ABSTRACT
A two-step synthetic sequence was developed for the undergraduate organic chemistry laboratory using vanillin as the starting material. The multi-step synthesis was designed to replace two traditional experiments teaching electrophilic aromatic substitution and carbon–carbon bond forming chemistries with greener transformations. Vanillin is iodinated using Oxone® and potassium iodide in refluxing water, and students are tasked with determining the position of aromatic substitution using 1H NMR spectroscopy. The tan, shiny, pleasant-smelling iodovanillin is subsequently used in an aqueous Suzuki-Miyaura reaction with para-methylphenylboronic acid; strategically chosen to afford a second instructive 1H NMR spectrum. Both conventional heating and microwave conditions can be employed for the palladium-catalyzed reaction. This synthetic sequence, successfully performed over multiple semesters by hundreds of students, models green chemistry principles through the use of a potentially renewable feedstock and safer reagents, the choice of water as a safer reaction solvent, and the employment of a catalytic reaction. Additionally, the sequence minimizes waste in teaching labs through use of an intermediate product.
GRAPHICAL ABSTRACT
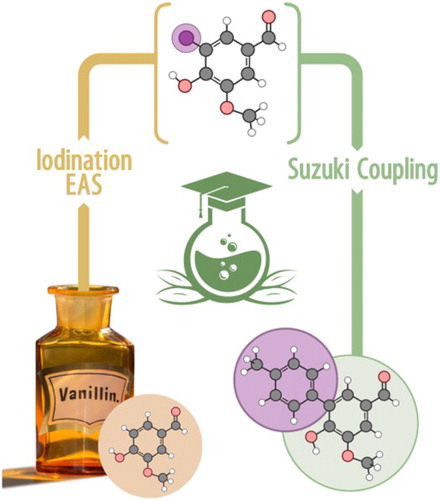
Introduction
Continuing with our efforts to revise the organic chemistry laboratory curriculum to demonstrate modern green synthetic methods, we sought to replace our program’s decades-old electrophilic aromatic substitution (EAS) reaction and Grignard synthesis with more environmentally-friendly chemistries. These classes of reactions are important topics to include in both organic chemistry lecture and laboratory courses. EAS reactions are invaluable synthetic tools for functionalizing aromatic compounds, and the Grignard reaction has historically been a primary carbon–carbon bond forming reaction. Though there are reported green replacements for each of these types of reactions ( Citation1), we sought to develop novel experiments which would complement our current curriculum with specific learning outcomes related to guided-inquiry pedagogy, spectroscopic analysis, laboratory techniques used, green chemistry principles, and scalability for the large teaching laboratory environment.
Greener, guided-inquiry electrophilic aromatic substitution reaction
Like students at institutions across the nation, students in our laboratories gained hands-on experience with an electrophilic aromatic substitution reaction through the nitration of methyl benzoate. This experiment had many virtues in that the white crystalline product (methyl meta-nitrobenzoate) was ideal for learning the technique of recrystallization, and its 1H nuclear magnetic resonance (NMR) spectrum featured well-resolved meta and ortho aromatic coupling for student interpretation. However, the use of the strong mineral acid mixture of nitric and sulfuric acid posed significant hazards to the students both during experimentation and in producing a strong oxidizing waste. Improper disposal by students of the nitration experiment waste into an organic waste stream presented a high risk for explosion or fire. This scenario was most likely the case in a reported explosion and injury in a teaching laboratory performing this same experiment ( Citation2).
