ABSTRACT
This paper attempts to demonstrate empirically the possibility of using a hydrophobic extract of hop cone obtained under the conditions of supercritical carbon dioxide to produce all-purpose cleaner with antimicrobial activity and low irritant potential. A series of prototypes of products varied by the extract concentration was developed. The formulations were assessed for physicochemical properties: particle size, turbidity, and color. The results indicate that the hydrophobic extracts of hop cone can be a valuable component of chemical products intended for washing, having a broad spectrum of action and contributing to improvement of the product’s safety.
GRAPHICAL ABSTRACT
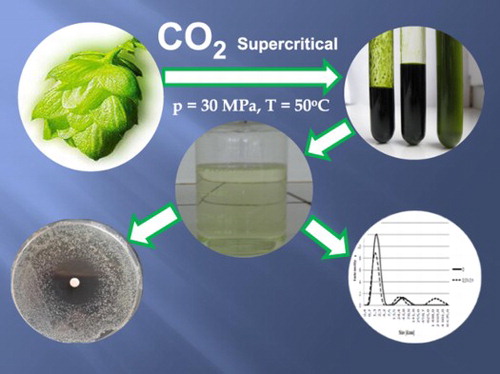
Introduction
All-purpose cleaners (acronym APC) are cleaning agents intended for general applications. They are typically used for cleaning slightly soiled areas including kitchen tops, cookers, painted, and ceramic surfaces, floors as well as plastic objects. APC should successfully handle a range of soils including organic residues (food remains, grease) and a broad spectrum of inorganic contaminants. Cleaning formulations are expected to remove all soiling enumerated above quickly, effectively, and safely. In addition to good detergent properties, however, they must also be safe for the surfaces on which they will be used. To prevent the scratching of objects or areas to be cleaned, APC do not contain abrasive agents ( Citation1–3).
APC should possess an array of qualities, the most important of which are good wetting, penetrating, and dispersing properties. A particularly significant aspect is the ability of cleaners to work effectively in cold water. Furthermore, they should have reduced foaming properties so that they can be easily removed from the surface being cleaned. All detergents which come into direct contact with the skin of the hands during use should be sufficiently mild, without a potential to produce skin irritation and cause excessively dry skin. Since APC may be used for cleaning surfaces and objects which get into contact with food, they must not contain potentially toxic substances, so that they are safe for the consumer even after inadequate removal from the surface cleaned ( Citation1–3).
In order to meet the requirements applicable to APC, care must be given to ensure their proper composition. A key role in the cleaner performance is attributed to surfactants which determine wetting, dispersing, and emulsifying effects. Commercially available cleaners are mainly formulated with anionic surface-active agents such as alkylbenzene sulfonates ( Citation4), sulfates of oxyethylenated fatty alcohols ( Citation5), or non-ionic surface-active agents, for example oxyethylenated fatty alcohols ( Citation6).
The detergent activity of APC can be enhanced by enriching their composition with amphiphilic organic solvents. The most commonly used substances include ethanol ( Citation7), isopropanol ( Citation8), ethylene glycol ( Citation9), propylene glycol ( Citation10), terpenes ( Citation11–13), etc. Amphiphilic organic solvents added to cleaners of this type significantly improve the dissolution of organic soils, increase the penetration of surface-active agents into soil particles, speed up the drying of cleaned surfaces, and enhance their gloss.
The formulations of APC also contain ingredients enhancing the cleaning performance – for example sequestrants which reduce water hardness, such as ethylenediaminetetraacetic acid disodium salt, sodium gluconate, sodium citrate, citric acid, calcium gluconate, gluconic acid, acetic acid, sodium phytate, calcium phytate ( Citation14–16). Other additions include substances intended to increase formulation appeal to consumers, for example hydrotropes which improve solution clarity – including salts of lower alkylaryl sulfonates, urea, ethanolamines ( Citation17) – but also colorants, fragrances, and preservatives.
As is known, substances with antimicrobial activity are commonly used in many fields, particularly in medical device technology, pharmacy, cosmetics technology, household chemistry, health care products, hygienic applications, etc. One of the most health problem is resistant microorganisms (bacteria, viruses, fungi, and protozoa) that mutate rapidly and easily, making their elimination difficult. The frequency of antimicrobial resistance in bacteria has increased in concert with increasing usage of antimicrobial compounds. From this reason, antimicrobial resistance in bacteria are an important threat to public health due to the slow development of new antimicrobial substances to replace those that become ineffective ( Citation18–20). Nowadays, phenols ( Citation21,Citation22), organic acids ( Citation23,Citation24) and, biguanides ( Citation25), surface-active agents ( Citation26,Citation27), aldehydes ( Citation28), halogens, chlorine compounds, heavy-metal derivatives ( Citation29), alcohols ( Citation30), terpenes ( Citation31,Citation32), quaternary ammonium compounds ( Citation33–36), esters and salts, aromatic diamidines, bromine, inorganic acid, quinoline and isoquinoline derivatives, peroxygens, anilides, derivatives of 1,3-dioxane, derivatives of imidazole, isothiazolone end derivatives of hexamine are used as antimicrobial substances in technology of above-mentioned products. The number of biocides in use is large and their activity varies. However, these substances can be dangerous to humans, animals, and the environment in a variety of ways due to their intrinsic ability to kill living organisms ( Citation37). From the standpoint of use safety, a potentially interesting alternative to the biocidal compounds listed above may be active substances of natural origin with proven antibacterial properties. Studies conducted to date have investigated the possible application of a number of compounds including eugenol ( Citation38,Citation39), thymol ( Citation40,Citation41), menthol and geraniol ( Citation42,Citation43), α-pinene and β-pinene ( Citation44,Citation45), linalool ( Citation46,Citation47), limonene and 1,8-cineole ( Citation48), carvacrol, α-tujone, lupulone, humulone, borneol, camphen, and others ( Citation49,Citation50).
