ABSTRACT
The aqueous extract of Adiantum capillus-veneris L was used as a reducing agent in the batch method for the synthesis of silver nanoparticles (AgNPs). For improving AgNP synthesis, the concentrations of metal ions solution and leaf extract were optimized in 24 h. Using Fourier transform infrared spectroscopy (FTIR), powder X-ray diffraction (XRD), transmission electron microscopy (TEM), and UV-Vis spectroscopy, AgNPs were characterized. In the UV-Vis spectrum, the peak was observed at 453 nm, indicating the surface plasmon resonance (SPR) of AgNPs. The purity and crystalline nature of AgNPs were confirmed based on the XRD assessment. In addition, specific functional groups, which led to silver nitrate reduction in AgNP formation, were examined using FTIR. According to TEM, the average particle size was 18.4 nm in AgNPs. Antibacterial activity assays with Escherichia coli and Staphylococcus aureus demonstrated that AgNPs reduced bacterial growth and produced well-defined inhibition zones.
GRAPHICAL ABSTRACT
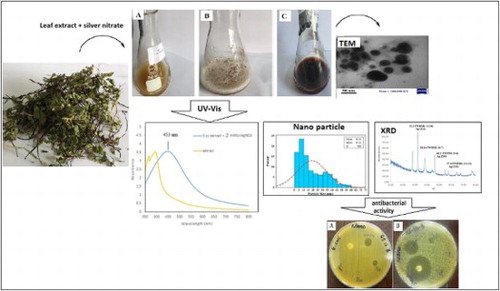
Introduction
Nanotechnology development and research have grown rapidly around the world (Citation 1,Citation 2). Today, AgNPs are recognized as commonly used NPs with a variety of applications (Citation 3). They are used as optical receptors, coating agents in solar energy absorption, and biological labels (Citation 4).
For the synthesis of metal NPs, different metals including Ag, Pd, Zn, Au, and CdS have been applied (Citation5–7). Among metal NPs, AgNPs show significant physical, chemical, and biological properties (Citation 8), with potential antimicrobial and anticancer applications in different industries (e.g. textile, food packaging, and cosmetic industries) (Citation9–12). Various chemical, physical, and biological approaches have been examined for the synthesis of AgNPs (Citation 13). Although chemical synthesis is generally a rapid process, it necessitates the use of toxic chemicals, such as NaBH4, N2H4, NH2OH, and ethanol (Citation 3,Citation 14). Moreover, control of the growth, stability, and aggregation of particles is difficult through chemical synthesis, and for stabilizing the NP size, use of capping agents is necessary (Citation 3). In recent years, use of plant extracts has been highlighted for NP synthesis. Considering its nontoxicity, simplicity, and large-scale production, application of biosynthetic green metal NPs is growing (Citation 1,Citation15–19). Various parts of plants, including the stem, root, leaf, flowers, and fruits, contain phytochemical substances and function as stabilizing and reducing agents during NP formation (Citation 20). Recently, extracts of various plant such as Nigella sativa (Citation 3), Tragia involucrata (Citation 4), Ulva flexuosa (Citation 9), Achillea biebersteinii (Citation 15), Lonicera japonica (Citation 16), pennyroyal (Citation 21), olive (Citation 22), Acalypha indica (Citation 23), Azadirachta indica (Citation 24), Morinda pubescens (Citation 25), tea (Citation 26), and Rosmarinus officinalis have been reported to aid in the formation of NPs ranging in size from 10 to 33 nm (Citation 27), and have been further explored as a source for the synthesis of AgNPs. Also previous studies have been reported synthesis of silver nanoparticles via Adiantum capillus-veneris L (Citation 28). Active compounds found in Adiantum capillus-veneris L include flavonoids, terpenoids, phenolic acids, alkaloids, fiber (Citation 29,Citation 30). In our rapid and simple method, Adiantum capillus-veneris L leaf extract was used for the synthesis of AgNPs as reducing and capping agents under normal atmospheric conditions in the batch reactor. This is a report on Adiantum capillus-veneris L-assisted synthesis of AgNPs in large-scale, prepared using low amounts of leaf extracts, without requiring any extra compounds or physical processes.
