ABSTRACT
Nanotechnology is a new star in the science horizon with many valuable applications and promises to offer. It includes the synthesis and utilization of nanostructure materials ranging from 1 to 100 nm. Mostly these materials are generally (or “could be”) produced via the laborious and hazard-prone physical and chemical methods but the green synthesis approaches easier, safe and scalable have been recently developed. Among other metal oxides nanoparticles, Titanium oxide (TiO2) nanoparticles have been mostly exploited for their photocatalytic, antimicrobial and antiparasitic applications. A diverse set of biological entities are used to reduce the precursor metal salt into respective nanoparticles. The secondary metabolites present in organisms such as plants or microbes are involved in the bio-reduction and capping processes. This article will provide an overview of the green synthesis of TiO2 NPs from different biological extracts such as plants, microbes and biological products as well as their potential applications.
GRAPHICAL ABSTRACT
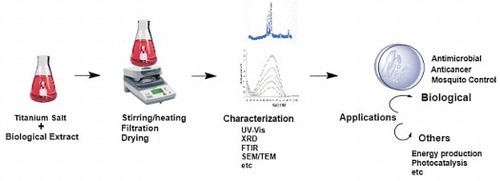
1. Introduction
Nanotechnology is an emerging field that has got phenomenal interest in the last few decades. The word “nano” means small, 1 billionth part of a meter Hussain et al. ( Citation1). The smaller size and unique surface chemistry of these nanostructures have been already exploited in medicine, nutrition, and energy Chandran et al. ( Citation2). Amongst other nanoparticles Titanium Oxide nanoparticles also exhibit unique surface chemistry and morphologies. It is used in synthesis of tints, textiles, papers, plastics, cosmetics, and foodstuffs Muhd Julkapli et al. ( Citation3). Colloidal TiO2 NPs are used in degradation of toxic chemicals in water Centi et al. ( Citation4), Pirkanniemi and Sillanpää ( Citation5). Mostly TiO2 NPs are produced via physicochemical methods like chemical vapor deposition, micro emulsion, chemical precipitation, hydrothermal crystallization, and sol–gel methods Muhd Julkapli and colleagues ( Citation3), Valencia et al. ( Citation6). All these methods require high temperature, pressure and toxic chemicals which limits their production and potential medical uses Chen et al. ( Citation7). Hence, an eco-friendly and cost-effective approach is needed to synthesize these nanosized materials on larger scale with lesser hazards Jayaseelan et al. ( Citation8). This could be possibly accomplished by using biological extracts as a reducing agent; the green synthesis approach. The same reducing agent can synthesize more than one type of metallic nanoparticle Edmundson et al. ( Citation9). Moreover, nanoparticles with better morphology and stability are also produced Suresh et al. ( Citation10), Bhainsa and D'souza ( Citation11), Song and Kim ( Citation12). Mostly water-soluble metabolites are involved in the reduction process. The bio-mediated TiO2 NPs have many applications such as diseases diagnostics, treatment, and manufacturing of surgical tools, tissue engineering, imaging, sensing, energy production and agriculture Jayaseelan and colleagues ( Citation8), Dobrucka ( Citation13), Órdenes-Aenishanslins et al. ( Citation14). This mini-review describes a brief overview of the research on bio-mediated TiO2 NPs from different plants, bacteria, fungi and other biological derivatives. Moreover, the potential applications of bio-mediated TiO2 NPs are also here emphasized.
2. Green synthesis of TiO2 nanoparticles from different sources
In attempt to develop a cost effective, eco-friendly and energy efficient approach, researchers have exploited the potential of biological resources for the synthesis of TiO2 NP Pantidos and Horsfall ( Citation15). The complete biosynthesis process as shown in , a simple precursor salt is mixed with biological extract; the metabolites present in the extract can then reduce and stabilize the bulk metal into elemental form following various mechanical steps. This biosynthetic approach offers many advantages and has emerged as a simple, safe and feasible substitute to chemical and physical methods Nadeem et al. ( Citation16), Asha et al. ( Citation17), Marimuthu et al. ( Citation18), Jalill et al. ( Citation19), Singh ( Citation20). Apart from these, biological approach can effectively catalyze the synthesis process at any scale and condition. Moreover, NPs with controlled size and shape can also be produced Bao et al. ( Citation21). Owing to these benefits, numerous researchers have intended to explore diverse species for their potential to synthesize TiO2 NPs.
2.1. Plants
Among the studied biological species, plants are considered as one of the most suitable candidates for the synthesis of NPs as they are cost effective, safe and easily available Mittal et al. ( Citation22). A diverse array of compounds in plants (phenolic acids, alkaloids, proteins and among them enzymes, as well as carbohydrates) regulate through reduction and stabilization processes the synthesis of NPs Dobrucka ( Citation13). Numerous plants species have been used for synthesis of various shapes of TiO2 NPs (). The reaction mixtures start vigorously when a precursor TiO2 salt is adulterated with plant extract, color change indicates the first sign of synthesis that can then be confirmed afterwards by spectroscopic techniques. Several color indicators have been reported ranging from light green to dark green in the formation of TiO2 NPs Dobrucka ( Citation13), Rajakumar et al. ( Citation23), Rajakumar et al. ( Citation24).
Table 1. TiO2 nanoparticles synthesized from different plants species.