In line with recent trends which place an emphasis on multifaceted activity (including antibacterial effects, natural aromas), a very interesting solution seems to be the application of natural extracts produced under supercritical carbon dioxide conditions in APCs. This type of extraction makes it possible to obtain materials at relatively low temperatures and eliminate the content of extraction solvents ( Citation51,Citation52). In addition, they are microbiologically pure and retain natural active ingredients. They are high-quality formulations which do not change their properties over a long storage period ( Citation53,Citation54). A promising approach to achieve APC with antibacterial action and natural aroma compounds could be using a hop cone extract obtained under supercritical conditions (Humulus lupulus CO2 extract). At present, hop cone extracts produced by this method are manufactured on an industrial scale (they are commonly used in the brewing industry) and have a very complex composition ( Citation55). These two qualities are an important argument for studying their suitability for use in household chemicals. Hop cone extract obtained under supercritical CO2 conditions mainly contains terpene derivatives including monoterpene hydrocarbons (7.4%), oxygenated monoterpenes (1.9%), sesquiterpene hydrocarbons (24.7%), oxygenated sesquiterpenes (9.5%), diterpene, triterpene, steroids (10.7%), and phloroglucinol derivatives (24.8%). The extract used in the studies contained: lupulone – 8,6%, α-humulene – 8,1%, (E)-β-farnesene – 7,5%, dehydrohumulinic acid – 7,5%, myrcene – 7,1%, 6α-acetoxy-4-propyl-3,4-seco-5α-androstene-3,17β-diol – 5,9%, trans-β-caryophyllene – 3,6%, dehydrocohumulinic acid – 3,0%, isopentyl butanoate – 2,4%, gymnomitrol – 2,3%, methyl caprylate – 2,1%, 17-hydroxy-5α,17α-pregnan-3-one – 2,1%, trans-isohumulone – 2%, garanyl isobuyrate – 1,8%, dehydroisohumulinic acid – 1,7%. ( Citation56).
The literature data show that antibacterial active ingredients found in hop cone extract include lupulones, humulones, isohumulone, humulinic acid, trans-β-caryophyllene, and β-pinene ( Citation56–64). The most important antifungal agents are terpenes, primarily α-humulene. The most potent antibacterial activity is associated with β-acids (lupulones) ( Citation65). Moreover, the presence of terpenes in the extract can be used for reinforcing the detergent activity of cleaning formulations. Other compounds used in similar applications involving terpenes include pine oil ( Citation66,Citation67), orange terpenes ( Citation68), D-limonene ( Citation69), and monoterpene mixtures ( Citation70,Citation71).
Moreover, hop cone extracts contain considerable quantities of natural pigments and fragrances which are beneficial alternatives to their synthetic equivalent. They have a long tradition of use in the perfume industry. They are usually added to formulations with a sharp oriental scent. The main aromatic substances present in hop cone extract are myrcene, α-humulene, (E)-β-farnesene, and trans-β-caryophyllene. The process of supercritical extraction of hop cones yields essential oils which constitute approx. 10% of the extract. One of the key ingredients of the oil is β-myrcene which gives it a sharp scent. Compounds responsible for a much more pleasant resin-like scent include β-caryophyllene and α-humulene ( Citation72).
An important aspect for ensuring safe cleaner use is the effect on reducing the skin irritation potential. Studies conducted to date have found that an addition of a hydrophobic extract may contribute to a decrease in the amount of free surfactant monomers getting into contact with the skin of the hands. In this way, it is possible to reduce the irritating effect on the skin ( Citation73,Citation74).
The study is an attempt to demonstrate via empirical means the possibility of using a hydrophobic hop cone extract obtained under supercritical carbon dioxide conditions in the production of APCs. To verify the hypotheses outlined above, a series of APC prototypes differing in the concentration of the hop cone extract was prepared. The prototypes thus obtained were subjected to a range of functional and physicochemical tests in order to determine the effect of the extract on formulation functionality and safety of use.
Materials and methods
Materials
The tests were performed with materials used in the household chemicals industry: Laureth-7 (trade name Rokanol L7; PCC ROKITA S.A., Brzeg Dolny, Poland), sodium laureth sulfate (trade name Texapon NSO; BASF, Ludwigshafen, Germany), Citric acid (Breentag), H. lupulus CO2 extract Hops – extract was produced from hops pellets (type 90, Marynka variety) by supercritical fluid extraction using carbon dioxide at pressures up to 30 MPa and at temperatures up to 50°C (New Chemical Syntheses Institute, Puławy, Poland), Methylchloroisothiasolinone and Methylisothiazolinone as preservatives (Euxyl K120; Schulke & Mayr, Fairfield, NJ, U.S.A.), distilled water.
Compositions and technology of preparing prototypical cleaners
Based on the professional literature ( Citation1–3,Citation36) and own experience ( Citation75,Citation76), prototypical APC formulations containing varying amounts of the hop cone extract were prepared. The compositions are listed in .
Table 1. Compositions of prototypical formulations.
The preparation process was as follows. The non-ionic surfactant Laureth-7 and hop cone extract were heated to 45°C and then mixed intensively (at approx. 300 rpm). In the next stage, an aqueous solution of sodium laureth sulfate was prepared. The two phases were then combined and mixed together for 30 min (at 200 rpm) until homogeneous consistency was obtained in the system. Next, sodium citrate was added, and the mixing procedure was repeated using a magnetic stirrer (200 rpm) for 5 min. The resulting formulations had the form of homogeneous liquids.
