ABSTRACT
In this paper, we have reported the green synthesis of silver nanoparticles (AgNPs) using silver nitrate and Ficus carica (fig) stem extract at room temperature. We have also explored the effect of volume of extract (1–3 ml) on the formation of AgNPs using various analytical techniques such as Fourier-transform infrared spectroscopy (FTIR), ultraviolet–visible spectroscopy (UV–vis), scanning electron microscopy (SEM), and energy-dispersive X-ray analyzer (EDX). The formations of AgNPs were monitored by ultraviolet–visible spectrophotometer as well as from color change through the naked eye. The absorption peaks appear between 442 and 455 nm, confirming to surface plasmon resonance (SPR) of AgNPs. It is clear from the FTIR results that the biologically active compounds present in the extract act as capping and reducing agents for AgNPs creation. Electron microscopy results show that by increasing the concentration of extract, the morphology and size of AgNPs changed due to the presence of more phenolic group. The green synthesized AgNPs have been sequentially employed for the selective and sensitive detection of toxic metal ion (Hg2+) in aqueous medium and antimicrobial activity against all tested microbes including five gram negative and two gram positive. Furthermore, the brown color of green synthesized AgNPs turned into colorless with Hg2+ ion by naked eye response and the limit of detection was 1.06 µM.
Figure (A) represents the UV–visible spectra of AgNPs with different concentrations of Hg2+ (0.1–10 µM) in aqueous solution and inset shows the variation of the absorbance of AgNPs solution as a function of Hg2+ ion concentration.
Figure (B) represents the images of color variations of AgNPs with different concentrations of Hg2+ (10−1–10−7 M) in aqueous solution.
GRAPHICAL ABSTRACT
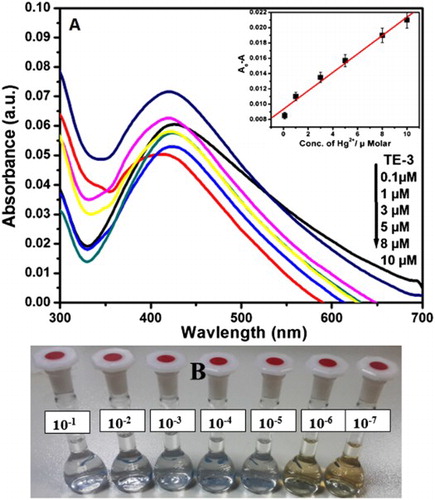
1. Introduction
It is well known that the noble metal nanoparticles in the range of 1–100 nm have received much more attraction in the area of modern nanotechnologies among the researchers due to their unique optical, electronic, biocompatibility, and catalytic properties ( Citation1–4). The biosynthesized silver nanoparticles (AgNPs) are the most preferred due to their cost-effectiveness, eco-friendliness, and ease of synthesis and have various most effective applications such as in catalysis, antibacteria, nonlinear optics, as optical receptor, bio-labeling, as coating for solar energy absorption, for electrical batteries, therapeutic, anti-HIV activity ( Citation5, Citation6). Generally, various approaches, such as sequential injection ( Citation7), reduction ( Citation8), irradiation-assisted chemical reaction ( Citation9), biosynthesis ( Citation10), and physical approaches ( Citation11, Citation12), have been reported for the synthesis of different shapes and sizes of AgNPs. The synthesis of AgNPs by chemical reduction method is the most common approach. But these methods used toxic and hazardous chemicals which pollute the environment. Earlier studies have reported that the green synthetic methods have fascinated more attention of researchers because they are cost-effective, eco-friendly, more stable, and simple. Various natural resources, such as leaf extract, seed extract, bacteria, enzymes, ( Citation13–16) have already been used to synthesize and stabilize AgNPs. However, most of them require long time and give poor Surface Plasmon Resonance (SPR) band. Fig plant (Ficus carica) is a naturally occurring plant and a native tree of Arab and African countries. It is easily available, cost effective, nontoxic, and eco-friendly. The fig fruit, white milk, and extract are used in traditional medicines for the treatment of cardiovascular disorders, as anti-inflammatory and antispasmodic remedy and for gastrointestinal diseases ( Citation17, Citation18). Only few reports are available on the synthesis of AgNPs using fig extract, but no report is yet available by using fig stem extract ( Citation19–21). Ulug et al. reported the synthesis of AgNPs by fig leaf extract and studied the role of light irradiation. The AgNPs are very popular as sensors due to their highly sensitive colorimetric properties.