In many countries, the significant biological activity, extensive availability, and easy collection of Equisetum arvense have led to its medicinal use. Recently, various antimicrobial activities have been attributed to Adiantum capillus-veneris L (Citation 31). Use of the extracts of Adiantum capillus-veneris L does not require any intermediate base groups and is considered cost-effective. In addition, it is not time-consuming, does not require expensive precursors or equipment, and yields a pure product without impurities.
Adiantum capillus-veneris L is recognized as the best source of NP synthesis, as it leads to large-scale production of stable NPs in different sizes and shapes. The leaf extract and metal solution concentrations were optimized to improve AgNP synthesis. AgNPs were characterized using several types of spectral analysis. For evaluating the antibacterial activity of silver NPs, the disc diffusion method was applied.
Materials and methods
Preparation of Adiantum capillus-veneris L aqueous extract
Green Adiantum capillus-veneris L leaves were collected from North of Iran in June 2016. After washing the leaves, they were dried under indirect light for 7 days and ground into powder; the powder was stored at a temperature of 25°C. Merck Co. provided AgNO3 (99.80%). By adding double-distilled water, the aqueous solution was prepared. Adiantum capillus-veneris L leaf powder (50 g) was incubated for 15 min in a 70°C water bath. Afterwards, the extract was centrifuged for 15 min at 4000 rpm to eliminate heavy biomaterials.
Synthesis of silver nanoparticles
The Adiantum capillus-veneris L aqueous extract was mixed with 1, 2, or 3 mM AgNO3 (1000 mL), at 25°C for 24 h via mechanical Stirring at 750 rpm. At room temperature, the color changed from yellow to dark brown.
Purification of silver nanoparticles
AgNPs were collected gradually during the reaction. For the removal of silver colloid residues, centrifugation was carried out for 15 min using double-distilled water at 4000 rpm; then, the solution was rinsed with deionized water three times. For further characterization, the dried AgNP powder was collected following incubation for three hours at 65°C (Citation 32,Citation 33).
Ultraviolet–visible (UV–Vis) absorption spectroscopy
Formation of AgNPs was monitored at 300–800 nm, based on the UV-Vis spectra of the reaction medium. For recording the spectra, a UV-Vis spectrophotometer (BioTek) was employed. Before every measurement, the samples were diluted in water.
Fourier transform infrared (FTIR) spectroscopy
Spectroscopy was performed to determine the functional groups in AgNP synthesis, which were bound distinctively to the AgNP surface. Before and after AgNP synthesis, the Adiantum capillus-veneris L extract was vacuum-dried to prepare the samples. Afterwards, the dried samples were mixed with potassium bromide and pressed into a sheer slice. An FTIR spectrometer (Perkin Elmer, USA) was used to examine the samples.
X-ray diffraction (XRD)
The crystal structure of AgNPs was determined and confirmed via XRD analysis. After placing the air-dried NPs on an XRD grid, they were assessed in terms of AgNP formation, using an X-ray diffractometer (Cu Kα radiation, θ–2θ configuration; PANalytical) with an X’Pert Pro generator (30 mA; 40 kV). For measuring the crystallite domain size, the XRD peak width was determined under the assumption that non-uniform strains are absent. The Scherrer equation was calculated as follows (Equation 1):where D denotes the average crystallite domain size perpendicular to the reflecting planes, λ presents the X-ray wavelength, β represents the full width at half maximum (FWHM), and θ indicates the diffraction angle.
Transmission electron microscopy (TEM)
A Zeiss TEM microscope (EM 10C/CR, Zeiss, Germany) was used for the morphological assessment of AgNPs at 100 kV. After drop-casting the suspension on a carbon-coated copper grid, it was left to dry overnight at 25°C.