Majority of green synthesis studies have been conducted on leaves extracts, as it is a rich source of metabolites. Spherical TiO2 NPs resulted when Annona squamosa L. was added to the aqueous solution of TiO2 salt at room temperature Roopan et al. ( Citation29). Similarly, leaf extract of Calotropis gigantea (L.) Dryand. was reported to reduce TiO2 to nanoparticles within 6 hours. This high bio-reduction potential was ascribed to the presence of primary amines containing in the extract. The bio-mediated TiO2 NPs showed good acaricidal activity against the larvae of Rhipicephalus microplus and Haemaphysalis bispinosa Marimuthu and colleagues ( Citation18). TiO2 NPs synthesized from aqueous leaf extract of medicinally important plant Catharanthus roseus (L.) G.Don has been reported. The aliphatic alcohols and amines present in the extract contributed to synthesis of TiO2 NPs. The particle size ranged between 25–110 nm with irregular morphologies Velayutham et al. ( Citation32). Bio-mediated TiO2 NPs have also been obtained from Morinda citrifolia L. extracts. The resultant NPs were confirmed by EDX, FTIR, SEM and XRD Sundrarajan et al. ( Citation41). The average size revealed via SEM was 15 nm, FTIR spectral data showed the presence of anthraquinones and various phenolic compounds which are supposed to be active reactants for the reduction of TiCl4 to TiO2 NPs. Moringa oleifera Lam. leaf extract was used to synthesize 100 nm nanoparticles with different structures and having good wound healing potential Sivaranjani and Philominathan ( Citation42). TiO2 NPs from Hibiscus rosa-sinensis L. leaf extract showed high antimicrobial activity against both gram-negative and positive strains of bacteria Kumar et al. ( Citation39). Nyctanthes leaves extracts derived TiO2 NPs had uniform spherical shape and ranged in size from about 100 to 510 nm. These NPs were found to have a significant pediculocidal, acaricidal, and larvicidal activity Sundrarajan and Gowri ( Citation43). Cicer arietinum L. beans extract TiO2 NPs (14 nm) have been synthesized, FTIR confirmed that the reduction is attributed to the presence of different phenolic compounds in the extract Kashale et al. ( Citation34). The variation in size and morphology observed among the reported studies is due to the influence of reaction temperature, time and source of plant Dobrucka ( Citation13), Roopan and colleagues ( Citation29). Thus, improvements can be established in these parameters as these aspects influence the synthesis mechanism. Furthermore, a wide propitious plant extracts are left to be explored for the synthesis of TiO2 NPs.
2.2. Microorganism-based synthesized NPs
Many microbial species have been exploited to synthesize metallic NPs Nadeem and colleagues ( Citation16). TiO2 NPs with various shapes and sizes have been reported in recent years (). Bacterial extracts in this regard have also been utilized for the green synthesis of TiO2 NPs. Similarly, like their plant counterparts, bacterial metabolites also play a crucial role in the bio-reduction and stabilization of TiO2 NPs. Órdenes-Aenishanslins and colleagues ( Citation14) reported synthesis of TiO2 NPs by using Bacillus mycoides, spherical NPs (40–60 nm) were observed and confirmed by UV, TEM, FTIR and DLS. TiO2 NPs (28–54 nm) synthesized using Aeromonas hydrophila extract showed effective inhibitory activity against Staphylococcus aureus (33 mm inhibition zone) and S. pyogenes (31 mm inhibition zone) Jayaseelan and colleagues ( Citation8). Jha et al. ( Citation49) observed the biosynthesis of TiO2 NPs by the bacterium Lactobacillus and proposed that these NPs were synthesized through the combined action of oxidoreductases and glucose at mild pH. However, owing to their potential pathogenicity and laborious process bacterial synthesis have fever chances to be commercialized.
Table 2. TiO2 nanoparticles synthesized from different microbial species.
The use of fungi in synthesis of metallic NPs has got substantial attention as they offer certain benefits over bacterial synthesis Pantidos and Horsfall ( Citation15). Ease of scaling up, easier extraction, large surface area and economic viability are significant advantages to consider Mukherjee et al. ( Citation61). Fungus mediated, TiO2 NPs with various shapes and sizes have been reported in recent years as well (). Fungus have the intrinsic ability to reduce bulk salt to elemental or ionic state either via enzymes or metabolites (). Extract of Aspergillus flavus was reported to have capability of reducing TiO2 ions to TiO2 NPs. The NPs showed good antibacterial activity against E.coli Rajakumar et al. ( Citation57). Saccharomyces cerevisa extract has also been used to synthesize TiO2 NPs (12.6 nm), FTIR results confirmed that the reduction was attributed to the quinines and membranes reductases present in cells Jha and colleagues ( Citation49). The surface chemistry and pH of culture media also play an important role in synthesis of TiO2 NPs. Like bacteria, fungi mediated TiO2 NPs also have safety drawbacks. However, the application of non-pathogenic strains will wipe out the risk and could be used for commercial purposes.
2.3. Biological derivatives
Various biological derivatives as a green source for nanoparticle synthesis have also been utilized apart from micro and macro organisms Balasooriya et al. ( Citation62). However, only a few researchers have particularly explored the use of biological derivatives for biomimetic synthesis of TiO2 nanoparticles. For instance, Farag et al. ( Citation63) utilized cellulose in order to synthesize TiO2 nanoparticle having average size of 5–10 nm. The peptide R5, biological derivative of Cylindrothica fusirormis have also been exploited for the synthesis of TiO2 nanoparticles Sewell and Wright ( Citation64). Titanium nano wires synthesized using alpha synuclein, a protein have been reported by Padalkar et al. ( Citation65). Bacterial flagella were used by Li et al 2012as template for production of titanium nanotubes. The lignocellulose waste material derived from rice straw has also been utilized for the synthesis of TiO2 nanoparticles Ramimoghadam et al. ( Citation66). Chen et al. ( Citation67) reported biomimetic synthesis to produce TiO2 nanoparticles using different enzymes. Rutile TiO2 nanoparticles were obtained when using glucose oxidase enzyme, catalase resulted in formation of anatase TiO2 nanoparticles while lysozyme resulted in formation of monoclinic anatase TiO2 nanoparticles Chen et al. ( Citation68). Some other notable biological derivatives used for biomimetic synthesis of TiO2 nanoparticles are given in . In compassion with microbial-derived NPs, these derivatives based NPs are safe, cost efficient and scalable as well. Such NPs could be scaled for commercial applications including food and pharmaceuticals. Nevertheless, more studies should be carried out to address their safety/toxicity.
Table 3. TiO2 nanoparticles synthesized from biological derivatives.
3. Applications of green TiO2 NPs
Green mediated NPs have many applications in various fields of physical sciences, medicine and enginneering technology Subhapriya and Gomathipriya ( Citation48). Though, biogenic TiO2 NPs are studied for a lesser number of practical applications. However, results proclaim that these green synthesized NPs exhibit huge potential as compared to chemical and physical mode of synthesis. The most important application which is widely exploited is their incredible photocatalytic activity to clean contaminated water and eliminate environmental pollutants Sankar and colleagues ( Citation31), Pelaez et al. ( Citation78). It also exhibits a vast application in field of electronics, energy production, making of batteries and sensors Órdenes-Aenishanslins and colleagues ( Citation14), Kashale and colleagues ( Citation34). The biomedical potential of green mediated TiO2 NPs have also been exploited, the main stream application includes photodynamic cancer treatment, antileshmanial agent and antimicrobial therapies Rajakumar and colleagues ( Citation57), Sahu and colleagues ( Citation37), Zahir and colleagues ( Citation38). The latter sections of the present review highlights, the most exploited biomedical applications with mechanistic approaches.