Methods
Formulation particle size
Measurements of mean particle size in the formulations, and an analysis of particle size distribution, were conducted using a Zetasizer Nano-ZS analyzer (Malvern Instruments, U.K.). The instrument relies on the technique of dynamic light scattering. The final result was the arithmetic mean of three independent measurements.
Turbidity
Turbidity was assessed by means of a EUTECH TN-100 turbidimeter. The final result was the arithmetic mean of five independent measurements.
Color
Parameters relating to the color of the formulations were determined using a Konica Minolta CR-400 colorimeter. The measurement was performed in the C.I.E. system based on determining three trichromatic components – L, a*, and b*. Every color determined in the C.I.E. space was defined by three components: L – indicates lightness (intensity of color brightness), a* – designates a value between red and green, b* – designates a value between yellow and blue.
The mean color parameters determined during measurements were used for calculating a change in total color difference (ΔE*) according to the following formula:where index x is the tested formulation, index b is the base formulation.
The results were processed using the following classification of absolute color differences (ΔE*):
0–1 – unidentifiable (deviation invisible);
1–2 – slight deviation identifiable by a person experienced in distinguishing nuances of color;
2–3.5 – moderate deviation identifiable even by an ordinary person;
3.5–5 – marked deviation;
Over 5 – large color deviation.
Antimicrobial activity
The antimicrobial activity of the tested formulations (performed on the formulations without synthetic preservatives) was determined against gram-positive bacterial strains (Staphylococcus aureus ATCC 25293). Microbial suspensions of 1.5×108 CFU/ml corresponding to 0.5 McFarland density obtained from an overnight culture of bacteria developed on solid media were used. Twenty five microliters of diluted inoculum was transferred onto Petri dishes containing Mueller-Hinton II agar. After 15 min of incubation, 6 mm paper discs filled with 15 μl of tested formulations were places onto each Petri discs in triplicate and incubated at 37°C for 16–18 h. The diameter of the inhibition zones was measured using a caliper.
The next stage involved determining the degree of antibacterial activity exhibited by formulations in contact with a bacterial test suspension of a S. aureus strain (ATCC 25923) using the method of dilution and neutralization laid down in the standard PN-EN 1440:2000. The tests were performed with formulations containing 0.5, 0.3, and 0.1% of hop cone extract in triplicate. To 8 mL of one of the test formulations was added 1 ml of distilled water and 1 ml of a bacterial test suspension containing 1.5×108 CFU/ml. The mixture was kept at the temperature of 20°C for 15 min. Next, a 1 ml portion of the test mixture was transferred to a test tube containing 8 ml 5% (v/v) of lecithin solution (neutralizer) and 1 ml of distilled water. After 5 min, a 1 ml portion of the neutralized mixture was transferred to Petri dishes onto which fluidized Trypticase Soy Agar (TSA) was added. After an incubation period of 16–18 h at the temperature of 37°C, the viable bacterial count (A) was determined with the formula:where c is the total of colonies in plates; n is the number of plates included in calculations; d is the dilution factor, 10−1; V is the volume of sample, 1 ml and the reduction of viable bacterial count (B) was calculated based on the formula:
where N is the number of CFU/ml in the bacterial test suspension prior to testing; Na is the number of CFU/ml in the bacterial test suspension after testing.
Irritant potential – zein value (ZV)
Irritant potential of the products was measured using the zein test. In the surfactant solution, zein protein is denatured and then is solubilized in the solution. This process simulates the behavior of surfactants in relation to the skin proteins. To 40 ml of all-purpose cleaners (APC) solution (10% wt.) was added 2 ± 0.05 g of zein from corn. The solutions with zein were shaken on a shaker with water bath (60 min at 35°C). The solutions were filtered on Whatman No. 1 filters and then centrifuged at 5000 rpm for 10 min. The nitrogen content in the solutions was determined by the Kjeldahl method. One milliliter of the filtrate was mineralized in a sulfuric acid (98%) containing copper sulfate pentahydrate and potassium sulfate. After mineralization, the solution was transferred (with 50 ml of Milli Q water) into the flask of the Wagner–Parnas apparatus. Twenty milliliters of sodium hydroxide (25 wt. %) was added. The released ammonia was distilled with steam, then the ammonia was bound by sulfuric acid (5 ml of 0.1 N H2SO4) in the receiver of the Wagner–Parnas apparatus. The unbound sulfuric acid was titrated with 0.1 N sodium hydroxide. Tashiro solution was used as an indicator. The zein number (ZN) was calculated from the equation:where V1 is the volume (cm3) of sodium hydroxide used for titration of the sample.
The final result was the arithmetic mean of three independent measurements.
Evaluation of foaming properties
The evaluation was performed in conformity with the Polish standard PN-EN 12728. To perform the assay, a 100 cm3 portion of 1% aqueous solution of the formulation was prepared in a glass graduated cylinder equipped with a perforated disc permanently attached to the end of the rod. Foam was created by beating with a frequency of 60 beats per 60 s. The volume of foam generated after 10 s, 1 min, and 10 min was then measured. The evaluated parameters included foaming ability (in cm3) expressed by the volume of foam present immediately after completing the measurement (10 s), and foam stability index (WTP) calculated from the equation:where V1 is the volume of foam measured after 1 min (cm3), V2 is the volume of foam measured after 10 min (cm3).