Antibacterial activity
For determining the antibacterial activity of AgNPs, agar disk diffusion was used according to the literature (Citation34–37). Briefly, using broth dilution, AgNPs at 5, 0.5, and 0.05 μg/mL were used to measure the minimum bactericidal concentration for preventing Escherichia coli (ATCC 25922) and Staphylococcus aureus (ATCC 25923) growth. Bacterial cultures at a concentration of 1.5 × 108 CFU/mL were inoculated with different concentrations of AgNPs. After incubating the culture plates at 37°C for 24 h, the bacterial growth inhibition zone was measured in centimeters. For each bacterial strain, three independent experiments were performed (Citation 38,Citation 39).
Results and discussion
To investigate whether an aqueous extract of Adiantum capillus-veneris L can be used in the green synthesis of AgNPs, we combined the extract with 1, 2 and 3 mM of AgNO3 for 24 h. The slow change of color in the solution (from yellow to dark brown) was indicative of AgNP formation. The dark brown color might be related to the surface plasmon resonance (SPR) effect and AgNO3 reduction (Citation 40).
UV–VIS spectroscopy
UV–Vis spectroscopy is described as a suitable tool for NP assessment, especially in stability analysis of metal NPs in aqueous solutions. To determine if the reaction had reached completion, different concentration of AgNO3 (1, 2 and 3 mM) was reacted with different concentration of the aqueous extract (5, 10 and 15 mL), and the spectra were recorded at a range of 300–800 nm (Citation 41); then, the wavelength of maximum absorption was identified (). Considering the color changes after the addition of AgNO3, AgNP biosynthesis by the Adiantum capillus-veneris L extract was confirmed.
Figure 1. UV visible absorption spectrum showing (a) Optimum AgNO3 concentration, (b) Optimum concentration of Adiantum capillus-veneris L extract.
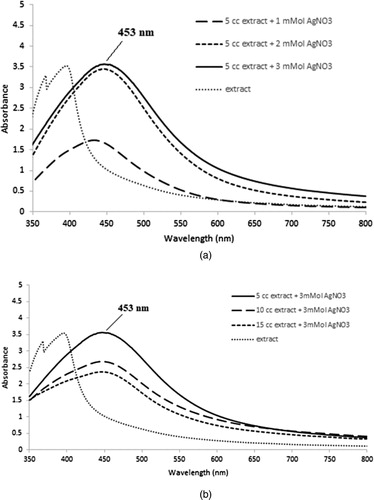
According to the analysis, a sharp SPR related to 3 mM AgNO3 and 5 mL aqueous extract was observed at nearly 453 nm (), which is in line with reports of peaks in the absorption spectra of 400–500 nm associated with SPR in AgNPs (Citation 14,Citation42–44). The NPs were dispersed without using any chemical or physical capping agents. AgNPs synthesized with Adiantum capillus-veneris L extract showed stability in the solution. The reported size of these synthesized AgNPs was smaller than many earlier green synthesis reports (Citation 43,Citation 45).
XRD analysis
Using the XRD pattern of synthesized AgNPs, distinctive peaks were identified, indexed to the crystalline planes of silver (); these results conform to the JCPDS reports (No, 04-0783). The crystalline nature of synthesized AgNPs was confirmed considering the sharp peaks (Citation
4). Intense NP peaks of (1 1 1), (2 0 0), (2 2 0), and (3 1 1) emerged, indexed as the crystalline silver face-centered-cubic (FCC) phase (Citation
46). The mean particles size of AgNPs was evaluated using the Debye–Scherer Equation 1(Equation 1):where λ denotes the X–ray wavelength (1.540560 Å), β represents the width of XRD peak, θ shows the Bragg angle, d refers to the size of particle, and K describes the Scherer constant (0.9) (Citation
14,Citation
47). The average size of AgNPs was almost around 25 nm.