3.1. Antimicrobial potential
In literature, different metallic NPs have been used against various strains of bacteria Nadeem and colleagues ( Citation16). Similarly, TiO2 NPs also exhibit eco-friendly biocidal properties, which are attributed to their strong oxidizing potential. These NPs have been used against a wide range of infectious microbes including various bacterial strains, endospores, fungi, algae, protozoa, viruses, microbial toxins and prions Visai et al. ( Citation79). TiO2 NPs trigger the onset of reactive oxygen species (ROS) when confronted with microbial cells Jayaseelan and colleagues ( Citation8). These ROS kill microbes by disrupting cell wall’s integrity mainly by phospholipids oxidation, which results in reduced adhesion and distorted ionic balance. Inside the cytosol, it inhibits the respiratory cytosolic enzymes and modifying macromolecules structures, producing substantial effects on cellular integrity and gene expression. Furthermore, it also decreases the phosphate uptake and cellular communication across the cell Jayaseelan and colleagues ( Citation8), Kubacka et al. ( Citation80). A possible mechanism of action is described in .
Both green synthesized and chemically derived TiO2 NPs kill microbes in same fashion but the biologically derived NPs show better antibacterial activity. Their excellent antimicrobial potential is attributed to the capping agents provided by the plant extracts Kumar and colleagues ( Citation39). Morphology of NPs, membrane biochemistry and type of bacteria significantly affect antibacterial activity. Green synthesized TiO2 NPs can efficiently inhibit both gram-positive and gram-negative bacteria, but due to comparative structural complexity of gram-negative bacterial cell wall, it is more reactive against gram-positive bacteria Marimuthu and colleagues ( Citation18). Some other studies of bio-mediated TiO2 NPs tested against various stains of pathogens are presented in . The antimicrobial activity of bio-mediated TiO2 NPs can be enhanced if irradiated with UV and fluorescent light Jayaseelan and colleagues ( Citation8), Roopan and colleagues ( Citation29). TiO2 NPs Nano composites also exhibit enhanced antileshmanial activity, when green synthesized TiO2 NPs were applied to Leishmanial cultures; lower cell viability, stunted growth and DNA fragmentation was observed Kubacka and colleagues ( Citation80). When compared to standard antibiotic disk TiO2 NPs showed better antimicrobial activity Santhoshkumar and colleagues ( Citation46). Thus with such improved antimicrobial activity it considerably diminishes the occurrences for the development of antibiotic confrontation of pathogenic stains.
Table 4. TiO2 nanoparticles synthesized from different microbial species.
3.2. Anti-parasitic activities
Metallic nanoparticles have been effectively used against many species of parasitic larva and adult’s insects Benelli et al. ( Citation81). Green synthesized TiO2 NPs have also been found to be effective larvicidal agents against various species of parasitic insects as shown in . The subcellular events leading to death include decrease in the biochemical parameters involved in growth and development Durairaj and colleagues ( Citation55) (). To date less has been revealed regarding the antiparasitic potential of TiO2. However, it shows huge potential in making anti-insect lotions and ointments and many others features, which will be unveiled as the technology emerges.
Table 5. List of larva species tested against TiO2 NPs.
3.3. Photocatalytic activity
Industrial and domestic effluents contain many hazardous pollutants such as toxic dyes and nitroarene compounds. Their low solubility and high stability makes them highly persistent and resulting in many threats to aquatic life Valentín et al. ( Citation82). Recently, the distinctive structures and high catalytic potential of metallic NPs have been exploited. Their large surface area makes them excellent heterogeneous catalysts Rodrigues et al. ( Citation83). Moreover, these nanostructure catalysts can be easily recovered and recycled reaction mixture. But the agglomeration and toxicity of these metallic nanoparticles remain a concern Hotze et al. ( Citation84), Park et al. ( Citation85). TiO2 NPs have been used mostly in catalyzing various reactions, due to their benign properties, low toxicity, high stability, excellent photocatalytic potential and optical properties Shah et al. ( Citation86). The photocatalytic activity of green mediated TiO2 NPs have been reported by various authors for reduction of various dyes and compounds Valencia and colleagues ( Citation6), Bao and colleagues ( Citation21), Sankar and colleagues ( Citation31), Muniandy and colleagues ( Citation71), KyuáKim and HyeokáPark ( Citation75), Pelaez and colleagues ( Citation78). shows a typical pathway of electron flow after the possible photo excitation. The photo excited electron initiates a cascade of electron transfer chain which results in degradation of toxic compounds aided by oxidizing potential of phytochemicals of the extracts Ganesan and colleagues ( Citation26), Muniandy and colleagues ( Citation71), Pelaez and colleagues ( Citation78). When compared with chemical synthesized TiO2, the green mediated NPs showed excellent photocatalytic potential. The reducing ability is dependent on plant species, type of dye and temperature Zahir and colleagues ( Citation38). For instance when doped with other NPs and composites it significantly improve the catalytic potential. Salomatina et al. ( Citation87). TiO2 NPs exhibit an incredible photocatalytic activity as revealed by these results, but still the reducing phenomenon is not elucidated completely which must be assessed.
Conclusion
Nanostructures materials have triggered a considerable attention in every field of science. Though metallic nanoparticles are produced by physio chemical process, but their toxicity, cost and laborious synthesis have led scientists to devolop new approaches for designing nanostructures. The solution of this dilemma resulted in an easy, safe and scalable approach known as the green synthesis method. In this method a precursor metallic salts is reduced by the metabolites of the organism. Moreover, NPs with high yield and better morphologies are also obtained. In this review article, we have discussed the synthesis of titanium oxide nanoparticles from different biological sources (plants, microbes and related bio-products). Furthermore the applications of green mediated titanium oxide NPs have also been described. Though, Titanium NPs have been reported by many authors till now but the synthesis steps have to be better characterized and need to be elaborated further by the identification of the responsible compounds in the extracts, the optimization of different factors such as pH, temperature or the amount of precursor salt, and extract should be studied for optimal yield and stable NPs production at feasible commercial scale.