Our aim was to design a guided-inquiry experiment exemplifying similar learning outcomes to the nitration experiment through a greener, safer transformation. The ideal substrate would have multiple possible substitution positions so that EAS selectivity could be studied and would afford a crystalline product with instructive 1H NMR spectral features. Recently, we developed an oxidation of borneol to camphor using Oxone® and catalytic sodium chloride ( Citation3). This experiment has been a highly successful green addition to our organic chemistry laboratory curriculum. Oxone® is a stable triple salt consisting of 2KHSO5•KHSO4•K2SO4 which has found wide spread application in synthetic chemistry ( Citation4). One such application is the halogenation of aromatic rings using a combination of Oxone® and a halide salt in various solvents ( Citation5–7). This reaction works most efficiently with aromatic substrates containing one or more electron-donating substituents ( Citation8). The active oxidizing agent in Oxone® is potassium peroxymonosulfate (KHSO5) which is thought to react with halide salts (M + X-) to produce a source of the electrophilic X+ in the form of a hypohalous acid, HOX ( Citation4). After workup, the by-products of the reaction are environmentally-benign potassium sulfate salts.
The first substrate investigated using the EAS Oxone® chemistry was para-methylanisole (). Para-methylanisole affords a question-driven approach for students as they compare the directing power of the methoxy substituent versus the methyl group. A student-friendly protocol, Oxone®/KBr (1 equivalent) in a two-phase solvent system of ethyl acetate and water, was developed in the research laboratory and implemented in the introductory organic chemistry laboratory course. Though the synthesis was successful in the hands of the students, yielding a product with a clean and instructive 1H NMR spectrum, the liquid 2-bromo-4-methylanisole compound had an unacceptably strong ‘urinal disinfectant’ odor that was not only unpleasant, but also lingered for days.
We then surveyed other select commercially available, cost-effective substrates, the majority of which are illustrated in , using Oxone®/bromide and iodide salts in various green solvent systems such as methanol, water, or an ethyl acetate/water co-solvent system. Acetaminophen, methyl para-anisate, para-cymene, and eugenol gave generally poor yields of products. Though not rigorously explored, one would expect that eugenol would have a competing epoxidation reaction with its double bond. Bromination of para-tolyl acetate gave a liquid product with an unpleasant odor and was therefore dismissed. 1,4-Dimethoxybenzene and para-methylacetanilide showed promise as substrates with respect to yield of product and each has appeared in independent publications involving green teaching lab experiments ( Citation9 , Citation10). As seen in , both references employed Oxone® and either sodium or ammonium bromide in an aqueous solvent system. Though para-methylacetanilide piqued our interest, the 2-bromo-4-methylacetanilide product did not have detectable coupling between the aromatic meta hydrogens using either a 400 or 500 MHz 1H NMR instrument.
To our delight, vanillin (4-hydroxy-3-methoxybenzaldehyde), a potentially renewable and affordable starting material with a pleasant odor, was observed to selectively undergo both bromination and iodination using Oxone® and the corresponding potassium salt in ethyl acetate/water. Upon further optimization of the reaction conditions, we determined that the iodination reaction was successful in a 100% aqueous system, whereas, the bromination reaction was not. Inspection of the Safety Data Sheets (SDS) of the 5-bromovanillin ( Citation11) and 5-iodovanillin ( Citation12) compounds, both commercially available from Sigma-Aldrich Millipore, revealed that 5-bromovanillin is known to have acute aquatic toxicity “with long lasting effects.” Therefore, iodination of vanillin using Oxone® and KI in refluxing water was chosen as the reaction to further pursue for the teaching laboratory ().
Not surprisingly, many other procedures are reported for the iodination of vanillin with more recent protocols using I2, KI, NaHCO3 in water ( Citation13), N-iodosuccinimide in hexafluoroisopropanol ( Citation14), and potassium triiodide (KI3), acetic acid and sodium periodate (NaIO4) ( Citation15). Of special note is a teaching laboratory experiment reported by Doxsee and Hutchison ( Citation16) wherein a sodium hypochlorite solution (NaOCl) (bleach) was used in combination with NaI in ethanol/water. These conditions most likely follow the same mechanistic pathway as the Oxone® procedure. Our prior experience with bleach as a reagent in the teaching lab reagent was that it often gave inconsistent results and was more challenging to handle as a liquid with noxious fumes. In contrast, Oxone® is an easily handled, stable salt that affords reliable results and forms benign by-products.
