ABSTRACT
This paper describes the design and implementation of a minimally hazardous, environmentally friendly, and energy efficient sequential reaction sequence within a sophomore level Organic Chemistry lab course to efficiently synthesize N-phenylmaleimide precursors for a Diels–Alder reaction. Substituted N-phenylmaleimides are a class of very expensive precursors of considerable interest due to their biological properties and use as intermediates in synthesis. The synthesis described herein produces a substituted N-phenylmaleimide in two steps from maleic anhydride and a substituted aniline followed by its Diels–Alder reaction with 2,5-dimethylfuran. The experiment exposes students to the green chemistry principles of atom economy, use of safer chemicals, design for energy efficiency, waste reduction, and inherently safer chemistry for accident prevention and enables students to use 1H NMR spectroscopy to characterize the products.
GRAPHICAL ABSTRACT
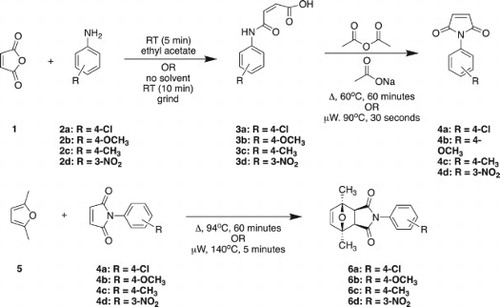
Introduction
The multi-step synthesis of natural and non-natural products is a vital field of Organic Chemistry ( Citation1–4). The “art of organic synthesis” demonstrated by Woodward, Nicolau, and others to produce molecules for use in the pharmaceutical, agricultural, biochemical, and other industries demonstrates the ability of organic chemists to transform bonds and functional groups to produce molecules with desired properties. The teaching of synthetic skills and problem-solving has long been part of the organic chemistry lecture and laboratory curriculum. The commitment of faculty to incorporating synthesis into the curriculum is evident in a large number of multi-step syntheses in Mayo’s classic laboratory textbook ( Citation5) and the multitude of synthesis experiments found in the literature. These experiments provide a variety of approaches to teaching synthesis in the sophomore organic chemistry curriculum with most of them focusing on the synthesis of a biologically ( Citation6–17) or commercially ( Citation18–24) relevant target while some are designed for pedagogical ( Citation25–37) reasons. The complexity of the syntheses differ greatly and range from two ( Citation6, Citation7, Citation11–Citation13, Citation16, Citation18, Citation20, Citation22, Citation27, Citation31, Citation36), three ( Citation8, Citation10, Citation14, Citation15, Citation17, Citation21, Citation23, Citation25, Citation28–Citation30, Citation34, Citation37), four ( Citation9, Citation26, Citation33, Citation38), five ( Citation19, Citation32), six ( Citation24, Citation39), and eight ( Citation40) step processes. A number of the experiments engage the sophomore organic students in research projects that expose students to the problem-solving skills necessary to optimize and trouble-shoot a multi-step synthesis ( Citation38–41) while a couple of experiments ask students to design a synthesis ( Citation42, Citation43).
Since the publication of Green Chemistry: Theory and Practice by Anastas and Warner 20 years ago ( Citation44), there has been a multitude of experiments published to illustrate green chemistry through the redesign of organic reactions ( Citation45, Citation46). However, there have been many fewer multi-step synthesis experiments published that incorporate green chemistry principles into the synthetic design ( Citation47–55). Three of these green syntheses are accomplished without isolation and purification of the intermediate products ( Citation49, Citation53, Citation55). Several of the experiments are designed to introduce students to multi-step synthesis using commonly discussed sophomore organic chemistry reactions ( Citation48, Citation51, Citation52, Citation54). The other experiments accomplish this goal while exposing the students to the synthesis of a biologically and/or commercially important compound ( Citation47, Citation49, Citation50, Citation53, Citation55).
We set out to develop a multi-step green synthesis for the sophomore organic chemistry course that produces a commercially valuable product and utilizes a Diels–Alder reaction. The Diels–Alder reaction has found exceptional utility in organic synthesis ( Citation56–58) and has been utilized in the synthesis of a number of biologically active molecules ( Citation57, Citation59–Citation61). The Diels–Alder reaction provides further utility in organic synthesis due to its reversible nature. Chen et al. developed a thermally re-mendable polymer based on the reversibility of the Diels–Alder reaction between furan and maleimides ( Citation62). Weizman et al. developed an advanced undergraduate experiment to synthesize self-healing polymers based on a reversible Diels–Alder reaction ( Citation63). The synthesis of polymers with maleimide moieties was further demonstrated by Nho et al. for the preparation of polyethylene glycol-maleimide (PEG-maleimide) polymers ( Citation64). PEG is water soluble and biologically inert and non-toxic ( Citation64). These properties allow the creation of bio-compatible materials using PEG-maleimide polymers that are conjugated to specific proteins or antibodies ( Citation64). These properties could allow for the application of self-repairing polymers in the medical field.
The great utility of the Diels–Alder reaction has resulted in several experiments designed for the sophomore organic chemistry laboratory that demonstrate the utility of the Diels–Alder reaction ( Citation65–69). Additionally, Amin and Shaw have published greener procedures utilizing solar irradiation ( Citation70) and microwave heating ( Citation71) as alternative heat sources. McKenzie et al. published a room temperature, solventless method that illustrates several green chemistry principles and couples the Diels–Alder reaction with an intramolecular nucleophilic acyl substitution ( Citation72). Several multi-step synthesis experiments utilizing a Diels–Alder step have been designed for the sophomore organic chemistry course ( Citation73–77). One of these experiments utilizes a green Diels–Alder by performing a solvent-free reaction at room temperature ( Citation73). A couple of undergraduate experiments have been published utilizing maleimides as the dienophile in the Diels–Alder reaction ( Citation78, Citation79).