The final result was the arithmetic mean of five foam volume measurements performed at specified time intervals. The measurements were carried out at 20°C.
Error analysis
The points in the charts represent mean values from a series of three or five independent measurements. Error values are presented in the figures.
Results and discussion
The base formulation was a combination of two surfactant types: Laureth-7 and sodium laureth sulfate. Laureth-7 is classified as a non-ionic surfactant. An appropriate hydrophilic/hydrophobic ratio ensures that the compound has a high ability to reduce surface tension and improves surface wetting. The parameters are very important in the first stage of the washing process ( Citation65). The material used (Rokanol L7) has an anhydrous form, which makes it easier to introduce hydrophobic substances into the system. Sodium laureth sulfate is an anionic surfactant. It is characterized by good foaming and detergent properties. Sodium citrate is the sodium salt of hydroxy tricarboxylic acid. In APCs, sodium citrate is used as a sequestrant and as an ingredient improving the process of washing and cleaning.
The formulation was additionally enriched with hop cone extract obtained under supercritical CO2 conditions (H. lupulus CO2 extract) as an antibacterial compound.
Particle size
The particle size in the test formulations was determined ().
Table 2. Particle size in the test formulations.
Correlations determined for all test formulations revealed two characteristic peaks corresponding to the presence of particles in two size ranges: 1.8–2.3 and 15.4–32.3 nm. Particles of this type arise probably as a result of the aggregation of surfactants (Laureth-7 and sodium laureth sulfate) used for the preparation of the prototypical formulations. In formulations containing a hydrophobic hop extract, a third peak was noted, corresponding to particles with sizes in the range of 2330–3464 nm. The intensity of the peak grows along with increasing proportion of the extract (from 2% for the extract concentration of 0.01–10.3% for the concentration of 0.5%). The results show that the extract added to the formulation becomes dispersed, and the size of resulting particles is such that it allows the scattering of light, thus increasing the turbidity of the formulation.
Turbidity
The results of turbidity tests performed for the formulations are shown in .
The turbidity of the test formulations is in the range of 2.00–6.40 NTU. The value of the parameter for commercial products was within the range of 1.60–10.50 NTU. Based on the particle size determination (), it was found that an increase in the content of the extract led to a rise in the content of relatively large particles in the volume phase of the formulation. The tests showed that an elevated concentration of the extract in the formulation caused a slight increase in the parameter. For formulations containing 0.3 and 0.5% of hop cone extract, there was a rise in turbidity by respectively 1.06 and 1.36 NTU in relation to the formulation containing 0.1% of the extract. Nevertheless, it must be noted that the changes are practically invisible to the naked eye and do not have a major impact on the visual appearance of the formulation.
Color
The mean values of colorimetric parameters recorded for the test formulations are shown in .
Table 3. Mean values of colorimetric parameters for the test formulations.
Data yielded by the study demonstrate that an increase in the concentration of hop cone extract triggers a change in the color of the formulations manifesting as a decrease in the parameter describing lightness L*. For example, the difference for the formulation containing 0.5% of the extract and the base formulation is –6.55. At the same time, there is a slight increase in the proportion of the green color manifested as a decrease in the parameter a*, and a marked increase in the parameter b* defining the proportion of the yellow color. For the formulation containing 0.5% of the extract, for example, changes in the parameters a* and b* relative to the base formulation are –2.27 and + 22.14, respectively.
Changes in total color difference (ΔE*) as a function of hop cone extract concentration in the test formulations are shown in .
Figure 2. Changes in the total color difference (ΔE*) of the formulations as a function of hop cone extract concentration.
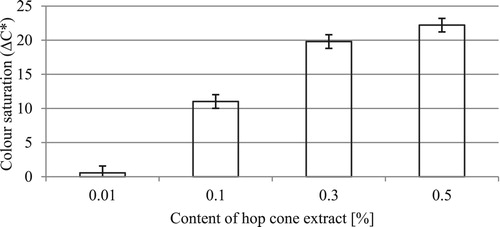
Even a small addition of hope cone extract (0.1%) triggers a noticeable change in color compared to the base formulation. The change, amounting to ΔE* = 11.02, is recognized by an ordinary person, considering that the threshold of detection by a person who is inexperienced in distinguishing colors is ΔE* = 2. An increase in extract concentration in the formulation leads to very significant color differences. For example, in 0.1 and 0.5% formulations the increase in the parameter tested is more than twofold.
The change in color stemming from the addition of hop extract to the formulations is probably attributable to the presence of chlorophyll – the basic green pigment present in plants. The pigments are derivatives of pyrrole which, based on the structure, can be separated into two main groups: chlorophyll a (dark green) and chlorophyll b (yellow-green). Depending on extraction conditions the ratio of the pigments may vary. The higher the temperature of extraction, the greater the amount of chlorophyll. Consequently, the resulting extract is darker in color. When the extraction temperature exceeds 70°C, the extract may become dark green and even brown in color. Due to the presence of hydrophobic groups, the compounds are practically insoluble in water ( Citation77,Citation78).
Evaluation of antimicrobial efficacy
The antimicrobial activity was evaluated on the formulations differing in the content of hop cone extract (). The tested formulations contained no synthetic preservatives. The tests also involved formulations containing hop cone extract at the concentrations of 0.001, 0.03, and 0.05%, in order to determine the antimicrobial effect of the extract also at lower concentrations. The antimicrobial effect was investigated on the basis of Gram-positive bacteria S. aureus, one of the most pathogenic species among streptococci, highly resistant to the conventional disinfectants and antiseptic agents. The results are shown in .