To the best of our knowledge, there has been no new report on the synthesis of AgNPs using aqueous fig stem extract. In continuation of our previous work, the intention of the current study is to perform green synthesis and characterization of AgNPs using the fig stem extract, which acts as a reducing and capping agent. Herein, we report the prepared AgNPs established as optical sensor for naked eye sensing of toxic metal (Hg2+) in aqueous medium and solid state. Moreover, antimicrobial activity against all tested microbes, including gram negative: Klebsiella oxytoca, Klebsiella pneumonia, Shigella flexneri, Proteus mirabilis, and Pseudomonas aeruginosa, as well as gram positive: Staphylococcus aureus and Candida albicans and the effect of amount of extract have also been addressed. The result showed the absorption intensity of prepared silver nanoparticle decreased in the presence of Hg2+ ion and the limit of detection was 1.06 µM. The paper strip has been used as the solid substrate for colorimetric sensing of different metal ions and the results revealed that AgNPs are extremely selective to mercury ion over other metal ions. Based on the present report, the prepared AgNPs revealed the multifunctional properties (such as chemosensor and antibacterial).
2. Materials and methods
2.1. Materials
All the reagents and solvents were used as received without further purification. Silver nitrate (AgNO3) (BDH reagent, ≥99.0%) was purchased from Sigma–Aldrich Co. Double distilled water was used in all experiments. The microbial strains were obtained from the Microbiology Lab., Faculty of Medicine, King Khalid University, Saudi Arabia.
2.2. Extraction and synthesis of AgNPs
Fig stems were procured from Abha near King Khalid University and washed several times with double distilled water to eliminate dust particles and kept at dark place for drying. 5.0 g of sieved dry fig (F. carica) stems is admixed with 250 ml distilled water and left it for 24 h at room temperature. Prior to an experiment, stem extract was centrifuged at 35000 rpm for 5 min to isolate solid particles from it. The aqueous extract was stored and used for the synthesis of AgNPs. Three samples of AgNPs (TE-1, TE-2, and TE-3) were prepared by adding 10 ml silver nitrate (0.01 molar) solutions dropwise into 1, 2, 3 ml of fig stem extract at 25oC. After 30 minutes, the color of mixture changes (from colorless) to light yellow, but it finally changes to dark brown with the increase in the time, which is the clear sign for the formation of AgNPs ( inset shows the images of TE-3 and extract). The prepared AgNP solution was stored at room temperature for further study.
2.3. Colorimetric sensing
For colorimetric sensing of various metal ions, the metal salt solutions of 10−3 M were added into the dilute solution of green synthesized AgNPs (final volume 10 ml) which demonstrated selective decolorization through naked eye only for Hg2+ion and recorded the spectra (c). In addition, for paper-based colorimetric sensing of metal ions, the prepared AgNPs (TE-3) were first added on the Whatman filter paper by using Eppendorf on the selected test zone and dried. In the next step, the prepared solution of different metal ions (10−3 M) was applied using capillary tube on the test zone area (b). For mercury sensing, we prepared Hg2+ ion solution with different concentrations (10−1–10−7 M) and added to the solution containing 0.1 ml AgNPs (TE-3) in a 10 ml volumetric flask. The spectral measurements were recorded by using UV–visible spectrophotometer after the addition of mercury solution.