FTIR analysis
For determining the biomolecules, resulting in AgNP reduction and stabilization, FTIR analyses were performed. In , the FTIR peaks of dried Adiantum capillus-veneris L and AgNPs are presented (Citation 4). The FTIR results revealed absorption bands at 3396.66, 1625.75, and 1427.55 cm−1 ((a)). The vibrational bands corresponded to bonds of aminos (=N−H), alcohols (−O−H), alkenes (>C=C), flavonoids, and amines (−NH2), all in the range of 800–3442 cm−1. Bonded hydroxyl (−OH) groups of carboxylic acids and phenols could produce absorption bands at 3396.66 cm−1 ((a)). In the AgNP spectrum, the new band at 1607.23 cm−1 might suggest a new C=O group (a ketone or an aldehyde) ((b)).
Figure 4. (a) FTIR spectra of Adiantum capillus-veneris L extract, (b) Silver nanoparticle synthesized using Adiantum capillus-veneris L extract.
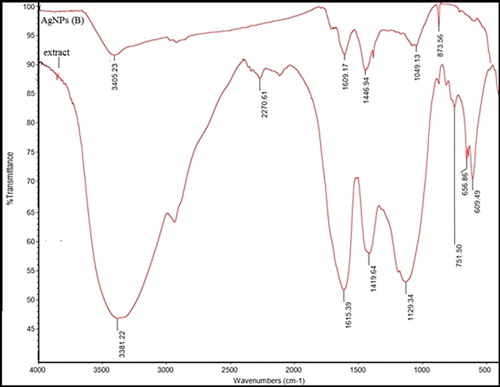
For identifying biomolecules, which may be involved in the capping and reduction of synthesized AgNPs, FTIR measurements were performed. The FTIR spectra of the extract indicated absorption bands, corresponding to functional groups including phenols, alcohol, amines, and carbonyls. This strongly suggests that hydroxyl groups reduce Ag+ and are oxidized to carbonyl groups in the process () (Citation 48).
Figure 5. Mechanism of Ag NPs formation from flavonoids and polyphenols present in the leaf extract of Adiantum capillus-veneris L.
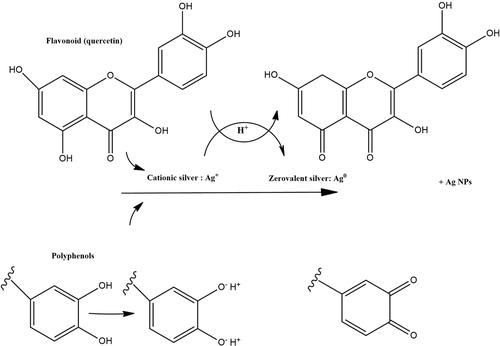
AgNPs show significant binding affinity to multiple functional groups in the compounds of plants (e.g. polyphenolics and proteins); a layer of these functional groups covers AgNPs. They act as capping agents, providing stability and preventing AgNP agglomeration, as confirmed in the green synthesis of AgNPs with other plant extracts (Citation 49,Citation 50). These findings confirm that phenolic agents in the Adiantum capillus-veneris L extract are responsible for the reduction of AgNO3.
TEM analysis
TEM imaging was applied to measure AgNP size and surface morphology in the solution. As shown in (a), the TEM study demonstrated that the average size was 18.4 nm with interring particle distance. The morphology of Ag nanoparticles was also spherical ((a)). In another work, Devaraj (Citation 51) synthesized AgNPs from Diospyros montana extract and obtained AgNPs in variable size and morphology.
Antibacterial activity
For evaluating the antibacterial activity of AgNPs and aqueous Adiantum capillus-veneris L extract, both Gram-positive (S. aureus) and Gram-negative (E. coli) bacteria were used ( and ).
Figure 7. Antibacterial activity of AgNPs against (a) Gram negative (E. coli), (b) Gram positive (S.aureus) in different solutions.