Figure 3. Antimicrobial activity of the test formulations against S. aureus ATCC 25923 (a); disc saturated with a formulation containing hop cone extract at the concentration of 0.5% (b).
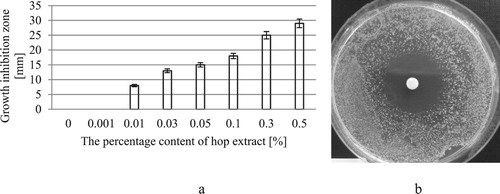
An antibacterial effect of the formulations against S. aureus was already observed at the hop cone extract concentration of 0.01%. The effect is visible as growth inhibition zones (8 mm for the formulation containing 0.01% of the extract) and increases with the increasing percentage content of the extract in the formulation (29 mm for the formulation containing 0.5% of the extract).
The evaluation of antimicrobial efficacy over time for the test formulations was performed according to the methodology set out in the standard PN-EN 1440:2000. The results of tests are listed in .
The results demonstrated that a 15-minute interaction of the test formulations with the test mixture of microorganisms reduces viable bacterial count by 5.0×104 CFU/ml for the formulation containing 0.5% of hop cone extract – up to 8.3×103 CFU/ml for the formulation containing 0.1% of the hop cone extract.
The antimicrobial activity of the extract against Gram-positive bacteria (particularly selected species of Micrococcus, Staphylococcus, Mycobacterium and Streptomycetes) stems from the presence of bitter α- and β-acids, i.e. humulones and lupolones ( Citation79–81). The probable mechanism of antimicrobial activity involves a change in the properties and permeability of microbial cell membranes following an interaction with the hydrophobic ingredients of the extract, resulting in a leakage of intracellular substances ( Citation82).
Irritant effect
The irritant effect of the formulations is shown in .
The test formulations based on a non-ionic surface-active agent are characterized by a very low irritant potential (in the range of 13.09–23.39 mg N/100 ml). The value of the parameter for commercial products was within the range of 31.54–133.35 mg N/100 ml. An addition of hydrophobic hop cone extract further lowers the value of the parameter. For the formulation containing 0.5% of the extract, there is a drop in the zein value by more than 10 mg N/100 ml in relation to the base formulation. Previous studies have shown that the irritant effect of cleaning formulations is chiefly attributable to monomers of surface-active agents which have an ability to penetrate into deeper skin layers ( Citation83,Citation84). The addition of hydrophobic extracts to a surfactant solution produces an increase in the size of emerging aggregates and makes them more stable (enlargement of distance between the hydrophilic parts of surfactant molecules decreases their mutual repulsive forces) ( Citation73,Citation74). An increased stability of resulting micelles may diminish the concentration of free monomers in the solution. Consequently, the intensity of impact of the surface-active agent is reduced, thus decreasing the irritant potential of the formulation ( Citation83–86). The results are consistent with the results reported by Wasilewski et al. ( Citation73,Citation74) for hand dishwashing liquids. They demonstrated that the presence of hydrophobic extract in the formulation led to the reduction of the irritant effect of the formulations. In the case of small concentrations of hydrophobic phase, intracellular solubilization occurs. Micelles transform into aggregates in the interior of which the hydrophobic substance is present. Surfactant monomers that are responsible for skin irritation are adsorbed on the surface of the aggregate. It is important to assume that the incorporation of the hydrophobic phase into the surfactant solution results in the formation of an “additional” phase boundary. It may have an effect on reducing free surfactant molecules in the system. In this way, the presence of a hydrophobic phase can lead to a decrease in irritant potential ( Citation74).
Foaming properties
The foaming properties determined for the test formulations are shown in .
Increasing concentrations of hop cone extract in the formulation were found to be correlated with significant differences in the volume of generated foam. The foaming ability determined in the study is within the range of 370–280 cm3. The value of the parameter for commercial products was within the range of 420–210 cm3. The test formulations were also analyzed to determine the values of foam stability index. It was observed that an increase in the proportion of hop cone extract in the formulation contributed to a decrease in the determined parameter in relation to the base system. For the base formulation, the index is 90%, and for the formulations containing 0.1% and 0.5% of hop cone extract it is 70 and 60%, respectively. The value of the parameter for commercial products was within the range of 90–40%. The foaming properties, however, are not a key parameter determining the quality of APCs. Formulations of this type are not, as a rule, washed off from cleaned surfaces with water, so large amounts of foam generated during the cleaning process and very long-lasting foam are, in fact, undesirable qualities (smudges, redeposition of soils).
Conclusions
The study yielded the following findings:
There is a possibility to produce stable and clear APCs containing a hydrophobic hop extract. The prototypical formulations obtained in the study had the required stability, visual appearance, and functionality.
Formulations containing hop extract at a level from 0.01% exhibit antimicrobial activity against S. aureus bacteria.
Formulations containing hop extract have a very low irritant potential.
An addition of hop cone extract has a favorable effect on the appearance of the formulation (by giving it a pleasant color) and its odor.
An addition of the extract slightly increases the turbidity of resulting formulations.
The extract may also be used as an efficient anti-foaming additive to APCs.
Acknowledgments
The work was carried out within the framework project No. POIR.01.01.02-00-0139/16 “Technology of implementation, combining probiotics with plant extracts obtained under supercritical CO2 conditions into the structure of the multifunctional ecological cleaning and washing product”, financed by POIR 2014-202.
Disclosure statement
No potential conflict of interest was reported by the authors.
