ABSTRACT
Sodium dodecyl benzene sulfonate was successfully used for the tandem Knoevenagel-Michael reaction of aromatic aldehydes with 1-phenyl-3-methyl-5-pyrazolone in water to synthesis of 4, 4′-arkylmethylene-bis(1-phenyl-3-methyl-5-pyrazolones) under mild reaction conditions. Efficient catalytic activity and high selectivity of the catalyst contributed to colloidal particle formation in water. The shape and size of the colloidal aggregates were confirmed by optical microscopy and dynamic light scattering.
GRAPHICAL ABSTRACT
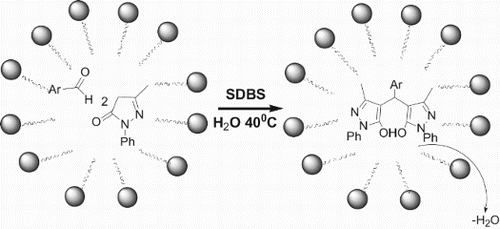
1. Introduction
Most organic reactions need organic solvents as reaction media, the organic solvents have caused environmental pollution. Compared with organic solvents, water is an eco-friendly and cheap solvent for organic reaction ( Citation1). However, the use of water as a solvent is restricted in both industrial and academic researches, because of insolubility of most organic substances in water. Lately, surfactants can make organic substance soluble in water by colloidal particles formation. Therefore, it can overcome the drawback of the organic transforms in water. For example, Kobayashi and co-workers showed that esterification, etherification, thioethrification proceeded smoothly in water containing colloidal particles formed by the surfactant dodecyl benzene sulfonic acid (DBSA) ( Citation2). In recent decades, surfactant-type catalysts have received special interest for organic reactions, because they play a dual role: both as a catalyst to activate the reactant molecules and as a surfactant to form micellar aggregates in order to increase the reaction rate or improve the reaction regio- and chemo selectivity ( Citation3). Moreover, on completion of the reaction, surfactants are removed by using water without using any organic solvent ( Citation4).
3-methyl-5-pyrazolone derivatives including 4,4′-arkylmethylene-bis(1-phenyl-3-methyl-5-pyrazolones) have a broad spectrum of approved biological activity, bearing with antiviral ( Citation5), anti-inflammatory ( Citation6), and antibacterial effect ( Citation7). In addition, the corresponding 4,4′-arkylmethylene-bis(1-phenyl-3-methyl-5-pyrazolones) was used as analytical reagents, textile dyes ( Citation8), and as ligands for extracting different metal elements ( Citation9). The conventional synthesis of 4,4′-arkylmethylene-bis(1-phenyl-3-methyl-5-pyrazolones) involves tandem Knoevenagel-Michael reaction for aromatic aldehydes with two equivalent of 1-phenyl-3-methyl-5-pyrazolone, which can be performed under a variety of reaction conditions ( Citation10, Citation11). In recent years, a wide array of catalysts, such as sodium dodecyl sulfate ( Citation12), eletrocatalytic procedure ( Citation13), and silica-bonded S-sulfonic acid ( Citation14), phosphomolybdic acid ( Citation15), 3-aminopropylated silica gel ( Citation16), 1,3-disulfonic acid imidazolium tetrachloroaluminate {[Dsim]AlCl4} ( Citation17) were employed to synthesize 4,4′-arkylmethylene-bis(1-phenyl-3-methyl-5-pyrazolones). Recently, the synthetic protocol for the 4-substituted pyrazolone derivatives was developed via the one-pot condensation of phenyl hydrazine, ethyl acetoacetate, and aldehydes in the presence of Na+-MMT-[pmim]HSO4 ( Citation18) or 2-hydroxy ethylammonium propionate ( Citation19). However, some of the methods suffer from long reaction time, harsh reaction conditions, or use of expensive catalysts and organic solvents. In view of the formed colloidal particles in water as an effective, environmentally benign method for organic synthesis and in continuation of our work in the green organic synthesis ( Citation20), we investigated the synthesis of 4,4′-phenylmethylene-bis(1-phenyl-3-methyl-5-pyrazolones) with 1-phenyl-3-methyl-5-pyrazolone and benzaldehyde using different surfactants in aqueous medium, and found that sodium dodecyl benzene sulfonate (SDBS) was a most effective catalyst. Consequently, the reaction conditions of the desired product were explored, the results demonstrated that 1:2 molar ratio of benzaldehyde and 1-phenyl-3-methyl-5-pyrazolones reacted for 3 h at about 40°C, in the presence of SDBS as an organocatalyst, which can make organic substance to form colloidal particles in water, and the desired product can be obtained with a 93.0% yield, Then, the catalytic mechanism of SDBS was proposed.