2.4. Biological studies
By using well diffusion methods, the antimicrobial activity of AgNPs stem extracts of F. carica was examined in triplicate sets against seven of human pathogenic microbes including five Gram-negative bacterial strains (K. oxytoca, K. pneumonia, S. flexneri, P. mirabilis, and P. aeruginosa) and one Gram-positive bacterial strain S. aureus and a pathogenic fungus C. albicans ( Citation22, Citation23). Each microbial strain was first subcultured in nutrient broth then equally distributed over solidified nutrient agar medium (109 spores/ml). In each agar plate, a well of 6 mm was made by using a sterilized cork borer. 100 µl from the prepared extract was poured in each well then plates were incubated for 48 h at 31°C. Cefotoxin 30 µg was used as a positive control and dimethyl sulfoxide (DMSO) as a negative control. The obtained inhibition zones for three replicates were measured.
2.5. Characterizations
The absorption spectra were recorded on UV–visible spectrophotometer (PG instrument) and the progress of reaction was observed by its color change. The surface morphologies and elements present in AgNPs were studied using scanning electron microscopy (SEM) and EDX (SEM; JEOL JSM-6360LA). Fourier transform infrared spectroscopy (FTIR) was used to determine the functional group and bioreductant. It was carried out in the range of 4000–500 cm−1 at room temperature by using JASCO 460 plus.
3. Results and discussion
3.1. UV–visible analysis
It is well established that the change in color from light yellow to dark brown during reaction between AgNO3 solution and plant extract is the clear sign for the formation of AgNPs ( Citation19, Citation24, Citation25). The same thing occurs with our experiment after the addition of different volumes of fig stem extract (1, 2, and 3 ml) to 10 ml AgNO3 solution and we noted a visible color change from colorless to dark brown ( inset). The intensity of color increased by increasing the volume of extract and reaction time, which is due to the SPR and reduction of Ag+ to Ag0 by the presence of bioreductant in the extract ( Citation26).
represents the UV–visible spectra of pure extract and synthesized AgNPs using different volumes of extract. From the figure, it can be observed that fig stem extract showed absorption band at 312.4 nm. The pure extract did not exhibit any absorption peak between 400 and 500 nm which is a clear indication that there are no nanoparticles. But for others SPR band appeared around 442–455 nm, which suggested the formation of spherical AgNPs. When the volume of extract increased from 1 to 3 ml, the intensity of SPR band increased and band slightly blue-shifted, which are the characteristics of the increase in the number of AgNPs and decrease in the particle size ( Citation27, Citation28). In addition, we can also see from figure that 1 ml of extract is not sufficient to increase the number of AgNPs formed due to less number of bio-reducing agents in the extract. Therefore, we particularly utilized AgNPs which are prepared by applying 3.0 ml of extract for further studies.
3.2. Morphological study
The morphology and size of green synthesized AgNPs were determined using SEM. represents the SEM images of green synthesized AgNPs with different volume of fig stem extract and 10 ml AgNO3 solution. It is clear from the SEM images that the volume of extract had no notable effect on the morphology. The morphology of green synthesized AgNPs was mostly spherical, caped and highly agglomerated except TE-3, which is slightly cubically spherical shape with 72 nm average particle size. Decreasing the size of AgNPs by increasing the volume of extract could be due to the presence of more polyphenol in the extract. It is reported that the presence of more bio-reducing agent leads to the formation of smaller particle sizes ( Citation29–31). The presence of silver in the synthesized AgNPs was confirmed by EDX results (). The peaks detected around 2.6, 3, and 3.20 keV can be consigned to the binding energy of Ag TE-1, Ag TE-2, and Ag TE-3, respectively ( Citation13). This observation indicates that AgNPs are capped by bioextract.