Disclosure statement
No potential conflict of interest was reported by the authors.
Notes on contributors
Muhammad Nadeem is an MPhil graduate scholar from Quaid I Azam University Islamabad, Pakistan. His research interests includes nanoparticle synthesis and their potential applications and toxicity.
Duangjai Tungmunnithum is a research scientist with expertise in biochemistry, medicinal chemistry and phytochemistry.
Christophe Hano is a senior research scientist. His research area includes medicinal plants biotechnology, plants physiology and nanoparticles synthesis.
Bilal Haider Abbasi is a senior scientist. His area of research includes bioprocess technology, medicinal plants biotechnology and green synthesis of nanoparticles.
Syed Salman Hashmi is an MPhil research Scholar at Quaid I Azam University Islamabad Pakistan. His current research is focused on the green synthesis of nanoparticles and its biological applications.
Waqar Ahmad is an MPhil research Scholar at Quaid i Azam University Islamabad Pakistan. His current research is focused on the green synthesis of nanoparticles and interactions with plants.
Adnan Zahir is an MPhil research Scholar at Quaid i Azam University Islamabad Pakistan. Presently he is working on biosynthesis of metallic nanoparticles and plant tissue culture.
ORCID
Muhammad Nadeem http://orcid.org/0000-0002-0373-5366
Christophe Hano http://orcid.org/0000-0001-9938-0151
Bilal Haider Abbasi http://orcid.org/0000-0002-6529-2134
Notes
Abbreviations: EDAX: energy dispersive X-ray; FESEM: field emission scanning electron microscope; FTIR: Fourier-transform infrared spectroscopy; SAED: selected area (electron) diffraction; SEM: Scanning electron microscopy; TEM: transmission electron microscopy; TGA: thermo gravimetric analysis; UV: Ultraviolet; XRD: X-ray powder diffraction
References
- Hussain, I.; Singh, N.; Singh, A.; Singh, H.; Singh, S. Green Synthesis of Nanoparticles and its Potential Application. Biotechnol. Lett. 2016, 38 (4), 545–560. doi: 10.1007/s10529-015-2026-7
- Chandran, S.P.; Chaudhary, M.; Pasricha, R.; Ahmad, A.; Sastry, M. Synthesis of Gold Nanotriangles and Silver Nanoparticles Using Aloevera Plant Extract. Biotechnol. Prog. 2006, 22 (2), 577–583. doi: 10.1021/bp0501423
- Muhd Julkapli, N.; Bagheri, S.; Bee Abd Hamid, S. Recent Advances in Heterogeneous Photocatalytic Decolorization of Synthetic Dyes. Sci. World J. 2014, 2014, 1–25. doi:10.1155/2014/692307.
- Centi, G.; Ciambelli, P.; Perathoner, S.; Russo, P. Environmental Catalysis: Trends and Outlook. Catal. Today 2002, 75 (1), 3–15. doi: 10.1016/S0920-5861(02)00037-8
- Pirkanniemi, K.; Sillanpää, M. Heterogeneous Water Phase Catalysis as an Environmental Application: A Review. Chemosphere 2002, 48 (10), 1047–1060. doi: 10.1016/S0045-6535(02)00168-6
- Valencia, S.; Vargas, X.; Rios, L.; Restrepo, G.; Marín, J.M. Sol–gel and Low-temperature Solvothermal Synthesis of Photoactive Nano-titanium Dioxide. J. Photochem. Photobiol., A 2013, 251, 175–181. doi: 10.1016/j.jphotochem.2012.10.025
- Chen, Y.-F.; Tsai, H.-Y.; Wu, T.-S. Anti-inflammatory and Analgesic Activities from Roots of Angelica Pubescens. Planta Med. 1995, 61 (1), 2–8. doi: 10.1055/s-2006-957987
- Jayaseelan, C.; Rahuman, A.A.; Roopan, S.M.; Kirthi, A.V.; Venkatesan, J.; Kim, S.-K.; Iyappan, M.; Siva, C. Biological Approach to Synthesize TiO2 Nanoparticles Using Aeromonas Hydrophila and Its Antibacterial Activity. Spectrochim. Acta, Part A 2013, 107, 82–89. doi: 10.1016/j.saa.2012.12.083
- Edmundson, M.C.; Capeness, M.; Horsfall, L. Exploring the Potential of Metallic Nanoparticles Within Synthetic Biology. New Biotechnology 2014, 31 (6), 572–578. doi: 10.1016/j.nbt.2014.03.004
- Suresh, K.; Prabagaran, S.; Sengupta, S.; Shivaji, S. Bacillus Indicus sp. nov., an Arsenic-resistant Bacterium Isolated from an Aquifer in West Bengal, India. Int. J. Syst. Evol. Microbiol. 2004, 54 (4), 1369–1375. doi: 10.1099/ijs.0.03047-0
- Bhainsa, K.C.; D'souza, S. Extracellular Biosynthesis of Silver Nanoparticles Using the Fungus Aspergillus Fumigatus. Colloids Surf. B 2006, 47 (2), 160–164. doi: 10.1016/j.colsurfb.2005.11.026
- Song, J.Y.; Kim, B.S. Rapid Biological Synthesis of Silver Nanoparticles Using Plant Leaf Extracts. Bioproc. Biosyst. Eng. 2009, 32 (1), 79. doi: 10.1007/s00449-008-0224-6
- Dobrucka, R. Synthesis of Titanium Dioxide Nanoparticles Using Echinacea Purpurea Herba (Spring 2017). Iran. J. Pharm. Res. 2017, 16, 756–762.
- Órdenes-Aenishanslins, N.A.; Saona, L.A.; Durán-Toro, V.M.; Monrás, J.P.; Bravo, D.M.; Pérez-Donoso, J.M. Use of Titanium Dioxide Nanoparticles Biosynthesized by Bacillus Mycoides in Quantum dot Sensitized Solar Cells. Microb. Cell Fact. 2014, 13 (1), 90.