2. Results and discussion
2.1. Reaction condition optimization
To evaluate the catalytic activity of the surfactant, the condensation between 1-phenyl-3-methyl-5-pyrazolone (1) and benzaldehyde (2) was selected as a simple model substrate in water using various type of surfactant (ionic and non-ionic), and optimization results are shown in .
Table 1. Optimization of reaction conditions with surfactants.a
In order to investigate the effects of different surfactants, dodecyl trimethyl ammonium bromide (DTAB), cetyltrimethyl ammonium bromide (CTAB) and SDBS, p-DBSA, polyoxyethylene(20) sorbitan monooleate (Tween80) were separately employed for the model reaction at ambient temperature in water, the results were shown in . In the absence of a surfactant, the low yield (63.0%) was only obtained after 3 h (, entry 22). However, in the presence of a different surfactant, good results were observed. For example, CTAB and Tween80 afforded 78.5% and 77.2% yields, respectively, the yield showed obviously increase for DBSA (80.0%) and DTAB (81.9%), the most dramatic improvement was obtained when the surfactant was switched to SDBS (87.0%) (, entries 1–5).Thus, SDBS was the most effective surfactant for the synthesis of 4,4′-arkylmethylene-bis(1-phenyl-3-methyl-5-pyrazolones) in water.
In order to optimize the reaction conditions for improving the yield of the desired product, the model reaction was checked in different amounts of SDBS (entries 6–10) at 33°C. The best result was observed when 15 mol% of SDBS was applied in the reaction, the desired product was obviously produced in the high yield (90.0%), when the amounts of SDBS was from 2.5% to 5 mol%, the yield increase abruptly from 80.7% to 89.0%, respectively. Thus, 5 mol% SDBS was chosen for further reaction condition optimization (, entry 7), because no considerable increase was found in yield from 7.5 to 15 mol% of SDBS. Along with the SDBS amount effect on yield, the effect of temperature was experimented by conducting reaction from 10°C, 33°C, and 40°C to 70°C and 90°C, the yield was 70.0%, 91.8%, 93.0%, 78.0%, 67.0%, respectively (entries 11–15). When the temperature was further increased to 100°C (at the reflux condition), the yield further decreased to 64.3% (entry 16). The best yield was obtained at room temperature (rt) (40°C). It seems that a disruption of micelles at higher temperature might decrease the rate of reaction and probably effective collisions may not occur because of the higher kinetic energy of the reactants. Similarly, the reaction time was checked (entries 17–20), 3 h of reaction time was selected as the effective time. Then, the molar ratio of 1-phenyl-3-methyl-5-pyrazolone (1) and benzaldehyde(2) was tested on the basis of the research conditions. The results indicated that an excellent yield of 91.8% was obtained, when the optimum molar ratio was 1:2 (entry 11and 7). Thus, on the optimized reaction conditions, the desired product was obtained with a 93.0% yield (entry 13).
2.2. Catalytic mechanism analysis
It is pertinent to mention that the addition of SDBS converted the initially floating reactants in water into homogenous mixture, which formed a turbid emulsion with magnetic stirring ((A,B)), when the reaction proceeded for 2 h, red precipitate appeared from the reaction mixture and the reaction mixture had been homogenous all the time in the presence of SDBS, comparison with no surfactant ((C,D)).
Figure 1. The mixture of the reactants (A) without surfactant; (B) with SDBS; (C) without surfactant after 2 h; (D) with SDBS after 2 h.
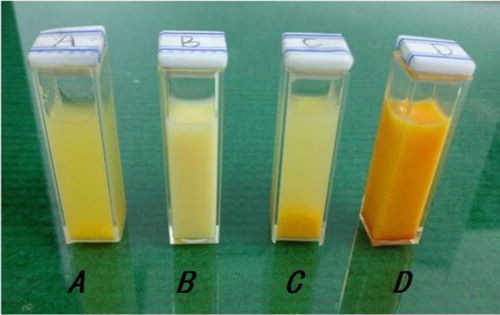
The above-mentioned observations indicate that there is a formation of micelles or micelle-like colloidal aggregates, the formation of emulsion droplets stabilized by SDBS in water was confirmed by optical microscopy (), the shape of emulsion droplets are mostly spherical, the sizes and size distributions of the colloidal particles formed by SDBS and the reaction mixture in water were also measured by dynamic light scattering (DLS), shows the sizes and size distributions of the colloidal aggregates was in range of 330–1600 nm in diameter ().