Notes on contributors
Tomasz Wasilewski received his Ph.D. in Materials Science and Engineering from the Technical University of Warsaw, Poland (2004) and his DSc. in Commodity Science from the Cracow University of Economics, Poland (2014). Main research topics of Assoc. Prof. Tomasz Wasilewski are cosmetics and household products, their manufacturing, properties, quality assessment and physicochemistry of aqueous solutions of surfactants. Currently, he is the Head of Department of Chemistry and the Dean of the Faculty of Materials Science, Technology and Design at University of Technology and Humanities in Radom.
Dominik Czerwonka, M.Sc., Ph.D. student at University of Kazimierz Pulaski Technology and Humanities in Radom.
Urszula Piotrowska, M.Sc., Ph.D. student at Medical University of Warsaw.
Artur Seweryn received his Ph.D. from University of Kazimierz Pulaski Technology and Humanities in Radom.
Zofia Nizioł-Łukaszewska received her Ph.D. from University of Agriculture in Cracow, Poland in Faculty of Horticulture (2013). Currently works as a researcher in Department of Cosmetology at The University of Information Technology and Management in Rzeszow. Main research topics are antioxidant activity, plants physiology and application of plant ingredients in cosmetics production.
Marcin Sobczak received his Ph.D. in Chemical Sciences from the Warsaw University of Technology, Poland (2001) and his DSc in Pharmaceutical Sciences from the Medical University of Warsaw (2012).
ORCID
Artur Seweryn http://orcid.org/0000-0002-9916-769X
Additional information
Funding
References
- Wisniewski, K. In Liquid Detergent, 2nd ed.; Kuo-Yann, L., Ed.; CRC Press: New York, 2006; pp 555–621.
- Wisniewski, K. In Handbook of Detergents. Part E Applications; Zoller, U., Ed.; Taylor & Francis Group: Boca Raton, FL, 2009; pp 5–39.
- Scialla, S. In Handbook of Detergents. Part D – Formulations; Showell, M.S., Ed.; Taylor & Francis Group: Boca Raton, FL, 2009; pp 153–178.
- Andrade Da Luz, M.; Gallotti, M.; Nunes, G. Eur. Pat. Appl. EP 1162254 A1, 2001.
- Peters, D.S. Multi-purpose cleaning compositions and method. US Patent 7,592,303, September 22, 2009.
- Loth, M.; Lambremont, Y.; Blanvalet, C. Eur. Pat. Appl. EP 0347110 A1, 1989.
- Toussaint, C.; Andries N.; Mondin M. All-purpose cleaning compositions. US Patent 8,618,041, December 31, 2013.
- Cate, S.; Garabedian, A.; Cheng, L.; Deleeuw, D. Low residue cleaners for food contact surfaces. US Patent 0,293,202 A1, 2006.
- Requejo, L.P.; Keyes, G.B. Solvent, nonionic or anionic surfactant, builder system which includes polyacrylic acid or salt, fatty acid dimer alkali salt hydrotrope. US Patent 4,983,317, January 8, 1991.
- Rose, E.S.; Wile, R.G. All purpose cleaner and polish in abrasive applicator. US Patent 6,503,136, January 7, 2003.
- Frieser, E.P.; Jainschig, A. Liquid all-purpose cleaning preparations containing terpene and hydrogenated naphthalene as fat dissolving agent. US Patent 4,790,951, December 13, 1988.
- Williams, W.A. Terpene-based microemulsion cleaning composition. US Patent 5,213,624, May 25, 1993.
- Matta, G.B. D-Limonene based aqueous cleaning compositions. US Patent 4,511,488, 16 April 16, 1985.
- Leskowicz, J.J.; Horner, M.G. Rinseable hard surface cleaner comprising silicate and hydrophobic acrylic polymer. US Patent 5,770,548, July 23, 1998.
- Culshaw, S.; Coox, W. Eur. Pat. Appl. EP 0261874 A2, 1988.
- Colclough, V.L. Fast acting disinfectant and cleaner containing a polymeric biguanide. US Patent 6,303,557, October 16, 2001.
- Mondin, M.; Andries, N.; Massaux, J. Microemulsion all purpose liquid cleaning composition based on EO-PO nonionic surfactant. US Patent 5,854,193, February 20, 1998.
- Sobczak, M.; Dębek, C.; Olędzka, E.; Kozłowski, R. Molecules 2013, 18, 14122–14137. doi: 10.3390/molecules181114122
- Manaia, C.M.; Macedo, G.; Fatta-Kassinos, D.; Nunes, O.C. Appl. Microbiol. Biotechnol. 2016, 100, 1543–1557. doi: 10.1007/s00253-015-7202-0
- Cizmas, L.; Sharma, V.K.; Gray, C.M.; McDonald, T.J. Environ. Chem. Lett. 2015, 13, 381–394. doi: 10.1007/s10311-015-0524-4
- Pesaro, M.; Oertling, H. Eur. Pat. Appl. EP 2606725 A1, 2013.
- Das, J.R.; Rabone, K.L. Antimicrobial cleaning compositions containing aromatic alcohols or phenols. Int. Pat. Appl. WO 98/1524, 1998.
- Coruzzi, M.; Inamura, T.; Jamieson, A.S.; De Scheur Van, F.T.; Trombetta, I.; Vijayakrishnan, V. Antimicrobial cleaning compositions. Int. Pat. Appl. WO 2002050225, 2002.
- Cook, W.J.; Wisniewski, K.L.; Dixit, N.S.; Rao, N.S. Acidic disinfectant all-purpose liquid cleaning composition. US Patent 5,008,030, April 16, 1991.
- Godfroid, R.A.; Binski, C.J.; Morelli, J.P. Antimicrobial compositions for hard surfaces. US Patent 6,559,116, May 6, 2003.
