing compounds such as DNA and proteins, and subsequently cell death ( Citation42–44). Some studies reported that, AgNPs may attach to the cell membrane, disturb their permeability and cause structural changes in bacteria ( Citation45, Citation46). The finer, spherical and high surface-area-to-volume ratio of nanoparticles to interact with flexible and elastic cell walls of pathogens creates a better antimicrobial activity ( Citation10).
Figure 7. Comparative Disk diffusion antibacterial assay of P. sylvestris L. seed extracts and Green-synthesized AgNPs and Bulk AgNO3 against (A) P. acnes, (B) S. epidermidis and (C) Standard.
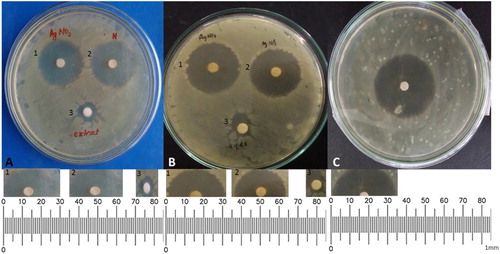
Table 3. Disk diffusion antibacterial assay of P. sylvestris L. seed extracts and green-synthesized AgNPs against acne vulgaris bacterial pathogens.
4. Conclusion and future prospects
AgNPs have been efficiently synthesized from the seed extract of P. sylvestris. Although the mechanism by which the seed extract was accountable for the synthesis of NPs is still obscure. However, it is acceptable to conclude from the literature and Phenolic and flavonoid assay that higher total phenolic content of extracts affirms the assembly of silver ions into finer AgNPs because of the donation of electrons by these compounds. The synthesized NPs and seed extracts possess high free radical scavenging activity can be concluded as good antioxidant agents against the pathogens causing acne vulgaris. The AgNPs also shows high antibacterial activity with high time-kill kinetics against both the pathogens. The data obtained from the study suggest the potential use of AgNPs synthesized by seed extract of P. sylvestris in the cosmeceuticals for the treatment of Acne vulgaris as it is proved effective antioxidant and antibacterial agents. Because of the unique optical, physical and antimicrobial properties, AgNPs would find widespread uses in the medical and other sectors, such as would healing, food sanitation and drug delivery. Further biomedical desired properties can be obtained by preparing a composite using AgNPs as reinforcement into a polymer matrix. Future investigations should be focused on the use of AgNPs to aim at designing an effective tool for drug delivery, diagnosis and treatment of fatal diseases besides ensuring higher safety and efficacy.
Acknowledgements
The authors express their gratitude to Head, Department of Botany, and University of Allahabad for providing research facilities, UGC, New Delhi for financial support, Mr Rick Z for providing anaerobic jar and Moti Lal Nehru Medical College, Allahabad for providing the anaerobic culturing facilities. Dr Dwivedi, Centre for Petrology and Mineralogy A.U, MNIT, Allahabad is acknowledged for providing characterization facilities and staffs at the AIIMS, New-Delhi, India are also acknowledged for providing TEM facilities.
Disclosure statement
No potential conflict of interest was reported by the authors.
Notes on contributors
Afifa Qidwai has completed her research (D.Phil) from Department of Botany, University of Allahabad, and has expertise in culturing and clinical isolation of microbes and formulation of drugs and expert in handling instruments like CO2 Incubator, Anoxomate advance instrument, Rotary Evaporator, Clevenger Apparatus, Spectramax (384 plus), RT PCR, UV–vis Spectrophotometer, Shaker Incubator, Ultrasonicator, etc. He has 9 publications, 3 book chapter and 1 award in her credit.