Figure 3. Size and size distribution of the colloidal particles formed by SDBS in the reaction system.
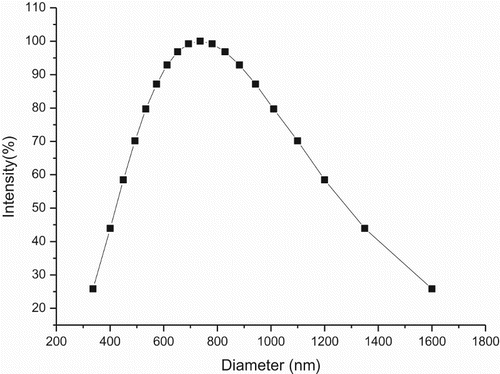
Keeping the above-mentioned fact in mind, we hypothesized the mechanism of the condensation reaction as depicted in . Firstly, on SDBS dissolution in water, the hydrophobic chain of SDBS is located away from the aqueous region and the hydrophilic head is arrayed around the aqueous peripheral. Because of hydrophobic interactions of SDBS with reactants, droplets with hydrophobic interior were formed in emulsion ( Citation21).
Scheme 1. Micelle-promoted synthesis of 4,4′-arylmethylene bis(1-phenyl-3-methyl-5-pyrazolones) in water.
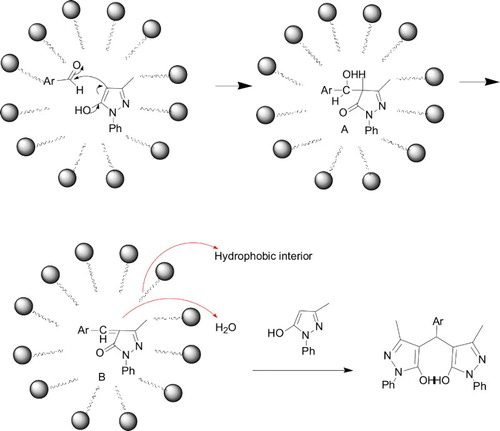
An aromatic aldehyde and1-phenyl-3-methyl-5-pyrazolone stayed within the droplets due to their hydrophobic nature. In the interior of the droplets, hydrogen atom of the hydroxyl group which exists in the enol form of 1-phenyl-3-methyl-5-pyrazolone combined with oxygen atom of carbonyl group in the aromatic aldehyde. Consequently, 4-carbon of the pyrazolone attacked the carbon (carbonyl group), the intermediate A was generated through the nucleophilic addition. Hydroxyl group in the benzyl group of A and hydrogen atom of 4-carbon in the pyrazolone generated water and intermediate B via the eliminate reaction. The dehydrated α,β-unsaturated ketone stayed in the internal of droplets, and the generated water was expelled to the external of the droplets due to its hydrophobic nature ( Citation22), the α,β-unsaturated ketone and another molecular of pyrazolone proceeded through Michael addition in the internal of droplets to produce the 4,4′-arkylmethylene-bis(1-phenyl-3-methyl-5-pyrazolones) in the reaction system. Furthermore, the aromatic aldehyde and 1-phenyl-3-methyl-5-pyrazolone were further concentrated within the droplets, the generated water molecules might have been removed from the droplets, such molecular reorientations caused effective collisions and enhanced a reaction rate in an aqueous medium, and shortened time of attaining equilibrium with an increase of collisions via a concentration effect ( Citation23). More importantly, we found that condensation product (α,β-unsaturated ketone), which generated in the starting of reaction, is easy to transform the Michael addition compound, even if the reaction proceeded by equal molar reactants. The reactions did not stay the stage of condensation product, but generated the addition compound, the reaction stabilized by SDBS in water is obviously different from that helped by microwave ( Citation3, Citation24), which demonstrated that the reaction in the colloid particles helped to improve the selectivity of the reaction.