Suzuki-Miyaura coupling reaction of 5-iodovanillin
Upon successful implementation of the Oxone®/KI iodination procedure in our teaching laboratories over several semesters, we sought to use the 5-iodovanillin product as a substrate in a subsequent reaction. Doxsee and co-authors ( Citation16 , Citation17) demonstrated the reaction of 5-iodovanillin in a Sonogashira coupling reaction (palladium (II) acetate, a phosphine ligand, CuI and N-methylmorpholine) with 2-methyl-3-butyne-2-ol to form a benzofuran product via the ortho phenol substituent (). We were interested in exploring the Suzuki-Miyaura reaction, the cross-coupling of an aryl or vinyl halide with an aryl or vinyl boronic acid, as a modern topic increasingly taught in organic chemistry lecture and laboratory courses. The importance of this chemistry is easy to emphasize to students through recognition of Akira Suzuki as a joint recipient of the Nobel Prize in Chemistry in 2010 for contributions in “palladium-catalyzed cross couplings in organic syntheses” ( Citation18) and the widespread application in synthesis of pharmaceuticals ( Citation19). Additionally, the Suzuki cross-coupling reaction has evolved to be exceptionally amenable to green chemistry approaches including the use of catalysis, green solvents (including water), ball-milling, microwave, ultrasound, and flow reactors ( Citation20). In summary, the Suzuki-Miyaura reaction offers an efficient, green, and cost-effective route to C–C bond formation for the organic chemistry curriculum.
Scheme 3. Published Sonogashira coupling reaction using 5-iodovanillin ( Citation16, Citation17).

There have been multiple Suzuki-type reactions published for the undergraduate laboratory curriculum employing both aryl bromides ( Citation21–23) and aryl iodides ( Citation24) as coupling agents with arylboronic acids using various palladium catalysts.
The procedure that appeared most compatible with 5-iodovanillin was that of Dicks and co-authors ( Citation24), who demonstrated that phenylboronic acid can be cross-coupled with 4-iodophenol using 10% Pd/C, K2CO3, in refluxing water for 30 min. This experiment featured ligand-free use of a palladium source, water as a solvent, and facile isolation of the product. We followed identical reaction protocols and discovered that the C–C coupling of 5-iodovanillin and 4-methylphenyl boronic acid yielded the corresponding biaryl product in 30 min. The reaction was also amenable to microwave conditions as illustrated in . The 1H NMR spectrum of the crystalline, diphenyl product, 6-hydroxy-5-methoxy-4′-methyl-[1,1′-biphenyl]-3-carbaldehyde, met our expectations with somewhat challenging, but interpretable peaks spanning 2.4 ppm to 9.9 ppm and displaying both ortho and meta coupling of aromatic protons. Keesara and Parvathaneni reported synthesis of this product in their studies of an arylpiperazine ligated catalyst for the Suzuki-Miyaura reaction ( Citation25).
Experimental methods
Materials
All materials were used as received unless otherwise noted. The 4-methyphenyl boronic acid was purchased from Combi-Blocks. The 10% Pd/C was purchased from Acros. All other chemicals were purchased from Millipore Sigma-Aldrich.
Hazards
Goggles should be worn at all times. Ethyl acetate, isopropanol, and acetone are highly flammable and cause eye irritation. Vanillin is a serious eye irritant. 5-Iodovanillin is a possible skin, eye, and respiratory irritant. Oxone® can cause severe skin burns and eye damage. Potassium carbonate and 4-methylphenylboronic acid are skin and eye irritants and may cause respiratory irritation. Potassium iodide causes thyroid damage through prolonged or repeated exposure if swallowed. Chloroform-d is an inhalation hazard and suspected carcinogen. The hydrochloric acid solution should be handled with gloves (nitrile rubber) to avoid severe skin burns.