Rajesh Kumar is currently working as a Senior Research Fellow (upgraded from CSIR-JRF), in the Biological Product Laboratory Department of Botany, University of Allahabad, India, under the supervision of Professor Anupam Dikshit FNASc on the topic “Studies on Controlled Delivery of Plant based Antimicrobials using Nanomaterials”, he has expertise in culturing and isolation of genetic material of microbes and in handling of instruments like rotary evaporator, Clevenger apparatus, Spectramax (384 plus), reverse transcription polymerase chain reaction (RT PCR), Uv–Vis spectrophotometer, shaker incubator, ultrasonicator, etc. He has three publications to his credit including one book, one book chapter and one journal paper.
Anupam Dikshit, FNASc, Head, Department of Botany, Co-ordinator Environmental Sciences, University of Allahabad, India, has a number of international patents and awards for developing various biological products for agricultural and human health after visiting the UK, USA and Germany. He is currently using molecular, nanotechnological and bioinformatical tools. In addition, he has a visiting Professorship in Sri Sathya Sai Institute of Higher Learning (SSSIHL), Prasanthi Nilayam and Visva Bharati, Santiniketan. He has delivered lectures as a Resource person in different states and Central Universities of India. Professor Dikshit has more than 100 publications in national and international journals. He has guided one DSc and 19 DPhil students.
References
- Dahl, J.A.; Maddux, B.L.S.; Hutchinson, J.E. Toward Greener Nanosynthesis. Chem Rev 2007, 107,2228–2269. doi: 10.1021/cr050943k
- Iravani, S. Green Synthesis of Metal Nanoparticles Using Plants. Green Chem 2011, 13,2638–2650. doi: 10.1039/c1gc15386b
- Ravindran, P.; Fu, J.; Wallen, S.L. Completely Green Synthesis and Stabilisation of Metal Nanoparticles. J Am Chem Soc 2003, 125,13940–13941. doi: 10.1021/ja029267j
- Albrecht, M.A.; Evans, C.W.; Raston, C.L. Green Chemistry and the Health Implications of Nanoparticles. Green Chem 2006, 8,417–432. doi: 10.1039/b517131h
- Ashok Kumar, S.; Ravi, S.; Kathiravan, V.; Velmurugan, S. Synthesis of Silver Nanoparticles Using A. indicum Leaf Extract and their Antibacterial Activity. Spectrochim Acta Part A: Mol Biomol Spectrosc 2015, 134,34–39. doi: 10.1016/j.saa.2014.05.076
- Nakkala, J.R.; Mata, R.; Gupta, A.K.; Sadras, S.R. Green Synthesis and Characterization of Silver Nanoparticles Using Boerhaavia diffusa Plant Extract and their Antibacterial Activity. Indus Crop Prod 2014, 52,562–566. doi: 10.1016/j.indcrop.2013.10.050
- Kumar, V.; Yadav, S.K. Synthesis of Stable, Polyshaped Silver and Gold Nanoparticles Using Leaf Extract of Lonicera japonica L. Int J Green Nanotechnol 2011, 3,281–291. doi: 10.1080/19430892.2011.633474
- Tolaymat, T.M.; Badawy, A.M.E.; Genaidy, A.; Scheckel, K.G.; Luxton, T.P.; Suidan, M. An Evidence-based Environmental Perspective of Manufactured Silver Nanoparticle in Synthesis and Applications: A Systematic Review and Critical Appraisal of Peer-reviewed Scientific Papers. Sci Total Environ. 2010, 408, 999–1006. doi: 10.1016/j.scitotenv.2009.11.003
- Alvarez-Puebla, R.A.; Aroca, R.A. Synthesis of Silver Nanoparticles with Controllable Surface Charge and their Application to Surface-enhanced Raman Scattering. Anal Chem 2009, 81,2280–2285. doi: 10.1021/ac8024416
- Agnihotri, S.; Mukherji, S.; Mukherji, S. Size-controlled Silver Nanoparticles Synthesized over the Range 5–100 nm Using the Same Protocol and their Antibacterial Efficacy. RSC Adv 2014, 4,3974–3983. doi: 10.1039/C3RA44507K
- Paralikar, P. Biogenic Synthesis of Silver Nanoparticles Using Leaves Extract of EpiphyllumOxypetalum and its Antibacterial Activity. Austin J Biotechnol Bioeng 2014, 1 (7), 5.