3.3. FTIR analysis
We used FTIR to determine the possible functional group present in the fig stem extract which are responsible for the reduction and stabilization of AgNPs. displays the FTIR spectra of pure fig stem extract and synthesized AgNPs. The pure extract showed characteristic absorption bands at 3400 (hydroxyl stretching band of alcohol and phenol), 1593 (hydroxyl bending of water), 1136 (C‒O stretching), 1040 and 853 (C‒O stretching vibration in carboxylic), 1357 (ascribed to C‒O‒H bending vibration of phenolic group) ( Citation32–34). The results revealed that carboxylic and hydroxyl groups might be responsible for the reduction of Ag+ to Ag (O). FTIR spectra of fig extract and AgNPs exhibit almost the same peaks which suggest that their capping molecules are the same. Decrease in intensity and shift in frequencies of synthesized AgNPs indicates the interaction of O–H on the surface of AgNPs. Therefore, the FTIR results revealed that the biomolecules of extracts are present on the surface of AgNPs ( Citation17).
3.4. Colorimetric sensing of mercury using TE-3
Colorimetric trial was accomplished to the primary detection of metal ions. (a) represents the performance of different metal ions (Cd2+, Fe2+, Co2+, Pb2+ , Ni2+, Mn2+, Hg2+) with synthesized AgNPs and absorption spectra were chronicled. (c) displays the color change side view of various metal ions (10−3 M) by the addition of green synthesized AgNPs (TE-3). The brown color of green synthesized AgNPs turned into colorless with Hg2+ among the other metal ions which corroborated the selective sensing of Hg2+ ion. In addition, the colorimetric sensing was also tested by using a paper-based device. (b) represents that the color of AgNPs was quickly envisaged through naked eye within a few seconds. It is clear from the absorption spectra as well as color of green synthesized AgNPs that the green synthesized AgNPs are extremely selective to mercury ion over other metal ions. Moreover, the selectivity of Hg2+ ion with AgNPs was analyzed using absorption spectra and naked eye. (b) represents that the color of AgNPs deviates from the variation of concentration of Hg2+ ion. The absorption spectra of AgNPs with different concentrations of Hg2+ ion (100.1 µ M) are shown in (a). It is clear from the spectra that by increasing the concentration of Hg2+ ions, the absorption spectra decrease simultaneously. Electrochemical series could be used for the explanation of colorimetric sensing mechanism of Hg2+ ion. According to the electrochemical series, metals with higher electrochemical reduction potential act as better oxidizing agents. As we know that, the standard reduction potential of Ag+ and Hg2+ is 0.80 V and 0.92 V, respectively. (a) inset represents a good linear correlation between the net adsorption (ΔA) value and the concentration of Hg2+ over the concentration range from 0.1 to 10 µM (R2 = 0.98124). represents the comparative LOD of Hg2+ ion using green synthesized AgNPs. The results revealed that LOD obtained in the present study is the lowest as compared to that of the previously reported green synthesized AgNPs.
Figure 4. (A) UV–visible spectra of AgNPs with different metal ions (10−3 M) in aqueous solution (B) and (C) paper-based colorimetric sensing and images of selective sensing of Hg2+ ion by AgNPs in aqueous solution.
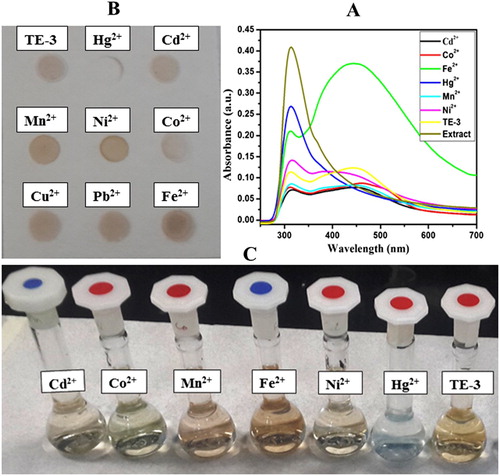
Figure 5. (A) UV–visible spectra of AgNPs with different concentrations of Hg2+ (0.1–10 µM) in aqueous solution (inset). Variation of the absorbance of AgNPs solution as a function of Hg2+ ion concentration. (B) Images of color variations of AgNPs with different concentrations of Hg2+ (10−1–10−7 M) in aqueous solution.