2.3. Catalytic performance of SDBS for other aromatic aldehydes
To assess the efficiency of SDBS, differential aromatic aldehydes were reacted with 1-phenyl-3-methyl-5-pyrazolone under the optimized reaction using SDBS as a surfactant. The corresponding results are summarized in .
Table 2. SDBS catalyzed synthesis of 4,4′-arkylmethylene-bis(1-phenyl-3-methyl-5-pyrazolones) in water.
As shown in , the influence of electron and the nature of substituents on the aromatic ring did not display obvious effects on the yield under the above optimized conditions. Benzaldehyde and other aromatic aldehydes containing electron-withdrawing group (such as nitro group) and electron-donating group (such as methyl group) give the corresponding products in excellent yields, suggesting the optimized model is suitable to most aromatic aldehydes. It is noteworthy that the colloidal catalysis method is also suitable to heteroaromatic aldehydes (, entries 10–12). Because of the neutral nature of SDBS, acid-sensitive aldehydes (furfural) as well as basic aldehydes (2-pyridinecarbaldehyde) led to the desired products in good yields. As an extension, we found utilizing 3-methyl-5-pyrazole instead of 1-phenyl-3-methyl-5-pyrazole reacted with benzaldehyde, p-chlorobenzaldehyde, and p-methylbenzaldehyde in this transformation were converted into the corresponding products in 82.2%, 87.8% and 80.3%, respectively (the corresponding products were confirmed by comparison of melting points with those of authentic compounds ( Citation26)).
2.4. Spectra data for selected products
4,4′-[(Phenyl)methylene]bis(1-phenyl-3-methyl-5-pyrazolones) (, entry 1) (3a). IR (KBr,cm−1): 3342, 3085, 2910, 1577, 1489, 1412, 1267, 1187, 731, 688; 1H NMR (400 MHz, DMSO-d6):δ (ppm) 2.31 (s, 6H, 2CH3), 4.90 (s, 1H, CH), 7.08–7.16 (m, 5H, Ph-H), 7.24 (t, 2H, J = 7.8 Hz, Ph-H), 7.42 (t, 4H, J = 8.1 Hz, Ph-H), 7.71 (d, 4H, J = 8.0 Hz, Ph-H), 12.41 (br, 1H, OH), 13.92 (s, 1H, OH); 13C NMR (100 MHz, DMSO-d6): δ (ppm) 12.10, 21.00, 33.23, 120.96, 125.98, 127.55, 129.15, 129.38, 135.28, 139.61, 146.71; HRMS: calcd. For C27H24N4O2: [M–H]+:m/z: 435.1891, found: 435.1826.
4,4′-[(4-Nitrophenyl)methylene]bis(1-phenyl-3-methyl-5-pyrazolones) (, entry 2) (3b). IR (KBr, cm−1): 2923, 1593, 1499, 1342, 1104, 756, 740. 1H NMR (400 MHz, DMSO-d6): δ (ppm) 2.32 (s, 6H, 2CH3), 4.96 (s, 1H, CH), 7.05–7.14 (t, 2H, J = 8.4 Hz, Ph-H), 7.20–7.32 (m, 4H, Ph-H), 7.40–7.44 (t, 2H, J = 7.6 Hz, Ph-H), 7.70–7.72 (d, 2H, J = 8.4 Hz, Ph-H), 12.51 (br, 1H, OH), 13.92(s, 1H, OH); 13CNMR (100 MHz, DMSO-d6): δ (ppm) 12.07, 33.91, 115.09, 121.02, 126.07, 129.39, 129.44, 129.52, 138.66, 146.67, 159.92, 162.32.
4,4′-[(4-Methylphenyl)methylene]bis(1-phenyl-3methyl-5-pyrazolones) (, entry 3) (3c).1HNMR (400 MHz, DMSO-d6): δ (ppm) 1.08 (s, 3H, CH3), 2.30 (s, 6H, 2CH3), 4.85 (s, 1H, CH), 6.66 (d, 2H, J = 8.4 Hz, Ph-H), 7.05 (d, 2H, J = 8.4 Hz, Ph-H), 7.24 (t, 2H, J = 7.6 Hz, Ph-H), 7.44 (t, 4H, J = 7.6 Hz, Ph-H), 7.70 (d, 4H, J = 8.0 Hz, Ph-H), 9.22 (s, 1H, OH), 13.96 (s, 1H, OH); 13CNMR (100 MHz, DMSO-d6) 12.07, 18.98, 32.84, 56.53, 115.33, 120.97, 126.02, 128.57, 129.39, 132.76, 137.77, 146.68, 155.95.