- Ingle, A.; Gade, A.; Pierrat, S.; Sonnichsen, C.; Rai, M. Mycosynthesis of Silver Nanoparticles Using the Fungus fusarium Acuminatum and its Activity Against Some Human Pathogenic Bacteria. CurrNanosci 2008, 4,141–144.
- Rai, M.K.; Deshmukh, S.D.; Ingle, A.P.; Gade, A.K. Silver Nanoparticles: the Powerful Nanoweapon Against Multidrug-Resistant Bacteria. J ApplMicrobiol. 2012, 112,841–852.
- Cogen, A.L.; Nizet, V.; Gallo, R.L. Skin Microbiota: A Source of Disease or Defence? Br. J. Dermatol. 2008, 158,442–455. doi: 10.1111/j.1365-2133.2008.08437.x
- Purchiaroni, F.; Tortora, A.; Gabrielli, M.; Bertucci, F.; Gigante, G.; Ianiro, G.; Ojetti, V.; Scarpellini, E.; Gasbarrini, A.;et al. The Role of Intestinal Microbiota and the Immune System. Eur. Rev. Med. Pharmacol. Sci. 2013, 17 (3), 323–333.
- Kim, D.O.; Chun, O.K.; Kim, Y.J.; Moon, H.Y.; Lee, C.Y. Quantification of Polyphenolics and Their Antioxidant Capacity in Fresh Plums. J. Agric. Food Chem 2003, 516,509–6515.
- Bouhlali, E.d.T.; Alem, C.; Ennassir, J.; Benltyas, M.; Mbark, A.N.; Zagzaouti, Y.F. Phytochemical Compositions and Antioxidant Capacity of Three Date (Phoenix dactylifera L.) Seeds Varieties Grown in the South East Morocco. J Saudi Soc Agr Sci. 2015, 16 (7), 350–357.
- Shen, Q.; Zhang, B.; Xu, R.; Wang, Y.; Ding, X.; Li, P. Antioxidant Activity in Vitro of Selenium-Contained Protein from the SE-enriched Bifidobacteriumanimalis 01. Anaerobe 2010, 16 (4), 380–386. doi: 10.1016/j.anaerobe.2010.06.006
- Boly, R.; Lamkami, T.; Lompo, M.; Dubois, J.; Guissou, I.P. DPPH Free Radical Scavenging Activity of Two Extracts from Agelanthusdodoneifolius (Loranthaceae) Leaves. Int J Toxicol Pharmacol Res 2016, 8 (1), 29–34.
- Pandey, M.; Qidwai, A.; Kumar, R.; Pandey, A.; Shukla, S.K.; Pathak, A.; Dikshit, A. Pharmacological and Antibacterial Aspect of Psidium guajava L. Against Acne Vulgaris. IJPSR 2017, 8 (1), 145–150.
- Qidwai, A.; Pandey, M.; Shukla, S.K.; Kumar, R.; Pandey, A.; Dikshit, A. Antibacterial Activity of Mentha piperita and Citrus limetta against Propionibacterium acnes (Anaerobic bacteria).IJPSR 2016, 7 (7), 2917–2924.
- Aureli, P.; Costantini, A.; Zolea, S. Antimicrobial Activity of Some Plant Essential Oils against Listeria Monocytogenes. J Food Prot 1992, 55,344–348. doi: 10.4315/0362-028X-55.5.344
- Ponarulselvam, S.; Panneerselvam, C.; Murugan, K.; Aarthi, N.; Kalimuthu, K.; Thangamani, S. Synthesis of Silver Nanoparticles using Leaves of Catharanthus roseus Linn. G. Don and their Antiplasmodial Activities. Asian Pac J Trop Biomed 2012, 2 (7), 574–580. doi:10.1016/S2221-1691(12)60100-2.