Microwave reactor
CEM Discover® SP monomode microwave equipped with an Explorer 48 auto sampler was used.
Iodination of vanillin using Oxone® and KI in water
Oxone® (1.05 g, 3.41 mmol of KHSO5) is dissolved in 4 mL of deionized (DI) water. An apparatus is assembled using a 25-mL round bottom flask and Claisen adapter equipped with both a reflux condenser and addition funnel. The clear Oxone® solution is added dropwise over 5 min to the rapidly stirred suspension of vanillin (0.50 g, 3.3 mmol) and KI (0.55 g, 3.3 mmol) in 4 mL of DI water. The resulting turbid solution is refluxed for 1 h during which time purple iodine vapors/crystals may be observable. The solution is cooled and DI water (10 mL) is used to rinse the sides of the condenser into the flask to consolidate the material with continued stirring. The mixture is tested for excess oxidizing agent using starch-iodide paper. If a positive test (blue–black color) is obtained, a small spatula tip of sodium bisulfite is added until a negative test is observed. The solution is vacuum filtered to isolate the insoluble product and washed thoroughly with DI water. The product is dried for 5 min or more using a metal spatula to loosen any caked solid. The crude product is of sufficient purity for analysis or can be recrystallized from an 80:20 mixture of isopropanol/ethyl acetate mixture as the solubilizing solvent and hot water as the added crystallizing solvent. This three solvent system allows crystallization in the time allotted a teaching laboratory experiment and was based on a combination of the solvent systems presented used in the Doxsee and Hutchison procedure ( Citation16). The product is characterized by melting point (mp 183–185°C) and 1H NMR spectroscopy. Because the product is sparingly soluble in chloroform the 1H NMR sample is prepared using 10–15 mg of compound dissolved in 0.5–0.7 mL chloroform-d (CDCl3) and any undissolved material is removed using a small magnesium sulfate (MgSO4) microfiltration pipette. A 13C NMR spectrum and/or IR spectrum is optionally provided.
Suzuki-Miyaura cross-coupling reaction of 5-iodovanillin with 4-methylphenyl boronic acid
Reflux Procedure (student protocol) – Recommend all work be performed in a hood
Into a 50-mL round-bottom flask containing a stir bar is added 5-iodovanillin (0.25 g, 0.90 mmol), 4-methylphenyl boronic acid (0.16 g, 1.2 mmol), K2CO3 (0.40 g, 2.9 mmol), 10% Pd/C (5 mg, 5 µmol Pd) and 15 mL of DI water. The heterogeneous mixture is vigorously refluxed for 30 min. After cooling, 2.9 mL of 2.0 M HCl is slowly added with stirring to acidify the product mixture. Ethyl acetate (8 mL) is added with stirring to dissolve all organic solids and a gravity filtration of the biphasic mixture separates the Pd/C catalyst. The organic and aqueous phases are separated and the aqueous phase is extracted further with ethyl acetate (2 × 10 mL). The combined organic phases are washed with brine and dried over anhydrous MgSO4. Concentration using a hot plate and light stream of air affords the crude product that can be directly analysed or recrystallized. The product was recrystallized by dissolving in warm acetone and adding water as a solvent pair recrystallization. The product is characterized by melting point (mp 148°C ( Citation25), we wish to report mp of 137–139°C) and 1H NMR spectroscopy.