- Pantidos, N.; Horsfall, L.E. Biological Synthesis of Metallic Nanoparticles by Bacteria, Fungi and Plants. J. Nanomed. Nanotechnol. 2014, 5 (5), 1. doi: 10.4172/2157-7439.1000233
- Nadeem, M.; Abbasi, B.H.; Younas, M.; Ahmad, W.; Khan, T. A Review of the Green Syntheses and Anti-microbial Applications of Gold Nanoparticles. Green Chem. Lett. Rev. 2017, 10 (4), 216–227. doi: 10.1080/17518253.2017.1349192
- Asha, A.; Sivaranjani, T.; Thirunavukkarasu, P.; Asha, S. Green Synthesis of Silver Nanoparticle from Different Plants – A Review. Int. J. Pure App. Biosci. 2016, 4 (2), 118–124. doi: 10.18782/2320-7051.2221
- Marimuthu, S.; Rahuman, A.A.; Jayaseelan, C.; Kirthi, A.V.; Santhoshkumar, T.; Velayutham, K.; Bagavan, A.; Kamaraj, C.; Elango, G.; Iyappan, M. Acaricidal Activity of Synthesized Titanium Dioxide Nanoparticles Using Calotropis Gigantea Against Rhipicephalus microplus and Haemaphysalis bispinosa. Asian Pacific J. Trop. Med. 2013, 6 (9), 682–688. doi: 10.1016/S1995-7645(13)60118-2
- Jalill, R.D.A.; Nuaman, R.S.; Abd, A.N. Biological Synthesis of Titanium Dioxide Nanoparticles by Curcuma Longa Plant Extract and Study its Biological Properties. World Sci. News 2016, 49 (2), 204–222.
- Singh, P. Biosynthesis of Titanium Dioxide Nanoparticles and Their Antibacterial Property. World Acad. Sci. Eng. Technol. Int. J. Chem. Mol. Nucl. Mater. Metall. Eng. 2016, 10 (2), 275–278.
- Bao, S.-J.; Lei, C.; Xu, M.-W.; Cai, C.-J.; Jia, D.-Z. Environment-friendly Biomimetic Synthesis of TiO2 Nanomaterials for Photocatalytic Application. Nanotechnology 2012, 23 (20), 205601. doi: 10.1088/0957-4484/23/20/205601
- Mittal, A.K.; Bhaumik, J.; Kumar, S.; Banerjee, U.C. Biosynthesis of Silver Nanoparticles: Elucidation of Prospective Mechanism and Therapeutic Potential. J. Colloid Interface Sci. 2014, 415, 39–47. doi: 10.1016/j.jcis.2013.10.018
- Rajakumar, G.; Rahuman, A.A.; Priyamvada, B.; Khanna, V.G.; Kumar, D.K.; Sujin, P. Eclipta Prostrata Leaf Aqueous Extract Mediated Synthesis of Titanium Dioxide Nanoparticles. Mater. Lett. 2012, 68, 115–117. doi: 10.1016/j.matlet.2011.10.038
- Rajakumar, G.; Rahuman, A.A.; Jayaseelan, C.; Santhoshkumar, T.; Marimuthu, S.; Kamaraj, C.; Bagavan, A.; Zahir, A.A.; Kirthi, A.V.; Elango, G. Solanum Trilobatum Extract-mediated Synthesis of Titanium Dioxide Nanoparticles to Control Pediculus Humanus Capitis, Hyalomma Anatolicum Anatolicum and Anopheles Subpictus. Parasitol. Res. 2014, 113 (2), 469–479. doi: 10.1007/s00436-013-3676-9
- Madadi, Z.; Lotfabad, T.B. Aqueous Extract of Acanthophyllum Laxiusculum Roots as a Renewable Resource for Green Synthesis of Nano-sized Titanium Dioxide Using Sol-Gel Method. Adv. Ceram. Prog. 2016, 2 (1), 26.
- Ganesan, S.; Babu, I.G.; Mahendran, D.; Arulselvi, P.I.; Elangovan, N.; Geetha, N.; Venkatachalam, P. Green Engineering of Titanium Dioxide Nanoparticles Using Ageratina Altissima (L.) King & HE Robines. Medicinal Plant Aqueous Leaf Extracts for Enhanced Photocatalytic Activity. Ann. Phytomedicine – Int. J. 2016, 5, 69–75. doi: 10.21276/ap.2016.5.2.8
- Rao, K.G.; Ashok, C.; Rao, K.V.; Chakra, C.S.; Tambur, P. Green Synthesis of TiO2 Nanoparticles Using Aloe Vera Extract. Int. J. Adv. Res. Phys. Sci. 2015, 2 (1A), 28–34.
- Khadar, A.; Behara, D.K.; Kumar, M.K. Synthesis and Characterization of Controlled Size TiO2 Nanoparticles Via Green Route Using Aloe Vera Extract. Int. J. Sci. Res. 2016, 5 (11), 1913–1916.
- Roopan, S.M.; Bharathi, A.; Prabhakarn, A.; Rahuman, A.A.; Velayutham, K.; Rajakumar, G.; Padmaja, R.; Lekshmi, M.; Madhumitha, G. Efficient Phyto-Synthesis and Structural Characterization of Rutile TiO2 Nanoparticles Using Annona Squamosa Peel Extract. Spectrochim. Acta, Part A 2012, 98, 86–90. doi: 10.1016/j.saa.2012.08.055
- Saravanan, P.; Ganapathy, M.; Charles, A.; Tamilselvan, S.; Jeyasekaran, R.; Vimalan, M. Electrical Properties of Green Synthesized TiO2 Nanoparticles. 2016.
- Sankar, R.; Rizwana, K.; Shivashangari, K.S.; Ravikumar, V. Ultra-rapid Photocatalytic Activity of Azadirachtaindica Engineered Colloidal Titanium Dioxide Nanoparticles. Appl. Nanosci. 2015, 5 (6), 731–736. doi: 10.1007/s13204-014-0369-3
- Velayutham, K.; Rahuman, A.A.; Rajakumar, G.; Santhoshkumar, T.; Marimuthu, S.; Jayaseelan, C.; Bagavan, A.; Kirthi, A.V.; Kamaraj, C.; Zahir, A.A. Evaluation of Catharanthus Roseus Leaf Extract-mediated Biosynthesis of Titanium Dioxide Nanoparticles Against Hippobosca Maculata and Bovicola Ovis. Parasitol. Res. 2012, 111 (6), 2329–2337. doi: 10.1007/s00436-011-2676-x
- Valli, G.; Geetha, S. A Green Method for the Synthesis of Titanium Dioxide Nanoparticles Using Cassia Auriculata Leaves Extract. J. Biomed. Pharm. Sci 2015, 2, 490–497.