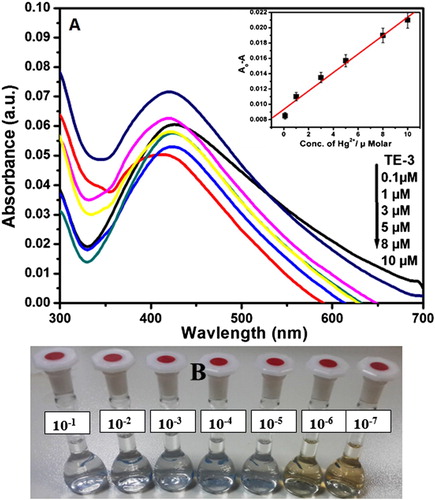
Table 1. Performance of different AgNPs for the detection of Hg2+.
3.5. Antimicrobial activity assay of TE-3
By using well diffusion methods, chemicals compounds, viz. TE-3 were examined in triplicate sets against seven of human pathogenic microbes and the results are shown in . TE-3 showed potent antimicrobial activities against all tested microbes. TE-3 revealed highest activity against C. albicans with inhibition zone (3.87 ± 0.05 cm) followed by P. aeruginosa (2.20 ± 0.35 cm) and S. flexneri (1.83 ± 0.15 cm). In addition, the least inhibition activities against S. aureus (1.5 ± 0.30 cm) and K. pneumonia (1.43 ± 0.17 cm). C. albicans is the most susceptible microbe of great importance. C. albicans can be more aggressive causing many diseases such as Candidiasis ( Citation35), mucosal infections with substantial morbidity rate ( Citation36, Citation37). TE-3 showed less inhibition to the Gram-positive bacteria strains than Gram-negative except K. pneumonia, in agreement with previous study that both types differ in their cell envelope structures, their surface charge and so on ( Citation38, Citation39). This will explain that the antimicrobial activity of TE-3 could be attributed to the variations in the structural, size and mode of actions leading to destroy the cell structure of the pathogenic microorganism.
Table 2. Inhibition zone (cm) observed for AgNPs against the test pathogenic microbes.
4. Conclusion
AgNPs caped with biomolecules have been synthesized by a simple and eco-friendly biological method at room temperature using fig stem extract. The biomolecules present in the extract act as reductant and reduced the silver ions into their corresponding metal which nucleate to form nanoparticles. The presence of the biomolecules and capping on the surface of as synthesized AgNPs was determined by FTIR spectroscopy. The main aim of the present study was to evaluate the sensing of toxic metals and antimicrobial activity against K. oxytoca, K. pneumonia, S. flexneri, P. mirabilis and P. aeruginosa, S. aureus, and C. albicans. The AgNPs showed promising colorimetric sensing with Hg2+ ion with different concentrations (10−1–10−7 M), highest inhibition activity against C. albicans with inhibition zone (3.87 ± 0.05 cm) and least inhibition activities against S. aureus (1.5 ± 0.30 cm) and K. pneumonia (1.43 ± 0.17 cm). The advantage of the prepared AgNPs could be further used as paper-based colorimetric sensor for Hg2+ and antimicrobial control system.
Acknowledgments
We are thankful to the Head of Department of Chemistry, College of Science, King Khalid University, Abha, Saudi Arabia, for providing facilities to carry out the research work.
Disclosure statement
No potential conflict of interest was reported by the authors.
Notes on contributors
Dr. Abul Kalam received his PhD degree from Allahabad Central University, India in 2003. Since 2009, he is working as Assistant Professor in the Department of Chemistry at King Khalid University, Saudi Arabia. His current research interest covers the synthesis of nano materials by chemicals and green method and its application as sensing, dye sensitized solar cell, optical band gap engineering, waste water treatment and catalysis.
Dr. Abdullah G. Al-Sehemi received his PhD degree from University of Leicester, UK in 2001. He is currently Member of the Saudi Consultative Council. He was the Dean of Scientific Research and director of RCAMS. He is an author of over 250 publications, co-inventor of several patents and the editor of 6 books. He is full Professor in the Department of Chemistry at King Khalid University, Saudi Arabia since 2011. His research interest includes energy, gas sensor, biosensor, optical band gap engineering, synthesis, characterization of organic, composite and nanocomposite materials.