- Kalaiselvi, A.; Roopan, S.M.; Madhumitha, G.; Ramalingam, C.; Elango, G. Synthesis and Characterization of Palladium Nanoparticles Using Catharanthus roseus Leaf Extract and its Application in the Photo-Catalytic Degradation. Spectrochim Acta A Mol Biomol Spectrosc 2015, 135,116–119. doi: 10.1016/j.saa.2014.07.010
- Ahmad, A.; Mukherjee, P.; Senapati, S.; Mandal, D.; Khan, M.I.; Kumar, R. Extracellular Biosynthesis of Silver Nanoparticles Using the Fungus fusarium Oxysporum. Coll Surf B Biointerfaces 2003, 28,313–318. doi: 10.1016/S0927-7765(02)00174-1
- Wang, J.; Wen, L.; Wang, Z.; Chen, J. Immobilization of Silver on Hollow Silica Nanospheres and Nanotubes and their Antibacterial Effects. Mater Chem Phys 2006, 96, 90–97. doi: 10.1016/j.matchemphys.2005.06.045
- Gengan, R.M.; Anand, K.; Phulukdaree, A.; Chuturgoon, A. A549 Lung Cell Line Activity of Biosynthesized Silver Nanoparticles Using Albiziaadianthifolia Leaf. Colloids Surf B Biointerf 2013, 105,87–91. doi: 10.1016/j.colsurfb.2012.12.044
- Yugandhar, P.; Haribabu, R.; Savithramma, N. Synthesis, Characterization and Antimicrobial Properties of Green-Synthesised Silver Nanoparticles From Stem Bark Extract of Syzygium Alternifolium (Wt.) Walp.3. Biotech 2015, 5 (6), 1031–1039.
- Singh, A.; Singh, N.B.; Hussain, I.; Singh, H.; Yadav, V.; Singh, S.C. Green Synthesis of Nano Zinc Oxide and Evaluation of its Impact Ongermination and Metabolic Activity of Solanum lycopersicum. J Biotechnol 2016, 233,84–94. doi: 10.1016/j.jbiotec.2016.07.010
- Ramalingam, V.; Rajaram, R.; Premkumar, C.; Santhanam, C.; Dhinesh, P.; Vinothkumar, S.; Kaleshkumar, K. Biosynthesis of Silver Nanoparticles From Deepsea Bacterium Pseudomonas Aeruginosa JQ989348 for Antimicrobial, Antibioflim and Cytotoxic Activity. J Basic Microbiol 2014, 54,928–936. doi: 10.1002/jobm.201300514
- Muthukrishnan, S.; Bhakya, S.; Kumar, T.S.; Rao, M.V. Biosynthesis, Characterization and Antibacterial Effect of Plant-Mediated Silver Nanoparticles Using Ceropegiathwaitesii – An Endemic Species. Ind Crops Prod 2015, 63,119–124. doi: 10.1016/j.indcrop.2014.10.022
- Sharma, B.; Purkayastha, D.D.; Hazra, S.; Thajamanbi, M.; Bhattacharjee, C.R.; Ghosh, N.N.; Rout, J. Biosynthesis of Fluorescent Gold Nanoparticles Using an Edible Freshwater red Alga, Lemaneafluviatilis (L.) C. Ag. and Antioxidant Activity of Biomatrix Loaded Nanoparticles. Bioprocess Biosyst Eng 2014, 37 (12), 2559–2565. doi: 10.1007/s00449-014-1233-2
- Kalyanasundaram, G.T.; Doble, M.; Gummadi, S.N. Production and Downstream Processing of (1 → 3)-β-D-Glucan from Mutant Strain of Agrobacterium sp. ATCC 31750. AMB Express 2012, 2 (1), 31. doi: 10.1186/2191-0855-2-31
- Quaresma, P.; Soares, L.; Contar, L.; Miranda, A.; Osorio, I.; Carvalho, P.A.; Franco, R.; pereira, E. Green Photocatalytic Synthesis of Stable Au and Ag Nanoparticles. Green Chem Commun. 2009, 11, 1889–1893. doi: 10.1039/b917203n