4,4′-[(2-Methyoxyphenyl)methylene]bis(1-phenyl-3-methyl-5-pyrazolones) (, entry6) (3f). 1HNMR (400 MHz, DMSO-d6): δ (ppm) 2.33 (s, 6H, 2CH3), 3.82 (s, 3H, OCH3), 5.19 (s, 1H, Ph-H), 6.85 (m, 2H, Ph-H), 7.11–7.24 (m, 4H, Ph-H), 7.44 (t, 4H, J = 7.8 Hz, Ph-H), 7.71 (d, 4H, J = 7.8 Hz, Ph-H), 12.45 (s, 1H, OH), 14.43 (s, 1H, OH).
4,4′-[(4-Chlorophenyl)methylene]bis(1-phenyl-3-methyl-5-pyrazolones) (, entry 8) (3h).1HNMR (400 MHz, DMSO-d6): δ (ppm) 2.29 (s, 6H, 2CH3), 5.15 (s, 1H, CH), 7.21–7.32 (m, 4H, Ph-H), 7.39–7.41 (m, 5H, Ph-H), 7.71 (d, 4H, J = 8.0 Hz, Ph-H), 7.81 (m, 1H, Ph-H), 12.60 (br, 1H, OH), 13.91 (s, 1H, OH); 13CNMR (100 MHz, DMSO-d6): δ (ppm) 12.34, 32.12, 121.12, 126.11, 127.39, 128.53, 129.40, 129.93, 130.70, 132.34, 139.94.
4,4′-[(2-Pyridyl)methylene]bis(1-phenyl-3-methyl-5-pyrazolones) (, entry 10) (3j). 1HNMR (400 MHz, DMSO-d6): δ (ppm) 2.28 (s, 6H, 2CH3), 5.10 (s, 1H, CH), 7.23 (t, 2H, J = 7.5 Hz, Ar-H), 7.43 (m, 6H, Ar-H), 7.72 (m, 5H, Ar-H), 8.49 (d, 1H, J = 4.8 Hz, Ar-H), 12.36 (br, 1H, OH) 14.28 (s, 1H, OH); 13CNMR (100 MHz, DMSO-d6): δ (ppm) 12.30, 36.95, 120.80, 122.15, 122.42, 125.85, 129.36, 137.70, 147.07, 148.67, 161.65.
4,4′-[(2-Thienyl)methylene]bis(1-phenyl-3-methyl-5-pyrazolones) (, entry 11) (3k). 1HNMR (400 MHz, DMSO-d6): δ (ppm): 2.34 (s, 6H, 2CH3), 5.13 (s, 1H, CH), 6.75–6.77(m, 1H, Ar-H), 6.90–6.92 (m, 1H, Ar-H), 7.24–7.30 (m, 3H, Ar-H), 7.45 (t, 4H, J = 7.8 Hz, Ar-H), 12.51 (br, 1H, OH), 14.02 (br, 1H, OH); 13CNMR (100 MHz, DMSO-d6): δ (ppm) 29.43, 120.58, 124.05, 124.15, 126.75, 128.94, 132.99, 134.13, 147.73
4,4′-[(2-Furyl)methylene]bis(1-phenyl-3-methyl-5-pyrazolones) (, entry 12) (3l). 1HNMR (400 MHz, DMSO-d6): δ (ppm): 2.31 (s, 6H, 2CH3), 4.99 (s, 1H, CH), 6.12–6.14 (m, 1H, Ar-H), 6.34–6.36(m, 1H, Ar-H), 7.25(t, 2H, J = 6.0 Hz, Ar-H), 7.43–7.51 (m, 5H, Ar-H), 7.71(d, 4H, J = 8.0 Hz, Ar-H), 12.46 (br, 1H, OH), 13.85 (s, 1H, OH); 13CNMR (100 MHz, DMSO-d6): δ (ppm) 28.72, 106.11, 109.88, 110.34, 120.43, 120.55, 125.60, 128.91, 131.92, 141.54
4,4′-[(Phenyl)methylene]bis(3-methyl-5-pyrazolones) 1HNMR (400 MHz, DMSO-d6): δ (ppm): 2.30 (s, 6H, 2CH3), 4.99 (s, 1H, CH), 7.24–7.27 (t, 2H, J = 7.2 Hz, Ar-H), 7.43–7.50 (m, 5H, Ar-H), 12.40 (br, 1H, OH), 13.84 (br, 1H, OH). 13CNMR (100 MHz, DMSO-d6): δ (ppm) 29.43, 106.61, 110.84, 121.02, 126.07, 129.40, 142.02, 146.45, 154.58
3. Conclusion
In summary, we have demonstrated a highly efficient, environmentally friendly procedure for the synthesis of 4,4′-arkylmethylene-bis(1-phenyl-3-methyl-5-pyrazolones) by tandem Knoevenagel-Michael reaction of aromatic aldehydes with 1-phenyl-3-methyl-5-pyrazolone catalyzed by SDBS in aqueous media. The advantages of this method are a high yield and selectivity, very mild reaction conditions. The benefits are contributed to colloidal particle formation stabilized by SDBS in water media. Also, the colloidal catalysis could be applied to other organic transformations in the future.
