ABSTRACT
The new synthesized cationic Gemini surfactant (CGS) was tested as a corrosion inhibitor for carbon steel (CS) in 1.0 M hydrochloric acid using weight loss, electrochemical spectroscopy and potentiodynamic polarization. The inhibition efficiency is increased by increasing the CGS concentration and is almost constant within the temperature range studied. The inhibition was explained by adsorption of the CGS molecule on the CS surface. A mixed-type inhibitor is suggested for the inhibitory effects of CGS as revealed by the potentiodynamic polarization technique. The changes in impedance parameters suggested that the adsorption of the inhibitor on the CGS surface led to the formation of protective films. The adsorption of the CGS on the surface of CS obeys Langmuir adsorption isotherm. Thermodynamic and kinetic parameters were calculated and discussed.
GRAPHICAL ABSTRACT
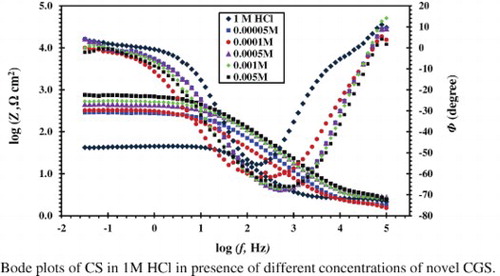
1. Introduction
There are different ways to protect metals and alloys from damage caused by corrosion. One of these methods is applied on the inhibitor to retard the dissolution of CS in acid solutions. The use of organic compounds as additives to acid corrosion is a subject of continual interest to researchers because of many industrial applications, such as acid pickling, sediment removal and oil well cleaning ( Citation1). Thus, many organic compounds containing nitrogen, oxygen, sulfur and/or phosphorus were studied by many researchers ( Citation2–8). They act by adsorption on the steel surface that occurs through heterogeneous atoms, π bonds or aromatic rings. The inhibition efficiency follows the sequence O < N <S < P ( Citation9). The interactions between the organic molecule and the solid surface are primarily physical and/or chemical adsorption. The choice of suitable inhibitors depends mainly on the type, concentration and temperature of the acid.
The acidization of an oil well is an important stimulation technique for enhancing oil production. It is brought about by using a kind of solution of concentrated hydrochloric acid. To reduce aggressive acid attacks on tubing and casing materials, inhibitors were incorporated into the acid solution during the acidizing process ( Citation10). Several previous studies have shown that acidic inhibitors are effective during acidification under high temperature ( Citation11–13). To date, many authors have used surfactant as effective inhibitors for corrosion of iron or steel in acidic media ( Citation14–25).
The aim of this research is to report the inhibition performance of the CGS toward the corrosion of CS in 1 M HCl by weight loss, potentiodynamic polarization and electrochemical impedance techniques. The effect of temperature was studied and some thermodynamic functions were computed and explained.
2. Materials and experimental techniques
2.1. Synthesis of inhibitor
In this study, the inhibitor was synthesized through three steps. In the first step, the quaternization reaction of 1-bromododecane with an appropriate amount of 2-(dimethyl amino) ethanol in the molar ratio of 1:1 was carried out to produce N-(2-hydroxyethyl)-N,N-dimethyl-dodecan-1-aminium bromide ( Citation12). The reactants were allowed to reflux in ethanol for 12 h. Then, the reaction mixture was left to cool at room temperature. The precipitate was further purified by diethyl ether, then crystallized from ethanol. In the second step, the esterification reaction of the synthesized quaternary ammonium salt and citric acid in the presence of toluene as a solvent and p-toluene sulfonic acid as a dehydrating agent ( Citation14) in the molar ratio of 1:2 was carried out to produce N, N'-(4-carboxy-3-hydroxybutanoyl) bis (Oxy)) bis (ethane-2,1-diyl)) bi's (N,N-dimethyl-dodecan-1-aminium) bromide. The reaction was completed when the water was removed from the reaction system. The reaction mixture was distilled under vacuum to completely remove the solvent. In the third step, the product of the second step and polyethylene glycol were esterified as described in the second step in the molar ratio of 1:2 to N-dodecyl-6-(2-(dodecyldimethylammonio) ethoxy)-6,35-dihydroxy-N,N-dimethyl-4,8-dioxo-2,9,12,15,18,21,24,27,30,33-decaoxapentatriacontan-1-aminium) bromide.
The chemical structure of the synthesized inhibitor () was described by FTIR and 1HNMR, spectroscopic analyses.
2.2. Carbon steel (CS)
The chemical composition of the CS sample used in this study is (wt.%): 0.19% C, 0.05% Si, 0.94% Mn, 0.009% P, 0.004% S, 0.014% Ni, 0.009% Cr, 0.034% Al, 0.016% V, 0.003% Ti, 0.022% Cu, and balance Fe.