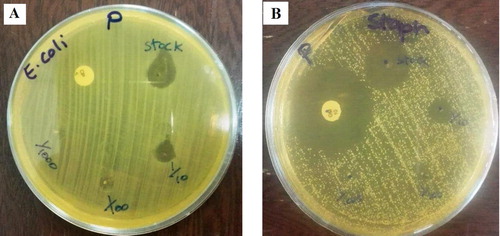
Figure 8. Antibacterial activity of Adiantum capillus-veneris L extract against (a) Gram negative (E. coli), (b) Gram positive (S.aureus) in different solutions.
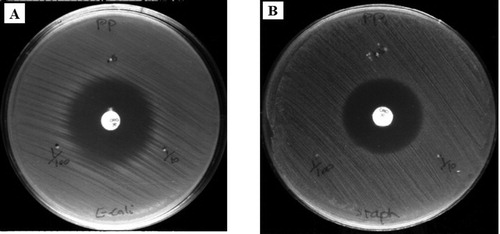
The maximum inhibition zone in a highly concentrated solution (stock AgNPs) is listed in . Also, the zone inhibition of two antibiotics Ciprofloxacin and Gentamicin was compared with AgNPs (Citation 52). AgNPs were as effective as Ciprofloxacin and Gentamicin. Also, silver not only have a close interaction with the surface of the membrane, but can also penetrate deep inside the bacteria. According to some studies, the antimicrobial activity of silver is relatively better against Gram-negative bacteria, compared to Gram-positive bacteria; this may be related to the thinner layer of peptidoglycans and beta barrel proteins (porins) in the cell wall structure (Citation 53). This mechanism is classified broadly as the direct effects of NPs on microbial cells. The cell wall becomes permeable to the extracellular medium as soon as the cell membrane is damaged, and bacterial cells become susceptible to damage (Citation 54).
Table 1. Antibacterial activity of AgNPs in dose of (5, 0.5 and 0.05 μg/mL).
Conclusion
The biosynthesis of stable AgNPs from an aqueous AgNO3 solution was revealed, using Adiantum capillus-veneris L as the reducing and capping agent. Also this method is easily adopted mass production of nanoparticles without requiring any extra compounds or physical processes and have well-defined size and morphology. Optimization of AgNO3 and Adiantum capillus-veneris L extract concentration was achieved to obtain large amount of silver nanoparticles in batch process. UV–Vis, FTIR, TEM, and XRD studies were performed to confirm the AgNP formation. For AgNPs, UV–Vis peak was observed at 453 nm. The crystalline structure of synthesized AgNPs was determined using XRD analysis. The peaks in XRD patterns were associated with those of the FCC form of metallic silver. AgNPs had a quasi-spherical shape and an anisotropic nature (average size, 18 nm). The strong antimicrobial activity of biosynthesized NPs was confirmed against Gram-positive (S. aureus), as well as Gram-negative (E. coli) bacteria. The green synthesis of AgNPs with the extract is a more cost-effective and eco-friendly strategy, compared to physical and chemical synthesis methods.
Acknowledgements
This work was supported by North Tehran branch and Shahr-e-Qods Branch of Islamic Azad University of Iran. We thank these two Universities for their kind Cooperation.
Disclosure statement
No potential conflict of interest was reported by the authors.
Notes on contributors
Sariyeh Omidi received M.Sc. degree in applied chemistry from Islamic Azad University-Shahre Ray, Yadegare Emam Branch, Tehran, Iran, in 2010. She is a Ph.D. Student in Applied Chemistry in Islamic Azad University-Tehran North Branch since 2014. Her main areas of interests are Nano technology, Green chemistry and water treatment.
Sajjad Sedaghat received the M.Sc. degree in organic chemistry from Shahid beheshti University, Tehran, Iran, in 2002. He received his PhD. in Applied chemistry from Islamic azad university, north Tehran branch in nano catalyst for fuel cells in 2006. Since 2006, he has been with Islamic azad university, Shahr-e-Qods branch, Tehran, Iran as a lecturer in the green chemistry group. His main areas interests are nano synthesis, nanocomposites and Green chemistry.