In addition to being an excellent dienophile, the maleimide moiety ((a)) is a core structural feature in several novel pharmaceuticals and crop safeners among other applications ( Citation80–82). Trujillo-Ferrara et al. have synthesized aryl-substituted maleimides ((b)) for application as new acetylcholinesterase inhibitors which are currently being investigated for the treatment of Alzheimer’s disease ( Citation80). Trujillo-Ferrara et al. produced five different aryl-substituted maleimides with solventless methods and obtained high yields ranging from 78% to 99%. Maleimides find further application in the agricultural industry as herbicides and fungicides ( Citation82).
Scheme 1. (a) Maleimide moity. (b) Maleimides synthesized by Trujillo-Ferrara et al. ( Citation80) as potential acetylcholinesterase inhibitors.
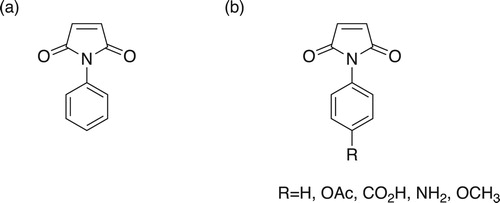
Cooley and Williams designed a multi-step synthesis utilizing a maleimide in a Diels–Alder reaction as an inquiry-based NMR experiment for the undergraduate organic chemistry laboratory ( Citation79). The synthesis utilizes an amine acylation of aniline to produce maleanilic acid developed by Cava et al. ( Citation83). The maleanilic acid is then converted to N-phenylmaleimide, and the N-phenylmaleimide is used as the dienophile in a Diels–Alder reaction with furan. The Diels–Alder reaction produces significant amounts of the endo and exo product and is used to demonstrate the NMR differences of the stereoisomers. The maleanilic acid synthesis and Diels–Alder reaction use diethyl ether and the maleimide synthesis uses cyclohexane. Diethyl ether and cyclohexane are generally accepted as non-green solvents ( Citation84).
This paper presents a similar synthetic pathway to the one presented by Cooley and Williams but utilizes greener conditions for each step of the synthesis (). Our main objectives are to enable students to: (a) identify and understand various Green Chemistry principles associated with a green reaction such as atom economy, use of safer chemicals, waste reduction, design for energy efficiency, and inherently safer chemistry for accident prevention; and (b) enable students to use 1H NMR spectroscopy data to identify the synthesized Diels–Alder product.
Experimental
Product characterization
All products were analyzed by a Perkin Elmer dual-beam FTIR spectrometer at a resolution of 4 cm−1. The products were also characterized by 1H and 13C NMR using a Bruker 400-MHz NMR spectrometer. Deuterated dimethyl sulfoxide (d6-DMSO) with 0.05% (v/v) TMS was used as the solvent for all maleanilic acids and N-(3-nitrophenyl)maleimide. Deuterated chloroform (CDCl3) with 0.3% (v/v) TMS was used as the solvent for the maleimides (except N-(3-nitrophenyl)maleimide) and maleimide-dimethylfuran cycloadducts. Reactions were also analyzed by thin-layer chromatography (TLC) using silica-gel plates containing a fluorescent indicator (Sigma-Aldrich, SKU Z122785) and a 1:1 solvent system of hexane and ethyl acetate. The TLC plates were viewed using a UV lamp. The TLC analysis gives similar results with a 1:1 heptane and ethyl acetate solvent system. Products were dissolved in ACS grade acetone for spotting. Melting points were determined by a Stanford Research Systems DigiMelt MPA-160 at a 1.0°C/min ramp rate.
Solvent-free synthesis of N-(4-chloro)maleanilic acid (3)
To facilitate mixing, 10 mmol (0.98 g) of maleic anhydride (1) was melted in a small porcelain mortar on a hotplate set to medium-low heat. Once melted, the mortar was removed from the heat and 10 mmol (1.28 g) of 4-chloroaniline (2) was added. This mixture was ground for 10 min after which the product was recovered and added to a 50 mL beaker containing 10 mL of ethyl acetate. The mixture was stirred with a stirring rod for 1 min and the product was recovered by vacuum filtration, washed with 20 mL ethyl acetate, and air-dried by spreading on a watch glass.
Alternative synthesis #1 of N-(4-chloro)maleanilic acid (3) used at University of Pittsburgh at Johnstown
In separate beakers, 6 mmol (0.588 g) of maleic anhydride (1) and 6 mmol (0.765 g) of 4-chloroaniline (2) were dissolved in ∼8 mL of ethyl acetate. The maleic anhydride solution was added to the 4-chloroaniline solution while it was stirred vigorously with a magnetic stir bar for about 5 min while an additional 5 mL of ethyl acetate was added. The product was recovered by vacuum filtration, washed with an additional 3 mL of ethyl acetate, and air-dried by spreading on a watch glass.
Alternative synthesis #2 of N-(4-chloro)maleanilic acid (3) used at Widener University
In separate beakers, 6 mmol (0.588 g) of maleic anhydride (1) and 6 mmol (0.765 g) of 4-chloroaniline (2) were dissolved in 5 mL ethyl acetate. The maleic anhydride solution was added to the 4-chloroaniline solution while it was stirred vigorously with a magnetic stir bar for about 5 min. The product was recovered by vacuum filtration, washed with an additional 3 mL of ethyl acetate, and air-dried by spreading on a watch glass.