2.3. Weight loss measurements
The CS specimens with a dimension of 5 cm × 3 cm × 0.5 cm were abraded with a series of emery paper (grade 320–500–800–1000–1200) and washed with distilled water and acetone. After weighing accuracy, the samples were immersed in a closed beaker containing 100 ml of 1.0 M HCl solution with and without different concentrations of the synthesized inhibitor at various temperatures in the range 20–80°C. The temperature was adjusted by water bath supplied with a thermostat control ±1°C. The CS specimens were taken out after 24 h. Then rinsed with distilled water two times and degreased with acetone. Afterward, the specimens were immersed in 1 M HCl solution for 10 s (chemical method for cleaning rust products), rinsed twice with distilled water, dried, and accurately weighted. Each experiment was repeated three times and the average value was taken. Then, the tests were repeated at different temperatures.
2.4. Electrochemical measurements
Potentiodynamic polarization experiments were performed using a conventional three-electrode cell with a platinum counter electrode (CE) and a saturated calomel electrode (SCE) as a reference electrode. The working electrode (WE) was a CS rod embedded in the PVC holder using epoxy resin so that the flat surface was the exposed area of the electrode. Before each measurement, the electrode was immersed in a test solution at an open circuit potential (OCP) for 30 min, until a steady state was reached. The choice of this time is due to the following: when the CS electrode is immersed in the test solution, the OCP is changed rapidly and then after a certain time the value of OCP is constant. This potential is known as the steady-state potential or corrosion potential.
All polarization curves were recorded by a Voltalab 40 Potentiostat PGZ 301 and a personal computer was used with Voltamaster 4 software at 20°C.
Potentiodynamic polarization (PP) measurements were obtained by automatically changing the electrode potential from −800 to −300 mV vs. SCE at open circuit potential with a scan rate of 2 mV s−1 at 20°C. Electrochemical impedance spectroscopy (EIS) measurements were performed as described previously ( Citation15). A small alternating voltage perturbation (5 mV) was imposed on the cell over the frequency range of 100 kHz–30 mHz at 20°C.
3. Results and discussions
3.1. Chemical structure confirmation of the synthesized surfactants
3.1.1. FTIR spectra
FTIR spectra showed that the characteristic bands for the alkyl part were at 2948.86 and 2890.81 cm−1 for asymmetric and symmetric stretching (CH), respectively. But, they were observed at 1334.81 cm−1 for symmetric bending (CH3), at 1438.77 cm−1 for symmetric bending (CH2), and at 725.61 cm−1 for –(CH2)n– rock. C–O stretching band 1240.39.12 cm−1, C–N+ at 1034.04 cm−1, C=O at 1768.08 cm−1, 3437.86 cm−1 was due to stretching OH. FTIR spectra confirmed the expected functional groups in the synthesized CGS compound, as shown in .
3.1.2. 1HNMR spectra
The 1H NMR spectra of the synthesized CGS compound showed different bands at δ = 0.8004–0.8272 ppm (t, 6H, NCH2CH2(CH2)nCH 3); δ = 1.2037 ppm (m, 36H, NCH2CH2(CH2)nCH3); δ = 1.6023 ppm (m, 4H, NCH2CH 2(CH2)nCH3); δ = 2.4672–2.9853 ppm (m,4 H, NCH2OCH 2COCH 2O); δ = 3.30120–3.3569 ppm (m, 32H, CH 3N, NCH2CH2CCO(CH 2CH 2O)nCH 2CH2OH); δ = 3.9773–4.111 ppm (t, 6 H, COO CH 2CH2O(CH2CH2O)nCH2CH 2OH); δ = 4.5795 (s, 4 H, COCH 2); δ = 5.6939–5.7512 (s, 2 H, NCH 2 OCH 2), as shown in .
3.2. EIS measurements
The Bode curves () show only one maximum peak at the phase angle versus the frequency plot. This maximum phase angle can be connected to the double-layer capacitance. The high frequency loop returns to the capacity of the double layer and is parallel to the charge transfer resistance. shows the typical Nyquist diagram of CS in HCl 1 M solution with and without different concentrations of CGS. It is clear that the addition of CGS leads to an increase in the diameter of the semicircle capacitive loop and at the maximum phase angle. Inspections of the data reveal that the Nyquist plot obtained for inhibitor appears to exhibit a depressed loop; such behaviors are mostly referred to as frequency dispersion, indicating irregularities and heterogeneity of solid surfaces ( Citation26).
Figure 5. Nyquist plots for the CS in 1 M HCl in the absence and presence of different concentrations of the novel CGS.