Kambiz Tahvildari received M.Sc. degree in applied chemistry from Islamic Azad University-Tehran North Branch, Tehran, Iran, in 1997. He received his PhD. in Applied Chemistry from same university in 2006. Since 2006 he is chemistry faculty member of this university and his main areas of interests are Biofuels, Nano technology, Green chemistry and water treatment.
Pirouz Derakhshi received M.Sc. degree in analytical chemistry from Islamic Azad University-Tehran North Branch, Tehran, Iran, in 1993. He received his PhD. in Applied Chemistry from same university in 2009. Since 2009 he is chemistry faculty member of this university and studied in Chemical Nano- Technology.
Fereshte Motiee received M.Sc. degree in analytical chemistry from Islamic Azad University-Tehran North Branch, Tehran, Iran. He received his PhD. in Applied Chemistry from the same university. He is expertise in Material Characterization, Mechanical Properties, Thermal Analysis, Thermogravimetry, Rubber, fillers and biofillers Polymer Chemistry, Materials Chemistry and Physical Chemistry.
References
- Ballester, A.; Blázquez, M.L.; Castro, L.; González, F.; Muñoz, J.A. IET Nanobiotechnol.. 2014, 7, 109–116.
- Ahmad, M.; Ahmed, Sh.; Ikram, S.; Swami, B.L. J. Adv. Res. 2016, 7, 17–28. doi: 10.1016/j.jare.2015.02.007
- Amooaghaie, R.; Azizi, M.; Saeri, M.R. Ecotox Environ Safe. 2015, 120, 400–408. doi: 10.1016/j.ecoenv.2015.06.025
- Johnson, I.; Prabu, H.J. Karbala Int. J. Mod. 2015, 1, 237–246. doi: 10.1016/j.kijoms.2015.12.003
- Tripathi, R.M.; Gupta, R.K.; Singh, P.; Bhadwal, A.S.; Shrivastav, A. Sensor Actuat B. 2014, 204, 637–646. doi: 10.1016/j.snb.2014.08.015
- Bhadwal, A.S.; Tripathi, R.M.; Gupta, R.K.; Singh, R.P. RSC Adv.. 2014, 4, 9484–9490. doi: 10.1039/c3ra46221h
- Mohammadi, F.M.; Ghasemi, N. J Nanostruct Chem, 2018, 8 (1): 93–102. doi: 10.1007/s40097-018-0257-6
- Le, A.-T.; Nguyen, V.Q.; Tran, Q.H. Adv. Nat. Sci. 2013, 4, 033001.
- Akbarzadeh, A.; Noori, A.; Rahimi, Z.; Yousefzadi, M. J Persian Gulf (Marine Science). 2014, 5, 9–16.
- Pantic, I. Rev. Adv. Mater. Sci.. 2014, 37, 15–19.
- Afshar, P.; Sedaghat, P. Curr. Nanosci., 2015, 12, 90–93. doi: 10.2174/1573413711666150529202238
- Mohammadzadeh, R. Adv. Pharm. Bull. 2012, 2, 133–133.
- Abd-Elaal, A.A.; Negm, N.A.; Tawfik, S.M. J. Ind. Eng. Chem.. 2015, 21, 1051–1057. doi: 10.1016/j.jiec.2014.05.015
- Akhtar, M.S.; Mohanty, S.K.; Sinniah, U.R.; Swamy, M.K. Spectrochim. Acta A. 2015, 151, 939–944. doi: 10.1016/j.saa.2015.07.009
- Baharara, J.; Hosseini, N.; Namvar, N.; Ramezani, T.; Rosfarizan, M. Molecules. 2014, 19, 4624–4634. doi: 10.3390/molecules19044624
- An, M.; Lee, K.D.; Lee, S.E.; Nagajyothi, P.C. Bull. Korean Chem. Soc. 2012, 33, 2609–2612. doi: 10.5012/bkcs.2012.33.9.3048
- Carneiro, Dm.; Cunah, Lc.; Jardim, Pcbv.; Tresvenzol, Lmf. IJBPAS. 2013, 2, 1579–1596.