- Both, S.; Muckenschnabel, Ch; Hazenkamp, M. Antimicrobial cleaning composition. Int. Pat. Appl. WO 2013045340, 2013.
- Scardera, M.; Grosser, F.R. Polyglycidol amine oxide surfactants having antimicrobial activity. US Patent 5,059,625, October 22, 1991.
- White, M.J.R.; Lis-Balchin, M.T.; Simpson, E.J.M.; Deans, S.D.; Hendrick, D.M.J.R. Eur. Pat. Appl. EP 1172118 B1, 2006.
- Purschwitz, J.; Hüffer, S.; Garcia, M.A.; Hazenkamp, M. Enhancing the antimicrobial activity of biocides with polymers. WO. Patent 124784 A1, 2013.
- Rees, W.M.; Hilgers, D.S. Biocidal cleaner composition containing acid-anionic surfactant-alcohol combinations and method of using the composition. US Patent 6,812,196, November 2, 2004.
- Lu, R.Z.; Kloeppel, A.A. Blooming type disinfecting cleaning compositions. US Patent 6,110,295, February 29, 2000.
- Barranx, A.; Barsaco, M.; Dufau, G.; Lauilhe, J.P. Eur. Pat. Appl. EP 0741519 B1, 2000.
- Elfersy, J.; Villahoz, M.D. Methods and compositions for biocidal treatments. US Patent 8,999,357, April 7, 2015.
- Fong, R.; Kong, S.; Peterson, D. Antimicrobial hard surface cleaner comprising an ethoxylated quaternary ammonium surfactant. US Patent 6,605,584, August 12, 2003.
- Polzin, T.E.; Werkowski, L.M. Anti-bacterial cleaning composition.US Patent 9,090,855, July 28, 2015.
- Graubart, B.T.; Streit, A.L.; Sachs, E.J.; Beronio, C.A. All purpose cleaning composition. US Patent 5,454,984, October 3, 1995.
- Pauwels, M.; Rogiers, V. Toxicol. Appl. Pharmacol. 2010, 243, 260–274. doi: 10.1016/j.taap.2009.12.007
- Devi, K.P.; Nisha, S.A.; Sakthivel, R.; Pandian, S.K. J. Ethnopharmacol. 2010, 130, 107–115. doi: 10.1016/j.jep.2010.04.025
- Bevilacqua, A.; Corbo, M.R.; Sinigaglia, M. J. Food Prot. 2010, 73, 888–894. doi: 10.4315/0362-028X-73.5.888
- Xu, J.; Zhou, F.; Ji, B.P.; Pei, R.S.; Xu, N. Lett. Appl. Microbiol. 2008, 47, 174–179. doi: 10.1111/j.1472-765X.2008.02407.x
- McGimpsey, J.A.; Douglas, M.H.; Van Klink, J.L.; Beauregard, D.A.; Perry, N.B. Flavour Fragr. J. 1994, 9, 347–352. doi: 10.1002/ffj.2730090613
- Gallucci, M.N.; Oliva, M.; Casero, C.; Dambolena, J.; Luna, A.; Zygadlo, J.; Demo, M. Flavour Fragr. J. 2009, 24, 348–354. doi: 10.1002/ffj.1948
- Pattnaikm, S.; Subramanyam, V.R.; Bapaji, M.; Kole, C.R. Microbios. 1997, 89, 39–46.
- Marino, M.; Bersani, C.; Comi, G. J. Food Prot. 1997, 62, 1017–1023. doi: 10.4315/0362-028X-62.9.1017
- Rivas da Silva, A.C.; Lopes, P.M.; Barros de Azevedo, M.M.; Costa, D.C.; Alviano, C.S.; Alviano, D.S. Molecules 2012, 17, 6305–6316. doi: 10.3390/molecules17066305
- Delaquis, P.J.; Stanich, K.; Girard, B.; Mazza, G. Int. J. Food Microbiol. 2002, 74, 101–109. doi: 10.1016/S0168-1605(01)00734-6
- Park, S.N.; Lim, Y.K.; Freire, M.O.; Cho, E.; Jin, D.; Kook, J.K. Anaerobe 2012, 18, 369–372. doi: 10.1016/j.anaerobe.2012.04.001
- van Vuuren, S.F.; Viljoen, A.M. Flavour Fragr. J. 2007, 22, 540–544. doi: 10.1002/ffj.1843
- Burt, S. Int. J. Food Microbiol. 2004, 94, 223–253. doi: 10.1016/j.ijfoodmicro.2004.03.022
- Tassou, C.C.; Chorianopoulos, N.G.; Skandamis, P.N.; Nychas, G.J.E. In Handbook of Herbs and Spices, Volume 2. Peter, K.V., Ed.; Woodhead Publishing Series in Food Science: Cambridge, 2012; pp 22–50.
- Lang, Q.; Wai, C.M. Talanta 2001, 53, 771–782. doi: 10.1016/S0039-9140(00)00557-9
- Reverchon, E. J. Supercrit. Fluids 1997, 10, 1–37.
- Sahena, F.; Zaidul, I.; Jinap, S. J. Food Eng. 2009, 95, 240–253. doi: 10.1016/j.jfoodeng.2009.06.026
- Kostrzewa, D.; Dobrzyńska-Inger, A.; Rój, E. Fluid Phase Equilib. 2013, 360, 445–450. doi: 10.1016/j.fluid.2013.10.001
- Rój, E.; Skowroński, B. Przem Chem. 2006, 85, 655–657.