Synthesis of N-(4-chlorophenyl)maleimide (4) with thermal heating
In a 25 mL round-bottom flask, a slurry was created by combining 5.85 mmol (1.32 g) of N-(4-chloro)maleanilic acid (3), 1.83 mmol (0.15 g) of anhydrous sodium acetate, and 31.7 mmol (3 mL) of acetic anhydride. The reaction was held between 60–70°C for 60 min. The reaction mixture was poured into a 100 mL beaker that contained 50 mL of ice-cold DI-water and stirred vigorously with a magnetic stir bar for a few minutes. The product was collected by vacuum filtration and recrystallized in ethanol. The product was air-dried until the following lab period (1 week).
Synthesis of N-(4-chlorophenyl)maleimide (4) with microwave heating
In a 10 mL borosilicate glass reaction vial with a PTFE-coated silicone septum, a slurry was created by combining 5.85 mmol (1.32 g) of N-(4-chloro)maleanilic acid (3), 3.65 mmol (0.3 g) of anhydrous sodium acetate, and 31.7 mmol (3 mL) of acetic anhydride. The reaction was heated using an Anton Paar Monowave 400 microwave at 90°C for 30 s. The flask was heated as fast as possible to reach 90°C and continuously stirred at 1200 rpm for the duration of the experiment. After cooling to 55°C, the reaction mixture was poured into a 100 mL beaker that contained 50 mL of DI-water chilled to 0°C and stirred vigorously with a magnetic stir bar for a few minutes. The product was recovered by vacuum filtration and washed with cold DI-water (2 × 10 mL) and recrystallized in ethanol. The product was air-dried for a minimum of 24 h.
Diels–Alder reaction with thermal heating
In a 3 mL conical vial, 0.44 mmol (100 mg) of N-(4-chlorophenyl)maleimide (4) was combined with 9.3 mmol (1.0 mL) of 2,5-dimethylfuran (5). After attaching a water condenser, an aluminum block was used to hold the reaction at reflux (approximately 92–94°C) for 60 min. The reaction was cooled to room temperature before the vial was placed in an ice-bath. The product was recovered by vacuum filtration and recrystallized in ethanol. The product was air-dried until the following laboratory (1 week).
Diels–Alder reaction with microwave heating
In a 10 mL borosilicate glass reaction vial with a PTFE-coated silicone septum, 0.44 mmol (100 mg) of N-(4-chlorophenyl)maleimide (4) was combined with 4.63 mmol (0.5 mL) of 2,5-dimethylfuran (5). The reaction was heated using an Anton Paar Monowave 400 microwave at 140°C for 5 min. The flask was heated as fast as possible to reach 140°C and continuously stirred at 1200 rpm for the duration of the experiment. The reaction was cooled to room temperature before the vial was placed in an ice-bath. The product was recovered by vacuum filtration and recrystallized in ethanol. The product was air-dried for a minimum of 24 h.
Results and discussion
The three-step sequential synthesis presented in provides a cost-effective, green synthesis of N-phenylmaleimides for subsequent use in a Diels–Alder reaction with 2,5-dimethylfuran. The synthesis is easily accomplished in two or three 3-h sophomore organic chemistry laboratory sessions. The synthesis is also amenable to analysis by TLC, melting point, IR spectroscopy, and/or 1H NMR spectroscopy and is an excellent capstone experiment for the second semester of the sophomore organic chemistry laboratory curriculum. The experiment has been performed in two different semesters at the University of Pittsburgh at Johnston by about 30 students each semester.
Amine acylation
The first step of the synthesis is acylation of 4-chloroaniline (2) with maleic anhydride (1) at room temperature to produce N-(4-chloro)maleanilic acid (3). An amine acylation reaction is extremely atom efficient as all the atoms from the reagents are incorporated into the maleanilic acid. The synthesis was successfully accomplished under solvent-free conditions. Similar solvent-free procedures have been reported by Trujillo-Ferrara et al. ( Citation80) and Saedi ( Citation85) but have not been adapted for the undergraduate teaching lab. While Trujillo-Ferrara et al. report 96-99% yields for several N-phenylmaleimic acids, the reaction requires 2 h of reaction time and uses 90 mL of water to wash the product (a significant increase in E-factor). The Saidi method reports a 92% yield of N-(4-chloro)maleanilic acid (3) with a 30 min reaction time followed by recrystallization with ethanol. The average student yields after only 10 min of reaction were 66%. To complete the amine acylation and cyclization steps in one laboratory period, we opted for a reaction time of 10 min. The method described here opts for washing with ∼30 mL of ethyl acetate rather than a recrystallization with ethanol to avoid the energy costs of a recrystallization and the heating of a flammable solvent. The two methods produce similar E-factors of 20–30.Footnote1
The acylation of 4-chloroaniline (2) was also accomplished using two alternative methods with ethyl acetate as a reaction solvent. The use of a solvent reduced the reaction time to ∼5 min. Alternative synthesis #1 reduced the total amount of ethyl acetate used by ∼6 mL, and produced student yields of 62%. While the student yields were slightly lower for this method, the research student yields were slightly higher for this method. Alternate synthesis #2 reduced the amount of ethyl acetate by an additional 11 mL significantly reducing the E-factor of the reaction from ∼30 to 6. Alternate synthesis #2 also resulted in a significantly higher percent yield of 87% for N-(4-chloro)maleanilic acid (3). The modified method was used by 37 students at Widener University this past semester. The 1H NMR for every student indicated a very pure product using this method.
The reaction can be analyzed thoroughly using any combination of TLC, melting point, IR spectroscopy, and 1H NMR spectroscopy. The IR and 1H NMR of the maleanilic acid are easily interpreted by the students. The 1H NMR shows six distinct peaks () and the IR clearly indicates carboxylic acid and amide groups. The reaction can be followed or analyzed after completion by TLC ((a)). The melting point of the maleamic acid (∼190°C) is significantly higher than 4-chloroaniline (72.5°C) and maleic anhydride (52.5°C) ().