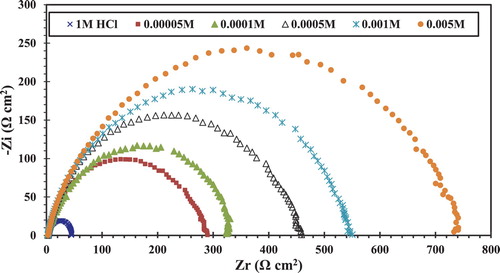
In particular, the high-frequency part of the impedance and phase angle reflects the behavior of heterogeneous surface layer, while the low-frequency part shows the kinetic response to the charge-transfer interaction (
Citation27). In order to obtain more accurate results, the analysis of the complex plane diagram was performed by constructing experimental results in the equivalent circuit shown in , previously used for the CS–acid interface model (
Citation15). The use of a fixed phase element (CPE) can be an effective way of representing the frequency dependence of non-ideal capacitive behavior. The impedance of the CPE is given by (
Citation28)(1) where Q is the constant phase element, ωmax is the angular frequency in rad S−1, ω = 2πfmax (where f is the frequency at which the imaginary component of the impedance is maximum), i is the imaginary number and n is a coefficient that can be used as a measure of surface inhomogeneity. Depending on the value of n, CPE can represent resistance (n = 0, Q = 1/R), capacitance (n = 1, Q = C), inductance (n = −1, Q = 1/L) or Warburg impedance (n = 0.5, Q = W). The values of n were in the range from 0.7 to 0.95, indicating non-ideal capacitive behavior.
Figure 6. The electrical equivalent circuit used for modeling the interface steel/1.0 M HCl solution in the absence and presence of the CGS molecule.
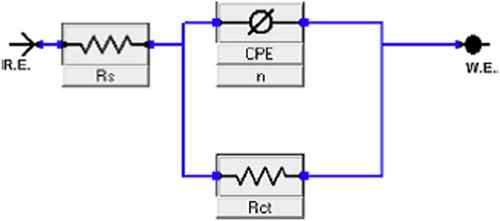
The circuit consists of solution resistance (Rs) in series with a parallel combination of charge-transfer resistance (Rct) and a constant phase element, CPE used in place of double-layer capacitance (Cdl) to represent the non-ideal capacitive behavior of the double layer more clearly. The values of inhibition efficiency of the charge-transfer resistance were calculated from the following equation:
(2) where
and Rct are the charge-transfer resistance values with and without inhibitor, respectively. The results obtained from this complex plot are presented in . The Cdl values were calculated using the following equation (
Citation29):
(3)
From , it is clear that the Rct value of CS in uninhibited solution increases significantly after the addition of the CGS. Also, the addition of the CGS to a corrosive solution reduces the double-layer capacitance. The double layer between the surface of the charged metal and the solution as an electrical capacitor. The reduction of this capacitance can be attributed to the gradual replacement of water molecules by the adsorption of the CGS at the CS–solution interface. The adsorption process followed by the formation of a surface layer film of the inhibitor protects the metal against corrosion ( Citation26). EIS revealed that the corrosion inhibition efficiency of the CGS increases with increasing CGS concentration.
Table 1. EIS parameters for corrosion of CS in 1 M HCl in the absence and presence of different concentrations of the synthesized novel CGS at 20°C.
3.3. PP measurements
shows PP curves for CS in 1 M HCl in the absence and presence of various concentrations of the CGS. shows the values of associated corrosion parameters such as corrosion potential (Ecorr), anodic Tafel slopes (βa), cathodic Tafel slopes (βc), and corrosion current density (icorr). The inhibition efficiency ηp (%) was calculated from PP measurements according to the following relationship (
Citation12):(4) where icorr and icorr(inh) are the corrosion current densities for CS electrode in the uninhibited and inhibited solutions, respectively.
Figure 7. Potentiodynamic polarization curves for the CS in 1 M HCl in the absence and presence of different concentrations of the CGS at scanning rate 2 mV s−1.
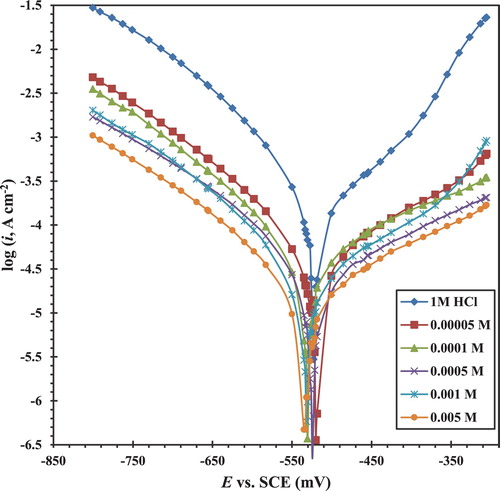
Table 2. Potentiodynamic polarization parameters for CS corrosion in 1 M HCl in the absence and presence of different concentrations of the synthesized novel CGS at 20°C.
shows that the values of anodic and cathodic Tafel constants βa and βc have changed slightly so that the CGS is a mixed type because it reduces the anodic dissolution of CS and retards the cathodic hydrogen evolution reaction. The presence of CGS does not significantly shift the corrosion potential (Ecorr). This indicates that the corrosion mechanism of CS in 1 M HCl solution does not change after adding the inhibitor. This inhibitor is first adsorbed on the CS surface, thus reducing the available surface area for corrosion reactions simply by preventing the interaction sites of the CS surface ( Citation30,Citation31). The values of icorr are reduced with increased CGS concentration, suggesting that the presence of this CGS retards the dissolution of CS in 1 M HCl solution and the degree of inhibition depends on the concentration.