- Awika, J.M.; Rooney, L.W.; Wu, X.; Prior, R.L.; Cisneros-Zevallos, L. Screening Methods to Measure Antioxidant Activity of Sorghum (Sorghum Bicolor) and Sorghum Products. J Agric Food Chem 2003, 51,6657–6662. doi: 10.1021/jf034790i
- Chang, S.T.; Wu, J.H.; Wang, S.Y.; Kang, P.L.; Yang, N.S.; Shyur, L.F. Antioxidant Activity of Extracts From Acacia Confuse Bark and Heartwood. J Agric Food Chem 2001, 49,3420–3424. doi: 10.1021/jf0100907
- Marcinkiewicz, J.; Biedroń, R.; Białecka, A.; Kasprowicz, A.; Mak, M.; Targosz, M. Susceptibility of Propionibacterium acnes and Staphylococcus epidermidis to Killing by MPO-Halide System Products.Implication for Taurinebromamine as a new Candidate for Topical Therapy in Treating Acne Vulgaris. Arch Immunol Ther Exp 2006, 54,61–68. doi: 10.1007/s00005-006-0007-1
- Burkhart, C.G.; Burkhart, C.N.; Lehmann, P.F. Acne: A Review of Immunologic and Microbiologic Factors. Postgrad Med J 1999, 75,328–331. doi: 10.1136/pgmj.75.884.328
- Jeremy, A.H.; Holland, D.B.; Roberts, S.G.; Thomson, K.F.; Cunliffe, W.J. Inflammatory Events are Involved in Acne Lesion Initiation. J. Invest. Dermatol 2003, 121,20–27. doi: 10.1046/j.1523-1747.2003.12321.x
- Koreck, A.; Pivarcsi, A.; Dobozy, A.; Kemeny, L. The Role of Innate Immunity in the Pathogenesis of Acne. Dermatology 2003, 206,96–105. doi: 10.1159/000068476
- Wang, L.; Li, H.; Tian, J.; Sun, X. Monodisperse, Micrometer-Scale, Highly Crystalline, Nanotextured Ag Dendrites: Rapid, Large-Scale, wet-Chemical Synthesis and their Application as SERS Substrates. ACS Appl Mater Interfaces 2010, 2,2987–2991. doi: 10.1021/am100968j
- Paszek, E.; Czyz, J.; Woźnicka, O.; Jakubiak, D.; Wojnarowicz, J.; Łojkowski, W.; Stępień, E. Zinc Oxide Nanoparticles Impair the Integrity of Human Umbilical Vein Endothelial Cell Monolayer in Vitro. J Biomed Nanotechnol 2012, 8 (6), 957–967. doi: 10.1166/jbn.2012.1463
- Baker, C.; Pradhan, A.; Pakstis, L.; Pochan, D.J.; Shah, S.I. Synthesis and Antibacterial Properties of Silver Nanoparticles. J Nanosci Nanotechnol 2005, 5,244–249. doi: 10.1166/jnn.2005.034
- Kumar, R.; Shukla, S.K.; Pandey, A.; Srivastava, S.K.; Dikshit, A. Copper Oxide Nanoparticles: An Antidermatophytic Agent for Trichophyton spp. Nanotechnol Rev 2015, 4,401–409.
- Sondi, I.; Sondi, B.S. Silver Nanoparticles as Antimicrobial Agent: a Case Study on E. Coli as a Model for Gram-Negative Bacteria. J Colloid Interface Sci 2004, 275,177–182. doi: 10.1016/j.jcis.2004.02.012
- Shankar, S.S.; Rai, A.; Ahmad, A.; Sastry, M. Controlling the Optical Properties of Lemongrass Extract Synthesized Gold Nanotriangles and Potential Application in Infrared-Absorbing Optical Coatings. Chem Mater 2005, 17, 566–572. doi: 10.1021/cm048292g