Figure 1. (a) TLC of p-chloroaniline (2a, left) and N-(4-chloro)maleanilic acid (3a, right). (b) TLC of N-(4-chloro)maleanilic acid (3a, left) and N-(4-chlorophenyl)maleimide (4a, right). (c) TLC of N-(4-chlorophenyl)maleimide (4a, left) and Diels–Alder product (6a, right). TLCs were performed using silica-gel plates containing a fluorescent indicator and a 1:1 solvent system of hexane and ethyl acetate.
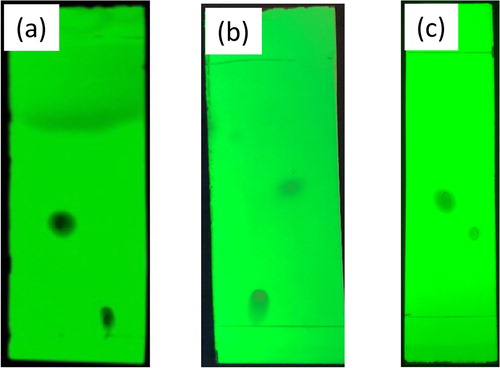
Table 1. Summary of 1H NMR data for each step of the sequential synthesis.
Table 2. Reaction conditions, percent yield, E-factors, and melting points for each step of the sequential synthesis.
Synthesis of maleimide
The second step of the synthesis uses a modification of a classic method ( Citation83, Citation86) to cyclize N-(4-chloro)maleanilic acid (3) to N-(4-chlorophenyl)maleimide (4) utilizing excess acetic anhydride and catalytic sodium acetate at 60–70°C. The reaction was accomplished using a reaction temperature of about 15°C lower than the reported methods with similar yields (70%) in our research labs; however, the average student yields were 55%. The lower student yields are likely due to overheating of the reaction as care must be taken to maintain a reaction temperature below 70°C and preferably around 65°C. The literature method was also modified to replace cyclohexane with ethanol as the recrystallization solvent. The reaction can be analyzed thoroughly using any combination of TLC, melting point, IR spectroscopy, and 1H NMR spectroscopy. The reaction can be followed or analyzed after completion by TLC ((b)). The melting points of the maleamic acid (∼190°C) and maleimide (∼115°C) are significantly different (). The IR spectra clearly indicate the loss of a hydroxyl group, and the 1H NMR shows three distinct peaks and demonstrates the symmetry created by the ring cyclization ().
The two-step sequence presented here provides a cost-effective synthesis of N-(4-chlorophenyl)maleimide (4). The cost of the raw materials to produce one gram of N-(4-chlorophenyl)maleimide (4) is $2.75. The commercial cost of N-(4-chlorophenyl)maleimide (4) from Millipore-Sigma is $133 per gram.
The reaction is not atom efficient with only 51% of the reactant atoms being incorporated into the maleimide. Trujillio-Ferrara et al. ( Citation80) report a slightly more atom efficient conversion of N-(4-chloro)maleanilic acid (3) to N-(4-chlorophenyl)maleimide (4) in the absence of acetic anhydride but the reaction requires heating at 80–85°C for 4 h. To implement this method in the undergraduate laboratory, the reaction time would need to be cut to 1–1.5 h and would result in much lower yields.
Kumar et al. report two solvent-free methods for converting maleanilic acids to maleimides ( Citation87). The first method reacts the maleanilic acid with dicyclohexylcarbodiimide by grinding at room temperature for 8 min. The atom economy is moderate at 48% and the resulting maleimide is produced in a 67% yield. The reaction requires the same workup with ice-cold water as our method but requires an additional wash with chloroform to remove the dicyclohexylurea by-product. This method is intriguing if the hazardous chloroform wash could be replaced with a greener solvent. Therefore, we attempted this method using an ethyl acetate wash but this resulted in low yields of the maleimide. The second method reported by Kumar et al. involves the grinding at room temperature of the maleanilic acid with an alkylating agent, potassium carbonate, and tetrabutylammonium bromide for 8 min. The resulting maleimide is produced in a 71% yield. This method requires about half the water wash as the method reported here. However, the atom economy is only 27% and the method requires the use of either a highly flammable or significantly hazardous alkylating agent.
Additionally, there are two reports of the synthesis of N-(4-chlorophenyl)maleimide directly from maleic anhydride and 4-chloroaniline that are extremely atom efficient (atom economy for both is 92%). Reddy et al. report that the grinding method utilized here results in the maleimide product ( Citation88), however, we have been unable to reproduce this result. Le et al. use an ionic liquid to directly synthesize maleimides from an anhydride and aniline ( Citation89). While Le’s method is atom efficient, the reaction requires a significantly higher reaction temperature of 140°C and synthesis of the ionic liquid. The synthesis of the ionic liquid requires a wash with THF which negates the low E-factor of the ionic liquid mediated cyclization reaction.