4. Experimental
4.1. Material and methods
The infrared spectra were obtained on a Nicolet 380 spectrometer. The 1HNMR,13CNMR were carried out on a Bruker 400 MHz Avance, melting points were determined using a melting point WRS-1A apparatus in open capillary tubes and are uncorrected, high-resolution spectra were recorded on Agilent 1290-G6538 Q-TOF system. The diameter of the colloidal particles formed by SDBS in the reaction system was collected on a NanoBroke 173Plus particle analyzer using DLS.
4.2. Typical experimental procedure
In a typical experimental, 1 mmol (0.1061 g) benzaldehyde and 5 mol% (0.0174 g) SDBS were added to 10 mL water in round-bottom flask and stirred at appropriate temperature for 5 min, the emulsions in the form of foam was obtained, 2 mmol (0.3480 g) 1-phenyl-3-methyl-5-pyrazolone was added to the solution, the reaction mixture was stirred for an appropriate time. After completion of the reaction, the mixtures were centrifuged to obtain the insoluble crude product, which was purified by recrystallization in 95% ethyl alcohol.
Disclosure statement
No potential conflict of interest was reported by the authors.
Notes on contributors
Yongjun Zheng received his Ph.D. from the Graduate University of Chinese Academy of Science in 2009. He is now a teacher in the Anyang Institute of Technology. His research interest focuses on the area of ionic liquid and micellar catalysis.
Yong ZShaoheng received his Ph.D. from the University of Chinese Academy of Sciences in 2013. He is now a teacher in the Anyang Institute of Technology. His research interest focuses on the area of ionic liquid.
Zhen Wang was obtained his Ph.D. from the Nankai University in 2014. His research focuses on the area of organic chemistry.
Yana Cao is studying in the School of Chemical and Environmental Engineering, Anyang Institute of Technology. He is mainly investigating the organic reaction.
Qian Shao is the professor of the College of Chemical and Environmental Engineering, Shandong University of Science & Technology. Her research interest focuses on novel catalysts.
Zhanhu Guo is the Director of Integrated Composites Laboratory in the Chemical and Biomolecular Engineering Department, University of Tennessee. His research interest focuses on novel catalysts.
Additional information
Funding
References
- Capello, C.; Fischer, U.; Hungerbuhler, K. Green Chem. 2007, 9, 927–934. doi: 10.1039/b617536h
- Manabe, K.; Sun, X.; Kobayashi, S. J. Am. Chem. Soc. 2001, 123, 10101–10102. doi: 10.1021/ja016338q
- Shiri, M.; Zolfigol, M.A. Tetrahedron 2009, 65, 587–598. doi: 10.1016/j.tet.2008.09.085
- Manabe, K.; Mori, Y.; Wakabayashi, T.; Nagayama, S.; Kobayashi, S. J. Am. Chem. Soc. 2000, 122, 7202–7207. doi: 10.1021/ja001420r
- Sujatha, K.; Shanthi, G.; Selvam, N.P.; Manoharan, S.; Perumal, P.T.; Rajendran, M. Bioorg. Med. Chem. Lett. 2009, 19, 4501–4503. doi: 10.1016/j.bmcl.2009.02.113
- Jagrat, M.; Behera, J.; Yabanoglu, S.; Ercan, A.; Ucar, G.; Sinha, B.N.; Sankaran, V.; Basu, A.; Jayaprakash, V. Bioorg. Med. Chem. Lett. 2011, 21, 4296–4300. doi: 10.1016/j.bmcl.2011.05.057
- Bhavanarushi, S.; Kanakaiah, V.; Bharath, G.; Gangagnirao, A.; Vatsala Rani, J. Med. Chem. Res. 2014, 23, 158–167. doi: 10.1007/s00044-013-0623-3
- Garnovskii, A.D.; Uraev, A.I.; Minkin, V.I. ARKIVOC 2004, No. iii, 29–41.