3.4. Weight loss measurement
The corrosion rate (k) and inhibition efficiency were calculated using the following equations (
Citation14,Citation26):
(5)
(6) where ΔW the average weight loss, kfree and kinh the corrosion rates of weight loss of CS in 1 M HCl in the absence and presence of different concentration of the CGS, respectively, A is the surface area in cm2 and t is the time in hours. presents data obtained from weight loss measurements. It was observed that the weight loss was significantly reduced in the presence of the CGS compared to the blank acid solution and found to be dependent on the inhibitor concentration. This suggests that CGS additives retard CS corrosion in 1 M HCl solution, suggesting an increase in inhibition efficiency by increasing concentration.
Table 3. Corrosion rate of CS, surface coverage and percentage inhibition efficiency for CS in 1 M HCl in the absence and presence of different concentrations of novel CGS from weight loss measurements at different temperatures.
3.5. Effect of temperature
Like most chemical reactions, the corrosion rate of CS increases with temperature, especially in acid media due to hydrogen evolution. Acid pickling of CS is usually done at elevated temperatures up to 60°C in HCl. Accordingly, pickling inhibitors are expected to be chemically stable to provide high protective efficiency under the above conditions ( Citation32). An inspection of the values given in , the inhibition efficiency of CGS is almost constant at the studied temperature range ( Citation13). The corrosion rate of CS increases rapidly with temperature in the absence of inhibitor. This finding confirms that the inhibitor acts as an effective inhibitor within the temperature range studied.
3.6. Adsorption isotherm
The adsorption of CGS on the surface of CS can be considered as a substitution process between CGS in the aqueous phase (CGSsoln) and water molecules adsorbed on the surface of the CS (H2Oads) as given in the following equation (
Citation33).(7) where x represents the number of water molecules that are replaced by one molecule of adsorbed CGS. The above process is balanced when the chemical potential in the left hand is equal to that on the right side.
The degree of surface coverage (θ) was determined at a constant temperature of the following equation (
Citation13).(8) where Wfree and Winh are weight losses for CS due to the dissolution in 1 M HCl in the absence and presence of different concentrations of inhibitor, respectively.
Adsorption isotherm is very important in determining the mechanism of electrochemical reactions. I have tried many attempts to find the most appropriate isotherms, for example, Langmuir, Frumkin, Temkin, Flory-Huggins, and Bockris-Swinkels (
Citation34). Langmuir is found to be a suitable isotherm. shows that the Langmuir adsorption isotherm for CS in 1 M HCl is drawn as C/θ vs. C. The straight line is obtained with a slope equal to approximately the unit and the intercept equal to (1/Kads). The experimental data are suitable for the isotherm adsorption of Langmuir, as represented in the following equation (
Citation12):(9) Langmuir isotherm postulates monolayer adsorption, hence no interaction between the adsorbate species on the metal surface.
Figure 8. Langmuir isotherm model for the adsorption of the CGS on the CS surface in 1.0 M HCl at different temperatures.
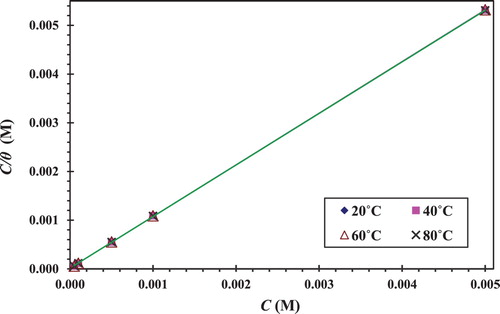
The surface coverage data are clearly useful for discussing the adsorption characteristics. The large the values of Kads the better the adsorption efficiency, thus better the inhibition efficiency (
Citation35). The kinetic adsorption isotherm can be written in the following form (
Citation13,Citation36):(10) or this equation can be written in linear form as
(11)
where K′ is a constant, and y is the number of inhibitory molecules occupying one active site. A plot of ln(θ/1 − θ) versus ln(C) gives a straight line of slope equal y and intercept equal ln(K′), as shown in .
Equilibrium constant corresponding to adsorption isotherm is given by K = K′1/y. The value of y > 1 means a multi-layer formation of the inhibitor on the metal surface. The value of y < 1 means that the inhibitor molecules given will occupy more than one active location.