Diels–Alder reaction
The Diels–Alder reaction is a highly efficient and green reaction that can be utilized to control the configuration of multiple stereocenters and can be enantioselective. The third step of the synthesis utilizes a solvent-free Diels–Alder reaction between N-(4-chlorophenyl)maleimide (4) and 2,5-dimethylfuran (5) via a 10.5 mmol excess of the furan. After 1 h of refluxing, the reaction produces a 46% yield of exo-2-(4-chlorophenyl)-3a,4,7,7a-tetrahydro-4,7-dimethyl-4,7-epoxy-1H-isoindole-1,3(2H)-dione (6). The yield could be improved with additional heating time. The product precipitates as a white powder upon cooling. Barr and Wustholz report a Diels–Alder reaction between N-phenylmaleimide and 1,3-cyclohexadiene in ethyl acetate ( Citation90). While they achieved higher student yields (78%), the E-factor of their reaction is significantly higher because of the ethyl acetate solvent. We found the 46% yield acceptable and plenty of product for TLC, melting point, IR, and 1H NMR analysis. Cooley ( Citation79) and Barr ( Citation90) also report significant yields (∼90%) for a room temperature Diels–Alder reaction involving N-phenylmaleimide after 7 days.
The analysis of this reaction nicely demonstrates the power of 1H NMR and the limitations of melting point and IR spectroscopy. There is a slight difference in the Rf values ((c)) of N-(4-chlorophenyl)maleimide (4) and the Diels–Alder adduct (6). Additionally, the melting points of the N-(4-chlorophenyl)maleimide (4) (∼115°C) and 6 (∼124°C) are similar (). The IR spectra of 6 do not readily identify the addition of functional groups. Thus, the students must rely on 1H NMR to identify the product. The 1H NMR shows five distinct, clearly identifiable peaks for the diastereotopic hydrogens while beautifully demonstrating the symmetry of a structurally complex molecule (). While we did not ask the students to identify whether the endo or exo product was produced. We found that the exo product was typically favored 95:5 under the presented reaction conditions. Identification of the exo:endo ratio using 1H NMR spectra in a Diels–Alder reaction involving a maleimide-furan cycloadduct was described by Cooley and Williams ( Citation79). The peak corresponding to the C5 and C6 protons appears at ∼Δ3.0 ppm for the exo product and at ∼Δ3.4 ppm for the endo product. Given this information, students could be asked to calculate the exo:endo ratio using the integrations.
Implementation with other anilines and dienes
The synthesis also works nicely using p-methoxyaniline (2b), p-methylaniline (2c), and m-nitroaniline (2d) in place of p-chloroaniline (2a). While no modifications to the conditions of alternative synthesis #1 were necessary using p-methoxyaniline or p-methylaniline, the amine acylation with m-nitroaniline required 45 min of stirring using the alternative ethyl acetate method. However, the maleimide formation (4b-d) and Diels–Alder reaction (6b-d) did not require any modification. While the reactions with these three alternative anilines have not been implemented in the sophomore organic chemistry lab, they have been successfully completed multiple times by undergraduate research students with nice yields () and easily interpreted 1H NMR spectra () for the maleimic acid (3b-d) and maleimide (4b-d) products. The yields for the Diels–Alder reaction using the N-(4-methoxyphenyl)maleimide and N-(3-nitrophenyl)maleimide were significantly lower but the 1H NMR spectra are easily obtained and interpreted ().
The Diels–Alder reaction was also performed using 2,3-dimethylbutadiene and furan with N-(4-chlorophenyl)maleimide (4). The reaction with 2,3-dimethylbutadiene worked nicely but the 1H NMR interpretation is significantly more complicated for undergraduates and 2,3-dimethylbutadiene is significantly more expensive than 2,5-dimethylfuran. The reaction also works nicely with furan as demonstrated by Cooley and Williams ( Citation79) but the NMR is more difficult to interpret and the low boiling point of furan introduces an additional hazard.
Implementation of a microwave reactor
A microwave reactor can be utilized in the second and third steps of the synthesis. We explored optimal temperature and reaction times in an Anton Parr Monowave 400 for maleimide formation and the Diels–Alder reaction.
Synthesis of maleimide
For the cyclization of N-(4-chloro)maleanilic acid (3) to N-(4-chlorophenyl)maleimide (4), we found the optimal microwave reaction conditions to be 90°C for 30 s. The reaction showed a slight increase in yield from 70% to 73% but the reaction time is reduced by 99% with a moderate increase in the reaction temperature (50%).
Diels–Alder reaction
For the Diels–Alder reaction between N-(4-chlorophenyl)maleimide (4) and 2,5-dimethylfuran (5), we found the optimal microwave reaction conditions to be 140°C for 5 min. The reaction showed a similar percent yield of 44% but the reaction time is reduced by 92% with a moderate increase in the reaction temperature (50%). Additionally, the excess amount of 2,5-dimethylfuran was reduced from 1 to 0.5 mL. This decrease reduces the E-factor for the microwave version from 15 to 8.
Conclusions
A three-step sequential synthesis for the undergraduate organic chemistry laboratory has been developed that produces a commercially and biologically important N-phenylmaleimide in two-steps. The product is then utilized as the dienophile in a Diels–Alder reaction with 2,5-dimethylfuran to produce a cycloadduct that also has potential biological applications. Students were excited by the drastic color change in the amine acylation step. Students were intrigued to synthesize an expensive commercial product (N-(4-chlorophenyl)maleimide) for a fraction of its commercial price. Since synthesis is a major emphasis in the Organic II curriculum, students enjoyed performing a sequential synthesis in the laboratory.
Supplemental Material
Download MS Word (1.1 MB)Acknowledgements
The authors would like to thank Mark Nathaniel for initial work performed on the project. The authors would also like to thank Brian Feronti for his contributions to the p-methyl derivatives. Loyd Bastin and Brendan Gainer thank Marty Schultz for NMR support and maintenance, and Krishna Bhat, Irina Knyazeva, Robert Mishur, and Michael Tylinski for their assistance implementing the experiment.
Disclosure statement
No potential conflict of interest was reported by the authors.