- Chopra, D.; Kaur, S.; Sandhu, N.S. Asia. Pac. J. Pharmacol. 2010, 3, 146–150.
- Sedaghat, S. Curr. Nanosci., 2015, 12, 79–82. doi: 10.2174/1573413711666150812010229
- Sharmila, G.; Muthukumaran, C.; Sandiya, K.; Santhiya, S.; Sakthi Pradeep, R.; Manoj Kumar, N.; Suriyanarayanan, N. J Nanostruct Chem, 2018, 8, 293–299. doi: 10.1007/s40097-018-0271-8
- Al-Harbi, L.M.; Al-Thabaiti, SH.A.; El-Mossalamy, E.H.; Khan, Z.; Obaid, A.Y. Arab J Chem. 2015. http://doi.org/10.1016/jarabjc.2014.12.035.
- El-Baghdady, K.H.Z.; Ismail, E.H.; Khalil, M.H.M.; Mohammad, D. Arab J Chem, 2014, 7, 1131–1139. doi: 10.1016/j.arabjc.2013.04.007
- Jagan, E.G.; Kalaichelvan, P.T.; Krishnaraj, C.; Mohan, N.; Rajasekar, S.; Selvakumar, P. Colloids Surf., B. 2010, 76, 50–56. doi: 10.1016/j.colsurfb.2009.10.008
- Ahmad, M.; Ahmed, SH.; Ikram, S.; Saifullah, SH.; Swami, B.L. J. Radiat. Res. App. Sci. 2016, 9, 1–7. doi: 10.1016/j.jrras.2015.06.006
- Inbathamizh, L.; Mary, E. Asian J Pharm Clin Res. 2012, 5, 159–162.
- Cai, X.; Li, J.; Sun, Q.; Yu, C.P. Colloids Surf., A. 2014, 444, 226–231. doi: 10.1016/j.colsurfa.2013.12.063
- Ghaedi, M.; Purkait, M.K.; Safarpoor, M.; Yousefinejad, M.; Zare Khafri, H. J. Ind. Eng. Chem. 2015, 31, 167–172. doi: 10.1016/j.jiec.2015.06.020
- Santhoshkumar, S.; Nagarajan, N. IJPSR. 2014, 5(12), 5511–5518.
- Rajurkar, N.S.; Gaikwad, K. J. Chem. Pharm. Res., 2012, 4(1), 365–374.
- Ansari, R.; Ekhlasi-Kazaj, K. J Adv Sci Res., 2012, 3 (4), 15–20.
- Pradeep, P.; Parihar, L.; Bohra, A. J. Microbiol. Antimicrob. 2010, 2 (2), 19–22.
- Bagheriyan, S.; Esmaeili, A.A.; Sedaghat, S. J. Nanostructure Chem. 2016, 6, 25–27. doi: 10.1007/s40097-015-0176-8
- Fathima, G.; Illiyas, M.; Kalyanaraman, R.; Krishnan, Th.; Krishnan, V.; Rather, H.M.; Sheriff, KH.; Thangam, R. World J Pharm Pharm Sci. 2015, 4, 723–733.
- Allafchian, A.R.; Mirahmadi-Zare, S.Z.; Jalali, S.A.H.; Hashemi, S.S.; Vahabi, M.R. J Nanostruct Chem. 2016, 6, 129–135. doi: 10.1007/s40097-016-0187-0
- Shrivastava, S.; Bera, T.; Roy, A.; Singh, G.; Ramachandrarao, P.; Dash, D. Nanotechnol. 2007, 18, 225103. doi: 10.1088/0957-4484/18/22/225103
- Kuppurangan, G.; Karuppasamy, B.; Nagarajan, K.; Krishnasamy Sekar, R.; Viswaprakash, N.; Ramasamy, Th. Appl. Nanosci.. 2016, 6, 973–982. doi: 10.1007/s13204-015-0514-7
- Chuah, E.L.; Zakaria, Z.A.; Suhaili, Z.; Abu Bakar, S.; Desa, M.N.M. J. Microbiol. Res, 2014, 4, 6–13.