Dr. Sulaiman Alrumman received his PhD from University of Aberdeen, UK in 2011. Presently, he is Dean of College of Science at King Khalid University, Saudi Arabia. He is Associate Professor in the Department of Biology, King Khalid University since 2016. His research interest covers:
Microbiological Analysis and Bioremediation of Soil and Contaminated Water.
Biodiversity of Microbes and Methods of Isolation and Characterization of Bacteria, Yeasts and Fungi.
Evaluation of the Quality of Environmental Media using Microbial Indicators.
Studying the Biological Activities of Medicinal Plants.
Field and Survey Studies of Birds.
Dr. Mohammed Assiri is Assistant Professor in the Department of Chemistry, Faculty of Science, King Khalid University, Abha, KSA since 2017. He received his PhD degree from University of Wyoming, Laramie, WY, USA in 2016. His research interests in green chemistry, sensing and capturing of toxic materials.
Dr. Mahmoud Fawzy Mahmoud Moustafa is a Professor in the Department of Biology, Faculty of Science, King Khalid University, Abha, KSA. He received a B.S. from Assuit University in 1991, and his M.S. from South Valley University, Egypt in 1996 in the field of Plant Taxonomy. He received his Ph. D. in higher plants molecular cell biology, September 2004, Laboratory of Molecular Cell Biology, Dept. Bio-Resources, College of Agricultural Sciences, Japan. His research interests in plant taxonomy, plant biotechnology, weeds Flora, weeds biological activities and medicinal plants. He published many publications in high ranked journals about 44 papers as well as he attended many international conferences all over the world. He supervised many master and Ph. D. students.
Dr. Pankaj Yadav is an Assistant Professor at Pandit Deendayal Petroleum University (PDPU), Gujarat, India. Prior to joining PDPU, he worked as a Swiss Excellence fellow with Prof. Michael Gratezel at Ecole Polytechnique Federale de Lausanne, Switzerland. Dr. Yadav is a recipient of many prestigious awards including Fulbright fellowship, Swiss Excellence fellowship, overseas postdoctoral fellowship, etc.At PDPU, Dr. Pankaj is exploring the fundamentals and applications of different materials for various energy harvesting and storage devices. His current research interests ranges from thin film devices, solar photovoltaic, perovskite solar cells, hydrogen generation, fuel cells, sensor, energy generation and storage devices, and electroanalytical characterizations.
Dr. Mehboobali Pannipara received his PhD from King Abdulaziz University, Saudi Arabia in 2014. Currently, he work as Assistant Professor of the Chemistry Department at King Khalid University, Saudi Arabia since September 2015. His research interest covers developing new synthetic methods for the synthesis of novel stimuli responsive molecular materials, synthesis of novel fluorescent materials and its application for as sensor and NL.