Notes on contributors
Loyd D. Bastin is a professor of chemistry and biochemistry at Widener University where he teaches Organic Chemistry, Biochemistry, and Sustainability and currently serves as Co-Chair of the Widener University Sustainability Council and a member of the Chester Environmental Partnership. Since 2001, Dr Bastin has developed best practices for incorporating green chemistry, sustainability, and environmental justice into the curriculum. His current areas of interest are the development of greener methods for synthesizing pharmaceuticals and the development of new laboratory experiments for the undergraduate chemistry curriculum.
Manisha Nigam is an associate professor of chemistry at the University of Pittsburgh at Johnstown where she teaches Organic Chemistry and Green Chemistry and Sustainability. Dr Nigam’s scholarship and professional development activities are primarily focused on Green Chemistry Education, where her research goal is to develop environmentally friendly experiments for the undergraduate laboratories to achieve chemical transformations without the use of hazardous chemicals.
Sam Martinus is an instructor in the Department of Chemistry at the University of Pittsburgh at Johnstown.
James E. Maloney recently graduated with a B.S. in biochemistry from the University of Pittsburgh at Johnstown and is currently an Associate Scientist at Eurofins Lancaster Laboratories.
Landon L. Benyack recently graduated with a B.S. in biochemistry from the University of Pittsburgh at Johnstown and is currently a first-year medical student at Drexel University.
Brendan Gainer recently graduated with a B.S. in chemistry from Widener University and is currently a Process Chemist at Johnson Matthey.
Additional information
Funding
Notes
1 The waste generated for each calculation was estimated by summing the amount of solvent required by each method (reaction and purification).
References
- Taber, D.F. In Organic Chemistry Highlights organic-chemistry.org, 2004 -present. www.organic-chemistry.org/Highlights (accessed April 23, 2019).
- Nicolaou, K.C.; Sorensen, E.J. Classics in Total Synthesis: Targets, Strategies, Methods; Wiley-VCH: New York, NY, 1996.
- Nicolaou, K.C.; Snyder, S.A. Classics in Total Synthesis II: More Targets, Strategies, Methods; Wiley-VCH: Chichester, 2003.
- Nicolaou, K.C.; Chen, J.S. Classics in Total Synthesis III: Further Targets, Strategies, Methods; Wiley-VCH: Hoboken, NJ, 2011.
- Mayo, D.W.; Pike, R.M.; Trumper, P.K. Microscale Organic Laboratory: With Multistep and Multiscale Syntheses, 4th ed.; Wiley: New York, NY, 2000.
- Marchetti, L.; DeBoef, B. J. Chem. Educ. 2015, 92, 1536–1538. doi: 10.1021/ed500961h
- Anderluh, M. Chem. Educ. 2013, 18, 155–158.
- Touaibia, M.; Guay, M. J. Chem. Educ. 2011, 88, 473–475. doi: 10.1021/ed100050z
- Kjonaas, R.A.; Williams, P.E.; Counce, D.A.; Crawley, L.R. J. Chem. Educ. 2011, 88, 825–828. doi: 10.1021/ed100892p
- Utku, Y.; Rohatgi, A.; Yoo, B.; Kirshenbaum, K.; Zuckermann, R.N.; Pohl, N.L. J. Chem. Educ. 2010, 87, 637–639. doi: 10.1021/ed100202f
- Baptistella, L.H.B.; Imamura, P.M.; Vilela de Melo, L.; Castello, C. Quim. Nova 2009, 32, 1069–1071. doi: 10.1590/S0100-40422009000400042
- Feist, P.L. J. Chem. Educ. 2008, 85, 1548–1549. doi: 10.1021/ed085p1548
- Taber, D.F.; Patel, S.; Hambleton, T.M.; Winkel, E.E. J. Chem. Educ. 2007, 84, 1158. doi: 10.1021/ed084p1158
- Maheut, G.; Liao, L.; Catel, J.-M.; Jaffrès, P.-A.; Villemin, D. J. Chem. Educ. 2001, 78, 654–657. doi: 10.1021/ed078p654
- Callam, C.S.; Lowary, T.L. J. Chem. Educ. 2001, 78, 73–74. doi: 10.1021/ed078p73
- deLannoy, P.; Mann, D.; Maycock, J.; Davidson, C. Chem. Educ. 1999, 4, 65–67. doi: 10.1007/s00897990285a
- Young, P.E.; Campbell, A. J. Chem. Educ. 1982, 59, 701–702. doi: 10.1021/ed059p701
- McCullagh, J.V.; Hirakis, S.P. J. Chem. Educ. 2017, 94, 1347–1351. doi: 10.1021/acs.jchemed.6b00778
- Cristiano, R.; Cabral, M.G.B.; de Aquino, R.B.; Cristiano, C.M.Z. Quim. Nova 2014, 37, 181–185. doi: 10.1590/S0100-40422014000100031
- Marks, P.; Levine, M. J. Chem. Educ. 2012, 89, 1186–1189. doi: 10.1021/ed300187d
- Habeck, J.C.; Diop, L.; Dickman, M. J. Chem. Educ. 2010, 87, 528–529. doi: 10.1021/ed800169h
- Miles, W.H.; Connell, K.B. J. Chem. Educ. 2006, 83, 285–286. doi: 10.1021/ed083p285
- Breton, G.W.; Belk, M.K. Chem. Educ. 2004, 9, 27–29.
- Baptistella, L.H.B.; Giacomini, R.A.; Imamura, P.M. Quim. Nova 2003, 26, 284–286. doi: 10.1590/S0100-40422003000200024
- Keller, V.A.; Kendall, B.L. J. Chem. Educ. 2017, 94, 1450–1457. doi: 10.1021/acs.jchemed.7b00093
- Solano, L.M.R.; Lourenço, N.M.T. Quim. Nova 2015, 38, 285–287.