The values of the equilibrium constants obtained from the Langmuir model were similar to those obtained from the thermodynamic model. Also, the value of y was less than the unit, indicating a monolayer formation on the metallic surface which agrees with the assumptions of the Langmuir adsorption isotherm.
The known thermodynamic adsorption parameters, the free energy of adsorption (), the heat of adsorption (
) and entropy of adsorption (
), were calculated by the mathematical method depending on different temperatures.
The equilibrium constant of the adsorption process from Langmuir () is associated with the standard free energy adsorption
by the following expression:
(12) where R is the molar gas constant, T is the absolute temperature and 55.5 is the concentration of water in solution expressed in mol dm−3.
For, , which can distinguish the interaction between adsorption molecules and metal surface (
Citation37). In general, ΔGads values of up to −20 kJ mol−1 correspond to the electrostatic reaction between charged molecules and charged metal (physical adsorption) while the negative values greater than −40 kJ mol−1 involve the sharing or transfer of electrons from the inhibitor molecules to the metal surface to form a coordinate bond (chemisorption) (
Citation38).
In this study, it was found that the value of is about −40 kJ mol−1, meaning that the adsorption mechanism of CGS on the CS surface is essentially a chemisorption.
The adsorption heat can be calculated according to the Van’t Hoff equation and can also be calculated from the Gibbs–Helmholtz equation, which is defined as follows (
Citation39):(13) which can be arranged to give the following equation:
(14)
The values of the entropy of adsorption were computed using the following equation.
(15)
shows a variation of /T vs. 1/T which gives a straight line with a slope equal to
. The values of the thermodynamic adsorption parameters are listed in . shows that the positive sign of
indicates that CGS adsorption on the CS surface is an endothermic process. The value of
was observed in the measured temperature range indicating that the inhibition of CS corrosion in these solutions was due to the chemical adsorption of the CGS on the CS surface. The positive sign of
indicates that the substitution process leads to an increase in the solvent entropy and more water desorption entropy (
Citation24).
Figure 10. Relationship between /T and 1/T of CS in the absence and presence of different concentrations of the novel CGS in 1 M HCl solution.
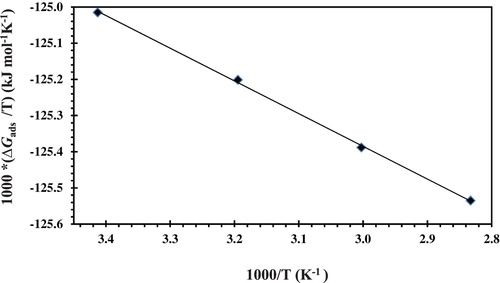
Table 4. Thermodynamic parameters of adsorption on CS surface in 1 M HCl containing different concentrations of the synthesized novel CGS.
Furthermore, the fundamental characteristic of Langmuir isotherm can be expressed in terms of a dimensionless separation factor, RL (
Citation26), which describes isotherm type and defines it by(16)
The smaller RL indicates very favorable adsorption. If RL > 1 is unfavorable, RL = 1 linearly, 0 < RL < 1 is favorable, and if RL = 0 is irreversible. gives the estimated value of RL to inhibitors at different concentrations. The value of the RL value was found to be less than the corresponding unit that the adsorption processes were favorable.
Table 5. Values of dimensionless separation factor, RL and other thermodynamic parameters of adsorption on CS surface in 1 M HCl containing different concentrations of the synthesized novel CGS.
3.7. Inhibition mechanism
The adsorption of the CGS molecule on the surface of the CS covered with primarily adsorbed bromide ions is a two-step process that is consistent with the results mentioned in the literature ( Citation40). Interpretation of the adsorption process depends on the concentration of CGS. First, at low concentrations, the surfactant molecules are distributed on the surface of CS, and it seems that the adsorption of Gemini surfactant molecule takes place by binding of individual Gemini surfactants on charging sites on the CS surface. This adsorption is preferred by the electrostatic interaction between the two ammonium groups (N+) and bromide ions, which is first adsorbed in the CS/solution interface by the electrostatic force of attraction. When the inhibitor concentration is increased due to the strong hydrophobicity of Gemini surfactants, the adsorption is expected to resume at a lower concentration which is the lowest for the most hydrophobic surfactant, but the first step is not yet complete. The driving force of the second adsorption step arises mainly from hydrophobic interactions between surfactant ions adsorbed in the first adsorption step and newly adsorbed ones. This results in the formation of surface micelles.
4. Conclusions
CGS molecule is a good corrosion inhibitor to protect CS during the acidification process.
The inhibition efficiency of CGS depends on its concentration.
CGS acts as a mixed-type inhibitor.
The high inhibition efficiency of the CGS molecule was demonstrated by adsorption on the CS surface and formation of a protective film formation.
The adsorption of the CGS molecule on the surface of CS in 1 M HCl solution follows Langmuir isotherm.