- Kashale, A.A.; Gattu, K.P.; Ghule, K.; Ingole, V.H.; Dhanayat, S.; Sharma, R.; Chang, J.-Y.; Ghule, A.V. Biomediated Green Synthesis of TiO2 Nanoparticles for Lithium ion Battery Application. Composites Part B Eng. 2016, 99, 297–304. doi: 10.1016/j.compositesb.2016.06.015
- Naik, G.K.; Mishra, P.M.; Parida, K. Green Synthesis of Au/TiO2 for Effective dye Degradation in Aqueous System. Chem. Eng. J. 2013, 229, 492–497. doi: 10.1016/j.cej.2013.06.053
- Rao, K.G.; Ashok, C.; Rao, K.V.; Chakra, C.S.; Rajendar, V. Synthesis of TiO2 Nanoparticles from Orange Fruit Waste. Synthesis 2015, 2 (1), 1.
- Sahu, N.; Soni, D.; Chandrashekhar, B.; Sarangi, B.K.; Satpute, D.; Pandey, R.A. Synthesis and Characterization of Silver Nanoparticles Using Cynodon Dactylon Leaves and Assessment of Their Antibacterial Activity. Bioproc. Biosyst. Eng. 2013, 36 (7), 999–1004. doi: 10.1007/s00449-012-0841-y
- Zahir, A.A.; Chauhan, I.S.; Bagavan, A.; Kamaraj, C.; Elango, G.; Shankar, J.; Arjaria, N.; Roopan, S.M.; Rahuman, A.A.; Singh, N. Green Synthesis of Silver and Titanium Dioxide Nanoparticles Using Euphorbia Prostrata Extract Shows Shift From Apoptosis to G0/G1 Arrest Followed by Necrotic Cell Death in Leishmania Donovani. Antimicrobial Agents Chemother. 2015, 59 (8), 4782–4799. doi: 10.1128/AAC.00098-15
- Kumar, P.S.M.; Francis, A.P.; Devasena, T. Biosynthesized and Chemically Synthesized Titania Nanoparticles: Comparative Analysis of Antibacterial Activity. J. Environ. Nanotechnol 2014, 3 (3), 73–81. doi: 10.13074/jent.2014.09.143098
- Hudlikar, M.; Joglekar, S.; Dhaygude, M.; Kodam, K. Green Synthesis of TiO 2 Nanoparticles by Using Aqueous Extract of Jatropha Curcas L. Latex. Mater. Lett. 2012, 75, 196–199. doi: 10.1016/j.matlet.2012.02.018
- Sundrarajan, M.; Bama, K.; Bhavani, M.; Jegatheeswaran, S.; Ambika, S.; Sangili, A.; Nithya, P.; Sumathi, R. Obtaining Titanium Dioxide Nanoparticles with Spherical Shape and Antimicrobial Properties Using M. Citrifolia Leaves Extract by Hydrothermal Method. J. Photochem. Photobiol., B 2017, 171, 117–124. doi: 10.1016/j.jphotobiol.2017.05.003
- Sivaranjani, V.; Philominathan, P. Synthesize of Titanium Dioxide Nanoparticles Using Moringa Oleifera Leaves and Evaluation of Wound Healing Activity. Wound Med. 2016, 12, 1–5. doi: 10.1016/j.wndm.2015.11.002
- Sundrarajan, M.; Gowri, S. Green Synthesis of Titanium Dioxide Nanoparticles by Nyctanthes Arbor-Tristis Leaves Extract. Chalcogenide Lett. 2011, 8 (8), 447–451.
- Jayasinghe, C.; Gotoh, N.; Aoki, T.; Wada, S. Phenolics Composition and Antioxidant Activity of Sweet Basil (Ocimum Basilicum L). J. Agric. Food Chem. 2003, 51 (15), 4442–4449. doi: 10.1021/jf034269o
- Hunagund, S.M.; Desai, V.R.; Kadadevarmath, J.S.; Barretto, D.A.; Vootla, S.; Sidarai, A.H. Biogenic and Chemogenic Synthesis of TiO 2 NPs via Hydrothermal Route and Their Antibacterial Activities. RSC Adv. 2016, 6 (99), 97438–97444. doi: 10.1039/C6RA22163G
- Santhoshkumar, T.; Rahuman, A.A.; Jayaseelan, C.; Rajakumar, G.; Marimuthu, S.; Kirthi, A.V.; Velayutham, K.; Thomas, J.; Venkatesan, J.; Kim, S.-K. Green Synthesis of Titanium Dioxide Nanoparticles Using Psidium Guajava Extract and Its Antibacterial and Antioxidant Properties. Asian Pacific Journal of Tropical Medicine 2014, 7 (12), 968–976. doi: 10.1016/S1995-7645(14)60171-1
- Chatterjee, A.; Nishanthini, D.; Sandhiya, N.; Abraham, J. , Biosynthesis of Titanium Dioxide Nanoparticles Using Vigna Radiata. Asian J Pharm Clin Res. 2016, 9 (4), 1–4.