- Rój, E.; Tadić, V.M.; Mišić, D.; Žižović, I.; Arsić, I.; Dobrzyńska-Inger, A.; Kostrzewa, D. Open Chem. 2015, 13, 1157–1171. doi: 10.1515/chem-2015-0131
- Langezaal, C.R.; Chandra, A.; Scheffer, J.J.C. Pharm. Weekbl. 1992, 14, 353–356. doi: 10.1007/BF01970171
- Pilna, J.; Vlkova, E.; Krofta, K.; Nesvadba, V.; Rada, V.; Kokoska, L. Fitoterapia 2015, 105, 260–268. doi: 10.1016/j.fitote.2015.07.016
- Teuber, M.; Schmareck, A.F. Arch. Mikrobiol. 1973, 94, 159–171. doi: 10.1007/BF00416690
- Zhao, F.; Watanabe, Y.; Nozawa, H.; Daikonnya, A.; Kondo, K.; Kitanaka, S. J. Nat. Prod. 2005, 68, 43–49. doi: 10.1021/np0499113
- Simpson, W.J.; Smith, A.R. J. Appl. Bacteriol. 1992, 72, 327–334. doi: 10.1111/j.1365-2672.1992.tb01843.x
- Van Cleemput, M.; Cattoor, K.; De Bosscher, K.; Haegeman, G.; De Keukeleire, D.; Heyerick, A. J. Nat. Prod. 2009, 72, 1220–1230. doi: 10.1021/np800740m
- Zeković, Z.; Pfaf-Šovljanski, I.; Grujić, O. J. Serb. Chem. Soc. 2007, 72 (1), 81–87. doi: 10.2298/JSC0701081Z
- Langezaal, C.R.; Chandra, A.; Scheffer, J.J.C. Planta Med. 1990, 56, 593. doi: 10.1055/s-2006-961203
- Niknejad, F.; Mohammadi, M.; Khomeiri, M.; Razavi, H.; Alami, M. Adv. Environ. Biol. 2015, 8, 395–401.
- Richter, A.F. Pine oil hard surface cleaning compositions. US Patent 5,591,708, January 7, 1997.
- Richter, A.F.; Taraschi, F.A. Reduced volatility, mixture of surfactants. US Patent 5,629,280, May 13, 1997.
- Taylor, G.E. Cleaning product. Int. Pat. Appl. WO 002698, 1987.
- Melikyan, A.V.; Stewart, P.H. Surfactants based aqueous compositions with D-limonene and hydrogen peroxide and methods using the same. US Patent 6,316,399, February 17, 2001.
- Brown, A.; Gorman, W.; Masters, R.A. Aqueous hard surface cleaners based on terpenes and fatty acid derivatives. US Patent 9,758,751, September 12, 2017.
- König, A. Liquid Detergent Compositions. Eur. Pat. Appl. EP 0080749 A1, 1983.
- Bernotiene, G.; Nivinskiene, O.; Butkiene, R.; Mockute, D. Chemija 2004, 15, 31–36.
- Wasilewski, T.; Seweryn, A.; Bujak, T. Green Chem. Lett. Rev. 2016, 9 (2), 114–121. doi: 10.1080/17518253.2016.1180432
- Wasilewski, T.; Seweryn, A.; Krajewski, M. J. Surf. Deterg. 2016, 19(6), 1315–1326. doi: 10.1007/s11743-016-1868-x
- Wasilewski, T.; Czerwonka, D.; Seweryn, A. Przem. Chem. 2016, 95 (4), 784–788.
- Wasilewski, T.; Czerwonka, D.; Piotrowska, U. Tenside Surfact. Det. 2016, 53 (4), 368–374. doi: 10.3139/113.110450
- Valle, J.M.; Rivera, O.; Teuber, O.; Palma, T. J. Sci. Food Agric. 2003, 13, 1349–1356. doi: 10.1002/jsfa.1547
- Brzozowski, R.; Kwiatkowski, J.; Jarosz, M.; Tęcza, W.; Goś, A. Method of obtaining hop extract. Patent description 329365, 1998.
- Teuber, M.; Schmalreck, A.F. Arch. Mikrobiol. 1973, 94, 159–171. doi: 10.1007/BF00416690
- Simpson, W.; Smith, A. J. Appl. Bacteriol. 1992, 72, 327–334. doi: 10.1111/j.1365-2672.1992.tb01843.x
- Ohsugi, M.; Basnet, P.; Kadota, S.; Ishii, E.; Tamura, T.; Okamura, Y.; Namba, T. J. Trad. Med. 1997, 14, 186–191.
- Zanoli, P.; Zavatti, M. J. Ethnopharmacol. 2008, 116, 383–396. doi: 10.1016/j.jep.2008.01.011
- Jackson, C.T.; Paye, M.; Maibach H. In Handbook of Cosmetic Science and Technology Fourth Edition: Barel, A.; Paye, M.; Maibach, H., Eds.; CRC Press; Taylor & Francis Group: Boca Raton, FL 2014; pp 353–365.
- Hall-Manning, T.J.; Holland, G.H.; Rennie, G.; Revell, P.; Hines, J.; Barratt, M.D.; Basketter, D.A. Food Chem. Toxicol. 1998, 36, 233–238. doi: 10.1016/S0278-6915(97)00144-0
- Seweryn, A.; Wasilewski, T.; Bujak, T. Ind. Eng. Chem. Res. 2016, 55, 1134–1141. doi: 10.1021/acs.iecr.5b04048
- Bujak, T.; Wasilewski, T.; Nizioł-Łukaszewska, Z. Colloids Surf. B Biointerfaces. 2015, 135, 497–503. doi: 10.1016/j.colsurfb.2015.07.051