The thermodynamic parameters indicate that the CGS molecule is strongly adsorbed on the CS surface.
Disclosure statement
No potential conflict of interest was reported by the authors.
Notes on contributors
M. Abdallah is professor of physical chemistry. He published more than 130 manuscripts in the international journals. Most publications in the field of corrosion inhibition of some metals and alloys in aqueous solutions using different chemical and electrochemical techniques. He supervisor of 42 Master degree and 22 Ph.D. degree.
M. A. Hegazy is a professor of applied chemistry, Egyptian Petroleum Research Institute, Egypt. He published several papers in corrosion inhibition of metals and alloys using different types of surfactants in international journals. He supervisor of several M.Sc and Ph.D degree.
M. Alfakeer is an assistant professor of physical chemistry, Chemistry Department, Faculty of Science, Princess Nourah bint Abdulrahman University, Riyadh, Saudi Arabia. She has published some manuscripts in the field of corrosion inhibitors.
H. Ahmed is a Ph.D students, Chemistry Department, Faculty of Science, Benha University, Benha, Egypt. He is published some papers in corrosion inhibition of steel in acidic solutions using surfactant compounds.
ORCID
M. Abdallah http://orcid.org/0000-0002-6132-8849
References
- Ozkır, D.; Kayakırılmaz, K.; Bayol, E.; Gurten, A.A.; Kandemirli, F. The Inhibition Effect of Azure A on Mild Steel in 1 M HCl. A Complete Study: Adsorption, Temperature, Duration and Quantum Chemical Aspects. Corros. Sci 2012, 56, 143–152. doi: 10.1016/j.corsci.2011.11.010
- Abdallah, M.; AlTass, H.M.; AlJahdaly, B.A.; Fouda, A.S. Inhibition Properties and Adsorption Behavior of 5-Arylazothiazole Derivatives on 1018 Carbon Steel in 0.5M H2SO4 Solution. J. Mol. Liq. 2016, 216, 590–597. doi: 10.1016/j.molliq.2016.01.077
- Al Jahdaly, B.A.; Althagafi, I.I.; Abdallah, M.; Khairou, K.S.; Ahmed, S.A. Fluorenone Hydrazone Derivatives as Efficient Inhibitors of the Acidic and Pitting Corrosion of Carbon Steel. J. Mater. Environ. Sci. 2016, 7, 1798–1809.
- Fouda, A.S.; Abdallah, M.; Attia, A. Inhibition of Carbon Steel Corrosion by Some Cyanoacetohydrazide Derivatives in HCl Solution. Chem. Eng. Comm. 2010, 197, 1091–1108. doi: 10.1080/00986440903412944
- Fouda, A.S.; Abdallah, M.; Medhat, M. Some Schiff Base Compounds as Inhibitors for Corrosion of Carbon Steel in Acidic Media. Prot. Metals Phys. Chem. Surf. 2012, 48, 477–486. doi: 10.1134/S2070205112040053
- Pandey, A.; Verma, C.; Singh, B.; Ebenso, E.E. Synthesis, Characterization and Corrosion Inhibition Properties of Benzamide–2-Chloro-4-Nitrobenzoic Acid and Anthranilic Acid–2-Chloro-4-Nitrobenzoic Acid for Mild Steel Corrosion in Acidic Medium. J. Mol. Str. 2018, 1155, 110–122. doi: 10.1016/j.molstruc.2017.10.114
- Abdel Hameed, R.S.; Abdallah, M. Corrosion Inhibition of Carbon Steel in 1M Hydrochloric Acid Using Some Pyrazolo[3,4-d]Pyrimidnone Derivatives. Prot. Met. Phys. Chem. Surf. 2018, 54, 113–121. doi: 10.1134/S2070205118010239
- El-Hajjaji, F.; Messali, M.; Aljuhani, A.; Aouad, M.R.; Hammouti, B.; Belghitid, M.E.; Chauhane, D.S.; Quraishi, M.A. Pyridazinium-based Ionic Liquids as Novel and Green Corrosion Inhibitors of Carbon Steel in Acid Medium: Electrochemical and Molecular Dynamics Simulation Studies. J.Mol. Liq. 2018, 249, 997–1008. doi: 10.1016/j.molliq.2017.11.111
- Fouda, A.S.; El-desoky, A.M.; Ead, D.M. Anhydride Derivatives as Corrosion Inhibitors for Carbon Steel in Hydrochloric Acid Solutions. Int. J. Electrochem. Sci. 2013, 8, 8823–8847.
- Abd El–Maksoud, S.A. The Effect of Organic Compounds on the Electrochemical Behaviour of Steel in Acidic Media. A Review. Int. J. Electrochem. Sci. 2008, 3, 528–555.