- Das, B.; Dash, S.K.; Mandal, D.; Ghosh, T.; Chattopadhyay, S.; Tripathy, S.; Das, S.; Dey, S.K.; Das, D.; Roy, S. Arab. J. Chem. 2015, 8, 1–15. doi: 10.1016/j.arabjc.2014.07.005
- Huang, J.; Zhan, G.; Zheng, B.; Sun, D.; Fenfen, L.; Yuan, L.; Chen, H.; Zheng, Zh.; Zheng, Y.; Qingbiao, L. Ind. Eng. Chem. Res.. 2011, 50, 9095–9106. doi: 10.1021/ie200858y
- Ahmad, M.; Azizi, S.; Mahdavi, M.; Mohamad, R.; Namvar, F. Materials. (Basel). 2013, 6, 5942–5950. doi: 10.3390/ma6125942
- Muniyappan, N.; Nagarajan, N.S. Process Biochem.. 2014, 49, 1054–1061. doi: 10.1016/j.procbio.2014.03.015
- da Cordeiro, T.S.; De Matos, A.; Samad, R.E.; Vieira, L.C., Jr., N.D. Colloids Surf., A. 2011, 389, 134–137. doi: 10.1016/j.colsurfa.2011.08.040
- Dipankar, C.; Murugan, S. Colloids Surf., B. 2012, 98, 112–119. doi: 10.1016/j.colsurfb.2012.04.006
- Ghaffari-Moghaddam, M.; Hadi-Dabanlou, R. J. Ind. Eng. Chem.. 2014, 20, 739–744. doi: 10.1016/j.jiec.2013.09.005
- Khan, Sh,; Saleh, T.A.; Wahab, A.; Khan, M.H.; Khan, D.; Khan, W.; Rahim, A.; Kamal, S.; Khan, F.; Fahad, Sh. Int. J. Nanomed, 2018, 13, 733–762. doi: 10.2147/IJN.S153167
- Baghizadeh, A.; Ranjbar, Sh; Kumar Gupta, V.; Asif, M.; Pourseyedi, Sh; Karimi, M.J.; Mohammadinejad, R. Green Synthesis of Silver Nanoparticles using Seed Extract of Calendula Officinalis in Liquid Phase. J. Mol. Liq. 2015, 207, 159–163. doi: 10.1016/j.molliq.2015.03.029
- Almashhedy, L.A.; Thamer, N.A. Int J Pharm Bio Sci. 2014, 5 (B), 759–770.
- Adil, SF.; Khan, M.; Tahir, M.N. Int. J. Nanomedicine. 2013, 8, 1507–1516.
- Aromal, S.A.; Philip, D.; Vidhu, V.K. Spectrochim. Acta A. 2011, 83, 392–397. doi: 10.1016/j.saa.2011.08.051
- Bapat, V.A.; Jagtap, U.B. Ind. Crop. Prod. 2013, 46, 132–137. doi: 10.1016/j.indcrop.2013.01.019
- Bharathi, D.; Josebin, M.D.; Vasantharaj, S.; Bhuvaneshwari, V. J Nanostruct Chem, 2018, 8 (1), 83–92. doi: 10.1007/s40097-018-0256-7
- Omidi, S.; Sedaghat, S.; Tahvildari, K.; Derakhshi, P.; Motiee, F. J Nanostruct Chem, 2018, 8, 171–178. doi: 10.1007/s40097-018-0263-8
- Zewde, B.; Ambaye, A.; Stubbs, J. III; Dharmara, R. JSM Nanotechnol Nanomed, 2016, 4 (2), 1043.
- Mohammadlou, M.; Maghsoudi, H.; Jafarizadeh-Malmiri, H. IFRJ. 2016, 23 (2), 446–463.