- Sivaprasad, G.; Perumal, P.T.; Prabavathy, V.R.; Mathivanan, N. Bioorg. Med. Chem. Lett. 2006, 16, 6302–6305. doi: 10.1016/j.bmcl.2006.09.019
- Singh, D.; Singh, D. J. Chem. Eng. Data 1984, 29, 355–356. doi: 10.1021/je00037a040
- Das Gupta, A.; Pal, R.; Mallik, A.K. Green Chem. Lett. Rev. 2014, 7, 404–411. doi: 10.1080/17518253.2014.970236
- Wang, W.; Wang, S.X.; Qin, X.Y.; Li, J.T. Synthetic Commun. 2005, 35, 1263–1269. doi: 10.1081/SCC-200054854
- Elinson, M.N.; Dorofeev, A.S.; Nasybullin, R.F.; Nikishin, G.I. Synthesis 2008, 2008, 1933–1937. doi: 10.1055/s-2008-1067079
- Niknam, K.; Saberi, D.; Sadegheyan, M.; Deris, A. Tetrahedron Lett. 2010, 51, 692–694. doi: 10.1016/j.tetlet.2009.11.114
- Phatangare, K.R.; Padalkar, V.S.; Gupta, V.D.; Patil, V.S.; Umape, P.G.; Sekar, N. Synthetic Commun. 2012, 42, 1349–1358. doi: 10.1080/00397911.2010.539759
- Sobhani, S.; Hasaninejad, A.; Maleki, M.F.; Parizi, Z.P. Synthetic Commun. 2012, 42, 2245–2255. doi: 10.1080/00397911.2011.555589
- Khazaei, A.; Zolfigol, M.A.; Moosavi-Zare, A.R.; Asgari, Z.; Shekouhy, M.; Zare, A.; Hasaninejad, A. RSC Adv. 2012, 2, 8010–8013. doi: 10.1039/c2ra20988h
- Shirini, F.; Seddighi, M.; Mazloumi, M.; Makhsous, M.; Abedini, M. J. Mol. Liq. 2015, 208, 291–297. doi: 10.1016/j.molliq.2015.04.027
- Zhou, Z.; Zhang, Y. Green Chem.Lett.Rev. 2014, 7, 18–23. doi: 10.1080/17518253.2014.894142
- Zheng, Y.; Zheng, Y.; Yang, S.; Guo, Z.; Zhang, T.; Song, H.; Shao, Q. Green Chem. Lett. Rev. 2017, 10, 202–209. doi: 10.1080/17518253.2017.1342001
- Manabe, K.; Iimura, S.; Sun, X.; Kobayashi, S. J. Am. Chem. Soc. 2002, 124, 11971–11978. doi: 10.1021/ja026241j
- Bruce, C.D.; Senapati, S.; Berkowitz, M.L.; Perera, L.; Forbes, M.D.E. J. Phys. Chem. B 2002, 106, 10902–10907. doi: 10.1021/jp025872x
- Wang, L.; Jiao, N.; Qiu, J.; Yu, J.; Liu, J.; Guo, F.; Liu, Y. Tetrahedron 2010, 66, 339–343. doi: 10.1016/j.tet.2009.10.091
- Bai, Y.J.; Li, M.; Lu, J.; Wang, Z.J. Chinese J. Org. Chem. 2004. 24(6), 616–620.
- Keshavarz, M.; Vafaei-Nezhad, M. Catal. Lett. 2016, 146, 353–363. doi: 10.1007/s10562-015-1668-3
- Jahanshahi, R.; Akhlaghinia, B. Chem. Pap. 2017, 71, 1351–1364. doi: 10.1007/s11696-016-0127-y
- Tayebi, S.; Baghernejad, M.; Saberi, D.; Niknam, K. Chinese J. Catal. 2011, 32, 1477–1483. doi: 10.1016/S1872-2067(10)60260-4