- Negm, N.A.; Ghuiba, F.M.; Tawfik, S.M. Novel Isoxazolium Cationic Schiff Base Compounds as Corrosion Inhibitors for Carbon Steel in Hydrochloric Acid. Corros. Sci. 2011, 53, 3566–3575. doi: 10.1016/j.corsci.2011.06.029
- Hegazy, M.A.; Abdallah, M.; Ahmed, H. Novel Cationic Gemini Surfactants as Corrosion Inhibitors for Carbon Steel Pipelines. Corros. Sci. 2010, 52, 2897–2904. doi: 10.1016/j.corsci.2010.04.034
- Ben Hmamou, D.; Salghi, R.; Zarrouk, A.; Zarrok, H.; Hammouti, B.; Deyab, S.; El Assyry, A.; Benchat, N.; Bouachrine, M. Electrochemical and Gravimetric Evaluation of 7-methyl-2-phenylimidazo[1,2-á]pyridine of Carbon Steel Corrosion in Phosphoric Acid Solution. Int. J. Electrochem. Sci. 2013, 8, 11526–11545.
- Hegazy, M.A.; Ahmed, H.M.; El-Tabei, A.S. Investigation of the Inhibitive Effect of p-Substituted 4-(N,N,N-Dimethyldodecylammoniu Bromide)Benzylidene Benzene-2-yl-Amine on Corrosion of Carbon Steel Pipelines in Acidic Medium. Corros. Sci. 2011, 53, 671–678. doi: 10.1016/j.corsci.2010.10.004
- Hegazy, M.A.; Abdallah, M.; Awad, M.K.; Rezk, M. Three Novel di- Quaternary-Ammonium Salts as Novel Corrosion Inhibitors for API X65 Steel Pipeline in Acidic Solutions. Part I: Experimental Results. Corros. Sci 2014, 81, 54–64. doi: 10.1016/j.corsci.2013.12.010
- Fawzy, A.; Zaafarany, I.A.; Ali, H.M.; Abdallah, M. New Synthesized Amino Acids-Based Surfactants as Efficient Inhibitors for Corrosion of Mild Steel in Hydrochloric Acid Medium: Kinetics and Thermodynamic Approach. Int. J. Electrochem. Sci. 2018, 13 (5), 4575–4600. doi: 10.20964/2018.05.01
- Abdallah, M.; Al Jahdaly, B.A.; Al-Malyo, O.A. Corrosion Inhibition of Carbon Steel in Hydrochloric Acid Solution Using Non- Ionic Surfactants Derived From Phenol Compounds. Int. J. Electrochem. Sci. 2015, 10, 2740–2754.
- Abdallah, M.; Jahdaly, B.A.A.L.; Sobhi, M.; Ali, A.I. Evaluation of Some Nonionic Surfactants Derived From Hydroquinol Compounds as Corrosion Inhibitors for Carbon Steel in Hydrochloric Acid. Int. J. Electrochem. Sci. 2015, 10 (6), 4482–4494.
- Hazzazi, O.A.; Abdallah, M.; Gad, E.A.M. Inhibition Effect of Some Cationic Surfactants on the Corrosion of Carbon Steel in Sulphuric Acid Solutions: Surface and Structural Properties. Int. J. Electrochem. Sci 2014, 9, 2237–2253.
- Abdallah, M.; Mead, A.I. Pitting Corrosion of an Iron Electrode in HCO- 3 /Cl- Solutions and Its Inhibition by SomeNonionic Surfactants. Ann. Chim. 1993, 83, 424–430.
- Hegazy, M.A.; Abdallah, M.; Alfakeer, M.; Ahmed, M.H. Corrosion Inhibition Performance of a Novel Cationic Surfactant for Protection of Carbon Steel Pipeline in Acidic Media. Int. J. Electrochem. Sci. 2018, 13, 6824–6842. doi: 10.20964/2018.07.53
- Abdallah, M. Corrosion Kinetics of Carbon Steel and Its Inhibition by Ethoxylated Fatty Amines. Ann. Chim. 1994, 84, 529–539.
- Sobhi, M.; El-Sayed, R.; Abdallah, M. Synthesis, Surface Properties and Inhibiting Action of Novel Nonionic Surfactants on C-Steel Corrosion in 1M Hydrochloric Acid Solution. Chem. Eng. Commun 2016, 203 (6), 758–768.