Additional information
Funding
References
- Nie, S.; Emory, S.R. Science 1997, 275, 1102–1106. doi: 10.1126/science.275.5303.1102
- Jana, N.R.; Sau, T.K.; Pal, T. J. Phys. Chem. B. 1999, 103, 115–121. doi: 10.1021/jp982731f
- Xue, X.; Wang, F.; Liu, X. J. Mater. Chem. 2011, 21, 13107–13127. doi: 10.1039/c1jm11401h
- Meng, H.; Liong, M.; Xia, T.; Li, Z.X.; Ji, Z.X.; Zink, J.I.; Nel, A.E. ACS Nano 2010, 4, 4539–4550. doi: 10.1021/nn100690m
- Mohanpuria, P.; Rana, N.K.; Yadav, S.K. J. Nanopart. Res. 2008, 10, 507–517. doi: 10.1007/s11051-007-9275-x
- Lara, H.H.; Ayala-Nunez, N.V.; Ixtepan-Turrent, L.; Rodriguez-Padilla, C. J. Nanobiotechnol. 2010, 8, 1–10. doi: 10.1186/1477-3155-8-1
- Passos, M.L.; Costa, D.; Lima, J.L.; Saraiva, M.L.M. Talanta 2015, 133, 45–51. doi: 10.1016/j.talanta.2014.04.089
- Mi, F.L.; Wu, S.J.; Zhong, W.Q.; Huang, C.Y. Phys. Chem. Chem. Phys. 2015, 17, 21243–21253. doi: 10.1039/C4CP05012F
- Sotiriou, G.A.; Pratsinis, S.E. Environ. Sci. Technol. 2010, 44, 5649–5654. doi: 10.1021/es101072s
- Ramanathan, R.; O'Mullane, A.P.; Parikh, R.Y.; Smooker, P.M.; Bhargava, S.K.; Bansal, V. Langmuir 2011, 27, 714–719. doi: 10.1021/la1036162
- Dang, M.C.; Dang, T.M.D.; Fribourg-Blanc, E. Adv. Nat. Sci. Nanosci. Nanotechnol. 2015, 6, 015003–015011. doi: 10.1088/2043-6262/6/1/015003
- Tien, D.C.; Tseng, K.H.; Liao, C.Y.; Huang, J.C.; Tsung, T.T. J. Alloys Compd. 2008, 463, 408–411. doi: 10.1016/j.jallcom.2007.09.048
- Ethiraj, A.S.; Jayanthi, S.; Ramalingam, C.; Banerjee, C. Mater. Lett. 2016, 185, 526–529. doi: 10.1016/j.matlet.2016.07.114
- Christensen, L.; Vivekanandhan, S.; Misra, M.; Mohanty, A.K. Adv. Mater. Lett. 2011, 2, 429–434. doi: 10.5185/amlett.2011.4256
- Singh, R.; Shedbalkar, U.U.; Wadhwani, S.A.; Chopade, B.A. Appl. Microbiol. Biotechnol. 2015, 99, 4579–4593. doi: 10.1007/s00253-015-6622-1
- Durán, M.; Silveira, C.P.; Durán, N. IET Nanobiotechnol. 2015, 9, 314–323. doi: 10.1049/iet-nbt.2014.0054
- Duke, J.A.; Bugenschutz-godwin, M.J.; Du collier, J.; Duke, P.K. Hand Book of Medicinal Herbs, 2nd ed. CRC Press: Boca Raton, FL, 2002.
- Werbach, M. Healing with Food; Harper Collins: New York, NY, 1993.
- Ulug, B.; Turkdemir, M.H.; Cicek, A.; Mete, A. Spectrochim. Acta Part A 2015, 135, 153–161. doi: 10.1016/j.saa.2014.06.142
- Kalam, A.; Al-Sehemi, A.G.; Alrumman, S.; Du, G.; Pannipara, M.; Assiri, M.; Almalki, H.; Moustafa, M.F. J. Fluoresc. 2017, 27, 2045–2050. doi: 10.1007/s10895-017-2143-x
- Jacob, S.J.P.; Prasad, V.L.S.; Sivasankar, S.; Muralidharan, P. Food Chem. Toxicol. 2017, 109, 951. doi: 10.1016/j.fct.2017.03.066
- Moustafa, M.F.; Alrumman, S.A. Pak. J. Pharm. Sci. 2015, 28, 2009–2017.