- Subhapriya, S.; Gomathipriya, P. Green Synthesis of Titanium Dioxide (TiO2) Nanoparticles by Trigonella Foenum-Graecum Extract and its Antimicrobial Properties. Microbial Pathogenesis 2018, 116, 215–220. doi: 10.1016/j.micpath.2018.01.027
- Jha, A.K.; Prasad, K.; Kulkarni, A. Synthesis of TiO2 Nanoparticles Using Microorganisms. Colloids Surf., B 2009, 71 (2), 226–229. doi: 10.1016/j.colsurfb.2009.02.007
- Khan, R.; Fulekar, M. Biosynthesis of Titanium Dioxide Nanoparticles Using Bacillus Amyloliquefaciens Culture and Enhancement of its Photocatalytic Activity for the Degradation of a Sulfonated Textile dye Reactive Red 31. J Colloid Interface Sci. 2016, 475, 184–191. doi: 10.1016/j.jcis.2016.05.001
- Kirthi, A.V.; Rahuman, A.A.; Rajakumar, G.; Marimuthu, S.; Santhoshkumar, T.; Jayaseelan, C.; Elango, G.; Zahir, A.A.; Kamaraj, C.; Bagavan, A. Biosynthesis of Titanium Dioxide Nanoparticles Using Bacterium Bacillus Subtilis. Mater. Lett. 2011, 65 (17), 2745–2747. doi: 10.1016/j.matlet.2011.05.077
- Dhandapani, P.; Maruthamuthu, S.; Rajagopal, G. Bio-mediated Synthesis of TiO2 Nanoparticles and its Photocatalytic Effect on Aquatic Biofilm. J. Photochem. Photobiol., B 2012, 110, 43–49. doi: 10.1016/j.jphotobiol.2012.03.003
- Prasad, K.; Jha, A.K.; Kulkarni, A. Lactobacillus Assisted Synthesis of Titanium Nanoparticles. Nanoscale Res. Lett. 2007, 2 (5), 248. doi: 10.1007/s11671-007-9060-x
- Malarkodi, C.; Chitra, K.; Rajeshkumar, S.; Gnanajobitha, G.; Paulkumar, K.; Vanaja, M.; Annadurai, G. Novel Eco-friendly Synthesis of Titanium Oxide Nanoparticles by Using Planomicrobium sp. and Its Antimicrobial Evaluation. Der Pharmacia Sinica 2013, 4 (3), 59–66.
- Durairaj, B.; Xavier, T.; Muthu, S. Research Article Fungal Generated Titanium Dioxide Nanoparticles: A Potent Mosquito (Aedes aegypti) Larvicidal Agent.
- Babitha, S.; Korrapati, P.S. Biosynthesis of Titanium Dioxide Nanoparticles Using a Probiotic from Coal Fly Ash Effluent. Mater. Res. Bull. 2013, 48 (11), 4738–4742. doi: 10.1016/j.materresbull.2013.08.016
- Rajakumar, G.; Rahuman, A.A.; Roopan, S.M.; Khanna, V.G.; Elango, G.; Kamaraj, C.; Zahir, A.A.; Velayutham, K. Fungus-mediated Biosynthesis and Characterization of TiO2 Nanoparticles and Their Activity Against Pathogenic Bacteria. Spectrochim. Acta, Part A 2012, 91, 23–29. doi: 10.1016/j.saa.2012.01.011
- Bansal, V.; Rautaray, D.; Bharde, A.; Ahire, K.; Sanyal, A.; Ahmad, A.; Sastry, M. Fungus-mediated Biosynthesis of Silica and Titania Particles. J. Mater. Chem. 2005, 15 (26), 2583–2589. doi: 10.1039/b503008k
- Bansal, V.; Poddar, P.; Ahmad, A.; Sastry, M. Room-temperature Biosynthesis of Ferroelectric Barium Titanate Nanoparticles. J. Am. Chem. Soc. 2006, 128 (36), 11958–11963. doi: 10.1021/ja063011m
- Raliya, R.; Tarafdar, J. Biosynthesis and Characterization of Zinc, Magnesium and Titanium Nanoparticles: an eco-Friendly Approach. Int. Nano Lett. 2014, 4 (1), 93. doi: 10.1007/s40089-014-0093-8
- Mukherjee, P.; Ahmad, A.; Mandal, D.; Senapati, S.; Sainkar, S.R.; Khan, M.I.; Parishcha, R.; Ajaykumar, P.; Alam, M.; Kumar, R. Fungus-mediated Synthesis of Silver Nanoparticles and Their Immobilization in the Mycelial Matrix: A Novel Biological Approach to Nanoparticle Synthesis. Nano Lett. 2001, 1 (10), 515–519. doi: 10.1021/nl0155274
- Balasooriya, E.R.; Jayasinghe, C.D.; Jayawardena, U.A.; Ruwanthika, R.W.D.; Mendis de Silva, R.; Udagama, P.V. Honey Mediated Green Synthesis of Nanoparticles: New Era of Safe Nanotechnology. J. Nanomater. 2017, 2017. doi:10.1155/2017/5919836.
- Farag, A.A.; El-shafei, A.; Ibrahim, H.M.; Asker, M.S. Influence of Bacterial Cellulose for Synthesis and Application of Titanium Dioxide Nanoparticles Compared with Sol-Gel Method. Int. J. Life Sci. Res. 2016, 4 (1), 69–75.
- Sewell, S.L.; Wright, D.W. Biomimetic Synthesis of Titanium Dioxide Utilizing the R5 Peptide Derived From Cylindrotheca F Usiformis. Chem. Mater. 2006, 18 (13), 3108–3113. doi: 10.1021/cm060342p
- Padalkar, S.; Schroeder, K.; Won, Y.; Jang, H.; Stanciu, L. Biotemplated Silica and Titania Nanowires: Synthesis, Characterization and Potential Applications. J. Nanosci. Nanotechnol. 2012, 12 (1), 227–235. doi: 10.1166/jnn.2012.5128
- Ramimoghadam, D.; Bagheri, S.; Abd Hamid, S.B. Biotemplated Synthesis of Anatase Titanium Dioxide Nanoparticles via Lignocellulosic Waste Material. BioMed. Res. Int. 2014, 2014. doi:10.1155/2014/205636.