- Abdallah, M.; Hatem, M.E.; Hegazy, M.A.; Ahmed, H. Adsorption and Inhibition Effect of Novel Cationic Surfactant for Pipelines Carbon Steel in Acidic Solution. Prot Met. Phys. Chem. Surf. 2016, 52 (4), 721–730. doi: 10.1134/S207020511604002X
- Sobhi, M.; El-Sayed, R.; Abdallah M, R. The Effect of Non-Ionic Surfactants Containing Triazole, Thiadiazole and Oxadiazole as Inhibitors for the Corrosion of Carbon Steel in 1Mhydrochloric Acid. J. Surf. Detergents 2013, 16, 937–946. doi: 10.1007/s11743-013-1468-y
- Deyab, M.A. Adsorption and Inhibition Effect of Ascorbyl Palmitate on Corrosion of Carbon Steel in Ethanol Blended Gasoline Containing Water as a Contaminant. Corros. Sci. 2014, 80, 359–365. doi: 10.1016/j.corsci.2013.11.056
- Morad, M.S. An Electrochemical Study on the Inhibiting Action of Some Organic Phosphonium Compounds on the Corrosion of Mild Steel in Aerated Acid Solutions. Corros. Sci. 2000, 42, 1307–1326. doi: 10.1016/S0010-938X(99)00138-9
- Martinez, S.; Hukovic, M.M. A Nonlinear Kinetic Model Introduced for the Corrosion Inhibitive Properties of Some Organic Inhibitors. J. Appl. Electrochem. 2003, 33, 1137–1142. doi: 10.1023/B:JACH.0000003851.82985.5e
- Popova, A. Temperature Effect on Mild Steel Corrosion in Acid Media in Presence of Azoles. Corros. Sci. 2007, 49, 2144–2158. doi: 10.1016/j.corsci.2006.10.020
- Torres, V.V.; Rayol, V.A.; Magalhمes, M.; Viana, G.M.; Aguiar, L.C.S.; Machado, S.P.; Orofino, H.; Elia, E.D. Corros. Sci. 2014, 79, 108–118. doi: 10.1016/j.corsci.2013.10.032
- Farag, A.A.; El-Din, M.R.N. The Adsorption and Corrosion Inhibition of Some Nonionic Surfactants on API X65 Steel Surface in Hydrochloric Acid. Corros. Sci. 2012, 64, 174–183. doi: 10.1016/j.corsci.2012.07.016
- Noor, E.A. Temperature Effects on the Corrosion Inhibition of Mild Steel in Acidic Solutions by Aqueous Extract of Fenugreek Leaves. Int. J. Electrochem. Sci. 2007, 2, 996–1017.
- Hegazy, M.A.; Hasan, A.M.; Emara, M.M.; Bakr, M.F.; Youssef, A.H. Evaluating Four Synthesized Schiff Bases as Corrosion Inhibitors on the Carbon Steel in 1 M Hydrochloric Acid. Corros. Sci. 2012, 65, 67–76. doi: 10.1016/j.corsci.2012.08.005
- Abdallah, M.; Al Jahdaly, B.A. Gentamicin, Kanamycin and Amikacin Drugs as non -Toxic Inhibitors for Corrosion of Aluminum in 1.0M Hydrochloric Acid. Int. J. Electrochem. Sci. 2015, 10, 9808–9823.
- Solmaz, R. Investigation of Adsorption and Corrosion Inhibition of Mild Steel in Hydrochloric Acid Solution by 5-(4-Dimethylaminobenzylidene)Rhodanine. Corros. Sci. 2014, 79, 169–176. doi: 10.1016/j.corsci.2013.11.001
- Boumhara, K.; Bentiss, F.; Tabyaoui, M.; Costa, J.; Desjobert, J.M.A.; Bellaouchou, A.; Guenbour, A.; Hammouti, B.; Al-Deyab, S.S. Use of Artemisia Mesatlantica Essential oil as Green Corrosion Inhibitor for Mild Steel in 1 M Hydrochloric Acid Solution. Int. J. Electrochem. Sci. 2014, 9, 1187–1206.
- Obot, I.B.; Egbedi, N.O.; Umoren, S.A. Adsorption Characteristics and Corrosion Inhibitive Properties of Clotrimazole for Aluminium Corrosion in Hydrochloric Acid. Int. J. Electrochem. Sci. 2009, 4, 863–877.
- Al-Sabagh, A.M.; Kandile, N.G.; Nasser, N.M.; Mishrif, M.R.; El- Tabey, A. Egypt. J. Petr. 2013, 22, 351–365. doi: 10.1016/j.ejpe.2013.10.004
- Badiea, A.M.; Mohana, K.N. Effect of Temperature and Fluid Velocity on Corrosion Mechanism of low Carbon Steel in Presence of 2-Hydrazino-4,7-Dimethylbenzothiazole in Industrial Water Medium. Corros. Sci. 2009, 51, 223–2241. doi: 10.1016/j.corsci.2009.06.011
- Zhang, Q.; Gao, Z.; Xu, F.; Zou, X. Adsorption and Corrosion Inhibitive Properties of Gemini Surfactants in the Series of Hexanediyl-1,6-bis-(Diethyl Alkyl Ammonium Bromide) on Aluminium in Hydrochloric Acid Solution. Colloids Surf. A Physicochem. Eng. Aspects 2011, 380, 191–200. doi: 10.1016/j.colsurfa.2011.02.035