- Feng, Z.V.; Edelman, K.R.; Swanson, B.P. J. Chem. Educ. 2015, 92, 723–727. doi: 10.1021/ed5005307
- Hagen, J.P. J. Chem. Educ. 2011, 88, 1603–1604. doi: 10.1021/ed200010d
- Alber, J.P.; DeGrand, M.J.; Cermak, D.M. J. Chem. Educ. 2011, 88, 82–85. doi: 10.1021/ed1004728
- Brown, D.P.; Durutlic, H.; Juste, D. J. Chem. Educ. 2004, 81, 1016–1017. doi: 10.1021/ed081p1016
- Cunha, S.; Liao, L.M.; Bonfim, R.R.; Bastos, R.M.; Monteiro, A.P.M.; Alencar, K.S. Quim. Nova 2003, 26, 425–427. doi: 10.1590/S0100-40422003000300022
- Hanson, J. J. Chem. Educ. 2001, 78, 1266–1268. doi: 10.1021/ed078p1266
- Labadie, G.R.; Cravero, R.M. Chem. Educ. 1999, 4, 219–220. doi: 10.1007/s00897990337a
- Todd, D. J. Chem. Educ. 1991, 68, 682. doi: 10.1021/ed068p682
- Green, I.R. J. Chem. Educ. 1982, 59, 698–699. doi: 10.1021/ed059p698
- Hawbecker, B.L.; Radovich, D.A.; Tillotson, L.G. J. Chem. Educ. 1976, 53, 398–399. doi: 10.1021/ed053p398
- Paulson, D.R.; Hartwig, A.L.; Moran, G.F. J. Chem. Educ. 1973, 50, 216–217. doi: 10.1021/ed050p216
- Pontrello, J.K. Biochem. Mol. Biol. Educ. 2015, 43, 417–427. doi: 10.1002/bmb.20915
- Fuller, A.A. J. Chem. Educ. 2016, 93, 953–957. doi: 10.1021/acs.jchemed.5b00671
- Pontrello, J.K. J. Chem. Educ. 2015, 92, 811–818. doi: 10.1021/ed500193h
- Slade, M.C.; Raker, J.R.; Kobilka, B.; Pohl, N.L.B. J. Chem. Educ. 2014, 91, 126–130. doi: 10.1021/ed300375v
- Graham, K.J.; Schaller, C.P.; Johnson, B.J.; Klassen, J.B. Chem. Educ. 2002, 7, 376–378. doi: 10.1007/s00897020612a
- Christiansen, M.A.; Crawford, C.L.; Mangum, C.D. Chem. Educ. 2014, 19, 28–33.
- Anastas, P.T.; Warner, J.C. Green Chemistry: Theory and Practice; Oxford University Press: New York, 1998.
- Dicks, A.P., Green Organic Chemistry in Lecture and Laboratory; CRC Press: New York, 2012.
- Morra, B.; Dicks, A.P. In Green Chemistry Experiments in Undergraduate Laboratories; Fahey, J.T., Maelia, L.E., Eds.; Vol. ACS Symposium Series 1233; American Chemical Society: 2016; pp 7–32.
- Johnston, M.R.; Makriyannis, A.; Whitten, K.M.; Drew, O.C.; Best, F.A. J. Chem. Educ. 2016, 93, 2080–2083. doi: 10.1021/acs.jchemed.6b00225
- Bastin, L.D.; Gerhart, K. In Green Chemistry Experiments in Undergraduate Laboratories; Fahey, J.T., Maelia, L.E., Eds.; American Chemical Society: 2016; Vol. 1233, p 55-69.
- Williamson, C.L.; Maly, K.E.; MacNeil, S.L. J. Chem. Educ. 2013, 90, 799–801. doi: 10.1021/ed300369m
- Dintzner, M.R.; Kinzie, C.R.; Pulkrabek, K.; Arena, A.F. J. Chem. Educ. 2012, 89, 262–264. doi: 10.1021/ed2000878
- Cardinal, P.; Greer, B.; Luong, H.; Tyagunova, Y. J. Chem. Educ. 2012, 89, 1061–1063. doi: 10.1021/ed200579w
- Bisol, T.B.; Marques, M.V.; Rossa, T.A.; Nascimento, M.d.G.; Sá, M.M. Quim. Nova 2012, 35, 1260–1263. doi: 10.1590/S0100-40422012000600035
- Dzyuba, S.V.; Kollar, K.D.; Sabnis, S.S. J. Chem. Educ. 2009, 86, 856–858. doi: 10.1021/ed086p856
- Cave, G.W.V.; Raston, C.L. J. Chem. Educ. 2005, 82, 468–469. doi: 10.1021/ed082p468
- Fringuelli, F.; Piermatti, O.; Pizzo, F. J. Chem. Educ. 2004, 81, 874–876. doi: 10.1021/ed081p874
- Evans, D.A.; Johnson, J.S. In Comprehensive Asymmetric Catalysis I-III; Jacobsen, E.N., Pfaltz, A., Yamamoto, H., Eds.; Springer-Verlag: Berlin , 1999; pp 1177–1235.
- Nicolaou, K.C.; Snyder, S.A.; Montagnon, T.; Vassilikogiannakis, G. Angew. Chem., Int. Ed. 2002, 41, 1668–1698. doi: 10.1002/1521-3773(20020517)41:10<1668::AID-ANIE1668>3.0.CO;2-Z
- Jiang, X.; Wang, R. Chem. Rev. 2013, 113, 5515–5546. doi: 10.1021/cr300436a
- Suryanarayana Birudukota, N.V.; Franke, R.; Hofer, B. Org. Biomol. Chem. 2016, 14, 3821–3837. doi: 10.1039/C5OB02539G
- Daly, J.W.; Spande, T.F. In Alkaloids: Chemical and Biological Perspectives; Pelletier, S.W., Ed.; Vol. 4; Wiley: New York, 1986; pp 1–254.