- Chen, G.; Li, M.; Li, F.; Sun, S.; Xia, D. Protein-mediated Synthesis of Nanostructured Titania with Different Polymorphs at Room Temperature. Adv. Mater. 2010, 22 (11), 1258–1262. doi: 10.1002/adma.200902901
- Chen, J.; Saggar, J.K.; Corey, P.; Thompson, L.U. Flaxseed and Pure Secoisolariciresinol Diglucoside, but not Flaxseed Hull, Reduce Human Breast Tumor Growth (MCF-7) in Athymic Mice. J. Nutrition 2009, 139 (11), 2061–2066. doi: 10.3945/jn.109.112508
- Yang, D.; Qi, L.; Ma, J. Eggshell Membrane Templating of Hierarchically Ordered Macroporous Networks Composed of TiO2 Tubes. Adv. Mater. 2002, 14 (21), 1543–1546. doi: 10.1002/1521-4095(20021104)14:21<1543::AID-ADMA1543>3.0.CO;2-B
- Bagheri, S.; Chekin, F.; Hamid, S.B.A. Cobalt Doped Titanium Dioxide Nanoparticles: Synthesis, Characterization and Electrocatalytic Study. J. Chin. Chem. Soc. 2014, 61 (6), 702–706. doi: 10.1002/jccs.201300486
- Muniandy, S.S.; Kaus, N.H.M.; Jiang, Z.-T.; Altarawneh, M.; Lee, H.L. Green Synthesis of Mesoporous Anatase TiO2 Nanoparticles and Their Photocatalytic Activities. RSC Adv. 2017, 7 (76), 48083–48094. doi: 10.1039/C7RA08187A
- Shi, J.; Yang, D.; Jiang, Z.; Jiang, Y.; Liang, Y.; Zhu, Y.; Wang, X.; Wang, H. Simultaneous Size Control and Surface Functionalization of Titania Nanoparticles Through Bioadhesion-assisted Bio-inspired Mineralization. J. Nanopart. Res. 2012, 14 (9), 1120. doi: 10.1007/s11051-012-1120-1
- Cole, K.E.; Ortiz, A.N.; Schoonen, M.A.; Valentine, A.M. Peptide-and Long-chain Polyamine-Induced Synthesis of Micro-and Nanostructured Titanium Phosphate and Protein Encapsulation. Chem. Mater. 2006, 18 (19), 4592–4599. doi: 10.1021/cm060807b
- Yan, Y.; Hao, B.; Wang, X.; Chen, G. Bio-inspired Synthesis of Titania with Polyamine Induced Morphology and Phase Transformation at Room-temperature: Insight into the Role of the Protonated Amino Group. Dalton Trans. 2013, 42 (34), 12179–12184. doi: 10.1039/c3dt50359c
- KyuáKim, J.; HyeokáPark, J. Lysozyme-mediated Biomineralization of Titanium–Tungsten Oxide Hybrid Nanoparticles with High Photocatalytic Activity. Chem. Commun. 2014, 50 (82), 12392–12395. doi: 10.1039/C4CC04820B
- Zhang, G.; Zhang, T.; Li, B.; Zhang, X.; Chen, X. Biomimetic Synthesis of Interlaced Mesh Structures TiO2 Nanofibers with Enhanced Photocatalytic Activity. J. Alloys Compd. 2016, 668, 113–120. doi: 10.1016/j.jallcom.2016.01.197
- Jiang, Y.; Yang, D.; Zhang, L.; Sun, Q.; Sun, X.; Li, J.; Jiang, Z. Preparation of Protamine–Titania Microcapsules Through Synergy Between Layer-by-layer Assembly and Biomimetic Mineralization. Adv. Funct. Mater. 2009, 19 (1), 150–156. doi: 10.1002/adfm.200800974
- Pelaez, M.; Nolan, N.T.; Pillai, S.C.; Seery, M.K.; Falaras, P.; Kontos, A.G.; Dunlop, P.S.; Hamilton, J.W.; Byrne, J.A.; O'shea, K. A Review on the Visible Light Active Titanium Dioxide Photocatalysts for Environmental Applications. Appl. Catal., B 2012, 125, 331–349. doi: 10.1016/j.apcatb.2012.05.036
- Visai, L.; De Nardo, L.; Punta, C.; Melone, L.; Cigada, A.; Imbriani, M.; Arciola, C.R. Titanium Oxide Antibacterial Surfaces in Biomedical Devices. Int. J. Artif. Organs 2011, 34 (9), 929–946. doi: 10.5301/ijao.5000050
- Kubacka, A.; Diez, M.S.; Rojo, D.; Bargiela, R.; Ciordia, S.; Zapico, I.; Albar, J.P.; Barbas, C.; Dos Santos, V.A.M.; Fernández-García, M. Understanding the Antimicrobial Mechanism of TiO2-based Nanocomposite Films in a Pathogenic Bacterium. Sci. Rep. 2014, 4, 4134. doi: 10.1038/srep04134
- Benelli, G.; Caselli, A.; Canale, A. Nanoparticles for Mosquito Control: Challenges and Constraints. J. King Saud Univ. Sci. 2016, 29, 424–435. doi: 10.1016/j.jksus.2016.08.006
- Valentín, L.; Nousiainen, A.; Mikkonen, A. Introduction to Organic Contaminants in Soil: Concepts and Risks. In Emerging Organic Contaminants in Sludges, Springer: Berlin, Heidelberg, 2013; pp 1–29.
- Rodrigues, T.S.; Fajardo, H.V.; Dias, A.; Mezalira, D.Z.; Probst, L.F.; Stumpf, H.O.; Barros, W.P.; de Souza, G.P. Synthesis, Characterization and Catalytic Potential of MgNiO2 Nanoparticles Obtained From a Novel [MgNi (Opba)]n· 9nH2O Chain. Ceram. Int. 2016, 42 (12), 13635–13641. doi: 10.1016/j.ceramint.2016.05.158
- Hotze, E.M.; Phenrat, T.; Lowry, G.V. Nanoparticle Aggregation: Challenges to Understanding Transport and Reactivity in the Environment. J. Environ. Qual. 2010, 39 (6), 1909–1924. doi: 10.2134/jeq2009.0462
- Park, H.-O.; Yu, M.; Kang, S.K.; Yang, S.I.; Kim, Y.-J. Comparison of Cellular Effects of Titanium Dioxide Nanoparticles with Different Photocatalytic Potential in Human Keratinocyte, HaCaT Cells. Mole. Cell. Toxicol. 2011, 7 (1), 67–75. doi: 10.1007/s13273-011-0010-4
- Shah, S.N.A.; Shah, Z.; Hussain, M.; Khan, M. Hazardous Effects of Titanium Dioxide Nanoparticles in Ecosystem. Bioinorg. Chem. Appl. 2017, 2017, 1. doi:10.1155/2017/4101735.
- Salomatina, E.; Loginova, A.; Ignatov, S.; Knyazev, A.; Spirina, I.; Smirnova, L. Structure and Catalytic Activity of Poly (Titanium Oxide) Doped by Gold Nanoparticles in Organic Polymeric Matrix. J. Inorg. Organomet. Polym. Mater. 2016, 26 (6), 1280–1291. doi: 10.1007/s10904-016-0409-4