- Alrumman, S.A. Arab. J. Sci. Eng. 2016, 41, 2123–2129. doi: 10.1007/s13369-015-1900-x
- Ahmed, M.J.; Murtaza, G.; Mehmood, A.; Bhatti, T.M. Mater. Lett. 2015, 153, 10–13. doi: 10.1016/j.matlet.2015.03.143
- Jeeva, K.; Thiyagarajan, M.; Elangovan, V.; Geetha, N.; Venkatachalam, P. Ind. Crops Prod. 2014, 52, 714–720. doi: 10.1016/j.indcrop.2013.11.037
- Jeyaraj, M.; Rajesh, M.; Arun, R.; Ali, D.M.; Sathishkumar, G.; Sivanandhan, G.; Dev, G.K.; Manickavasagam, M.; Premkumar, K.; Thajuddin, N.; Ganapathi, A. Colloids Surf. B 2013, 102, 708–717. doi: 10.1016/j.colsurfb.2012.09.042
- Zargar, M.; Shameli, K.; Najafi, G.R.; Farahani, F. J. Ind. Eng. Chem. 2014, 20, 4169–4175. doi: 10.1016/j.jiec.2014.01.016
- Shameli, K.; Ahmad, M.B.; Shabanzadeh, P.; Al-Mulla, E.A.J.; Zamanian, A.; Abdollahi, Y. Res. Chem. Intermed. 2014, 40, 1313–1325. doi: 10.1007/s11164-013-1040-4
- Basiri, S.; Mehdinia, A.; Jabbari, A. Spectrochim. Acta Part A 2017, 171, 297–304. doi: 10.1016/j.saa.2016.08.032
- Mo, Y.Y.; Tang, Y.K.; Wang, S.Y.; Lin, J.M.; Zhang, H.B.; Luo, D.Y. Mater. Lett. 2015, 144, 165–167. doi: 10.1016/j.matlet.2015.01.004
- Sánchez, G.R.; Castilla, C.L.; Gómez, N.B.; García, A.; Marcos, R.; Carmona, E.R. Mater. Lett. 2016, 183, 255–260. doi: 10.1016/j.matlet.2016.07.115
- Yang, N.; Wei, X.-F.; Li, W.-H. Mater. Lett. 2015, 154, 21–24. doi: 10.1016/j.matlet.2015.03.034
- Raghavendra, G.M.; Jung, J.; kim, D.; Seo, J. Int. J. Biol. Macromol. 2016, 86, 126–128. doi: 10.1016/j.ijbiomac.2016.01.057
- Raja, S.; Ramesh, V.; Thivaharan, V. J. Ind. Eng. Chem. 2015, 29, 257–264. doi: 10.1016/j.jiec.2015.03.033
- Gozalbo, D.; Roig, P.; Villamón, E.; Gil, M.L. Curr. Drug Targets 2004, 4, 117–135. doi: 10.2174/1568005043341046
- Weidong, Z.; Scott, G.F. Cell Microbiol. 2010, 12, 273–282. doi: 10.1111/j.1462-5822.2009.01412.x
- Villar, C.C.; Kashleva, H.; Nobile, C.J.; Mitchell, A.P.; Dongari-Bagtzoglou, A. Infect. Immun. 2007, 75, 2126–2135. doi: 10.1128/IAI.00054-07
- Shrivastava, S.; Bera, T.; Roy, A.; Singh, G.; Ramachandrarao, P.; Dash, D. Nanotechnology 2007, 18, 22225103–225111. doi: 10.1088/0957-4484/18/22/225103
- Tamboli, D.P.; Lee, D.S. J. Hazard. Mater. 2013, 260, 878–884. doi: 10.1016/j.jhazmat.2013.06.003
- Ravi, S.S.; Christena, L.R.; Subramanian, N.S.; Anthony, S.P. Analyst 2013, 138, 4370. doi: 10.1039/c3an00320e
- Laghi, L.; Parpinello, G.P.; Rio, D.D.; Calani, L.; Mattioli, A.U.; Versari, A. Food Chem. 2010, 121, 783. doi: 10.1016/j.foodchem.2010.01.002
- Farhadia, K.; Forougha, M.; Molaeia, R.; Hajizadeha, S.; Rafipourb, A. Sens. Actuators B 2012, 161, 880. doi: 10.1016/j.snb.2011.11.052
- Alam, M.N.; Chatterjee, A.; Das, S.; Batuta, S.; Mandalb, D.; Begum, N.A. RSC Adv. 2015, 5, 23419. doi: 10.1039/C4RA16984K