- Needleman, S.B.; Kuo, M.C.C. Chem. Rev. 1962, 62, 405–431. doi: 10.1021/cr60219a002
- Chen, X.; Dam, M.A.; Ono, K.; Mal, A.; Shen, H.; Nutt, S.R.; Sheran, K.; Wudl, F. Science 2002, 295, 1698–1702. doi: 10.1126/science.1065879
- Weizman, H.; Nielsen, C.; Weizman, O.S.; Nemat-Nasser, S. J. Chem. Educ. 2011, 88, 1137–1140. doi: 10.1021/ed101109f
- Nho, K.; Hyun, C.; Lee, J.; Pak, Y. United States Patent 6,828,401 B2, 2004.
- Keller, J.W. Chem. Educ. 2006, 11, 262–266.
- Persson, A.; Lindstroem, U.M. J. Chem. Educ. 2004, 81, 1353–1354. doi: 10.1021/ed081p1353
- Palmer, D.R.J. J. Chem. Educ. 2004, 81, 1633–1635. doi: 10.1021/ed081p1633
- Sauliova, J. Chem. Listy 2002, 96, 761–765.
- McDaniel, K.F.; Weekly, R.M. J. Chem. Educ. 1997, 74, 1465–1467. doi: 10.1021/ed074p1465
- Amin, S.; Barnes, A.; Buckner, C.; Jones, J.; Monroe, M.; Nurmomade, L.; Pinto, T.; Starkey, S.; Agee, B.M.; Crouse, D.J.; Swartling, D.J. J. Chem. Educ. 2015, 92, 767–770. doi: 10.1021/ed500850c
- Shaw, R.; Severin, A.; Balfour, M.; Nettles, C. J. Chem. Educ. 2005, 82, 625–629. doi: 10.1021/ed082p625
- McKenzie, L.C.; Huffman, L.M.; Hutchison, J.E.; Rogers, C.E.; Goodwin, T.E.; Spessard, G.O. J. Chem. Educ. 2009, 86, 488–493. doi: 10.1021/ed086p488
- Patterson, A.L.; May, M.D.; Visser, B.J.; Kislukhin, A.A.; Vosburg, D.A. J. Chem. Educ. 2013, 90, 1685–1687. doi: 10.1021/ed400445j
- Callahan, R.; Marshall, K.; Rothchild, R.; Rosmarion, K. Chem. Educ. 2001, 6, 227–234. doi: 10.1007/s00897010488a
- Mayo, D.W.; Pike, R.M.; Trumper, P.K. In Microscale Organic Laboratory: With Multistep and Multiscale Syntheses, 4th ed.; John Wiley & Sons, Inc.: New York, 2000; pp 419–452.
- Harrison, E.A., Jr. J. Chem. Educ. 1991, 68, 426–427. doi: 10.1021/ed068p426
- McClure, C.K.; Chenault, H.K. J. Chem. Educ. 1996, 73, 467–470. doi: 10.1021/ed073p467
- Baar, M.R.; Gammerdinger, W.; Leap, J.; Morales, E.; Shikora, J.; Weber, M.H. J. Chem. Educ. 2014, 91, 1720–1724. doi: 10.1021/ed4005485
- Cooley, J.H.; Williams, R.V. J. Chem. Educ. 1997, 74, 582–585. doi: 10.1021/ed074p582
- Trujillo-Ferrara, J.; Correa-Basurto, J.; Espinosa, J.; García, J.; Martínez, F.; Miranda, R. Synth. Commun. 2005, 35, 2017–2023. doi: 10.1081/SCC-200066665
- Matuszak, N.; Muccioli, G.G.; Labar, G.; Lambert, D.M. J. Med. Chem. 2009, 52, 7410–7420. doi: 10.1021/jm900461w
- Rubin, B.; Kirino, O. In Crop Safeners for Herbicides: Development, Uses, and Mechanisms of Action; Hatzios, K.K., Hoagland, R.E., Eds.; Academic Press, Inc.: San Diego, CA, 1989; pp 317–336.
- Cava, M.P.; Deana, A.A.; Muth, K.; Mitchell, M.J. In Organic Syntheses, Collective Volume 5 1973; pp 944–945.
- Henderson, R.K.; Jiménez-González, C.; Constable, D.J.C.; Alston, S.R.; Inglis, G.G.A.; Fisher, G.; Sherwood, J.; Binks, S.P.; Curzons, A.D. Green Chem. 2011, 13, 854–862. doi: 10.1039/c0gc00918k
- Saedi, H. Bull. Chem. Soc. Ethiop. 2013, 27, 137–141.
- Searle, N.E. United States Patent 2,444,536, 1948.
- Kumar, P.P.; Devi, B.R.; Dubey, P.K. Indian J. Chem., Sec. B 2013, 52B, 1166–1171.
- Reddy, Y.D.; Reddy, C.V.R.; Dubey, P.K. Afro Asian J. Sci. Tech. 2014, 1, 5–9.
- Le, Z.-G.; Chen, Z.-C.; Hu, Y.; Zheng, Q.-G. Synthesis. 2004, 2004, 995–998.
- Baar, M.R.; Wustholz, K. J. Chem. Educ. 2005, 82, 1393–1394. doi: 10.1021/ed082p1393