Microwave procedure (research lab tested only)
A 35-mL microwave vessel containing a micro-stir bar is charged with 5-iodovanillin (0.25 g, 0.90 mmol), 4-methylphenyl boronic acid (0.16 g, 1.2 mmol), K2CO3 (0.40 g, 2.9 mmol), 10% Pd/C (5 mg, 5 µmol Pd) and 15 mL DI water. The microwave vessel is shaken and vented three times to dissolve the 5-iodovanillin and potassium carbonate, and to release any pressure build-up. The reaction is heated under microwave radiation (200 W) at 150°C for 5 min. After cooling, 2.9 mL of 2.0 M HCl is slowly added with stirring to acidify the product mixture. Ethyl acetate (8 mL) is added with stirring to dissolve all organic solids and a gravity filtration of the biphasic mixture separates the Pd/C catalyst. The organic and aqueous phases are separated and the aqueous phase is extracted further with ethyl acetate (2 × 10 mL). The combined organic phases are washed with brine and dried over anhydrous MgSO4. Concentration using a hot plate and light stream of air affords the crude product that can be directly analysed or recrystallized. The product is recrystallized by dissolving in warm acetone and adding water as a solvent pair recrystallization. The product is characterized by melting point (mp 148°C ( Citation25), mp of 137–139°C this paper) and 1H NMR spectroscopy.
Results and Discussion
Iodination of vanillin using Oxone® and KI in water
The described iodination of vanillin experiment was successfully performed in our organic chemistry laboratory course over nine semesters (including three summer sessions) by approximately 2500 students. The experiment was placed in the latter half to two-thirds of the semester since practiced NMR skills and recrystallization techniques are required. The synthesis was performed individually. Students were first tasked with drawing the three possible mono-iodinated products and predicting which product is mostly likely formed based on substituent directing effects. They were also asked to consider how the aromatic 1H NMR coupling constants would be unique to each structure. A clicker question in the pre-laboratory lecture showed that approximately 54% of the students predicted the 5-iodovanillin as the product, with 30% predicting the least hindered position ortho to the aldehyde group, 4-hydroxy-2-iodo-5-methoxybenzaldehyde. The balance was distributed between the predictions of substitution on the 2-position and the methoxy group. Students were enthused by the opportunity to use vanillin, a familiar substance ubiquitously found in flavors and fragrances, in their organic chemistry laboratory setting.
The Oxone® solution is added dropwise with stirring to the flask containing the vanillin and KI in water to avoid collection of solids at the bottom and to maintain vigorous stirring. As previously mentioned, the purple color of iodine develops during reflux and the solution is quite turbid in appearance. Filtration of the product mixture and washing gives a tan solid which, upon adequate drying using the vacuum filtration apparatus can be analyzed directly. The crude 1H NMR spectrum generally contains 5% or less (most often undetectable quantities) of the starting vanillin which is easily quantifiable by the distinct aldehyde protons at 9.77 ppm for product versus 9.85 ppm for vanillin. Recrystallization of 5-iodovanillin is challenging and serves as a good evaluation of student technique. In designing the experiment and with pilot trials, a range of solvent systems was investigated for recrystallization in order to find a reproducible protocol easily managed in the 3 h and 50 min time frame allotted for the experiment. Use of either ethyl acetate alone or isopropanol/water, as reported in the literature ( Citation16), proved slow but may be acceptable if a multi-day recrystallization is possible. Alternatively, if a student did not isolate product the first day of the experiment, as mentioned above, a crude 1H NMR spectrum was obtained. Student crude yields were in the 65–75% range, whereas the recrystallized yields varied greatly, but on average were in the 30–40% range. With very few exceptions, students consistently obtained product for characterization. Successful recrystallizations yielded lustrous, gold crystals ().
To evaluate student learning, students complete a worksheet associated with the experiment (see Supporting Information). Experimental details and observations are reported as well as crude and recrystallized yields. The worksheet leads students through their characterizations and provides prompts to assess their understanding of purity, based on observed melting point and any peaks in the 1H NMR spectrum which are not attributable to product (e.g. recrystallization solvents). The 1H NMR spectrum of 5-iodovanillin () is instructive with its five distinguishable protons spanning a range of chemical shifts. Calculation of the J values for the aromatic protons Hc and Hd as 1.4 Hz identifies the coupling between these hydrogens as meta and supports the conclusion that 5-iodovanillin is the product. A spectrum with these aromatic protons expanded as an inset is made available to students on the course website so that the doublet can be clearly seen. Students are also asked to predict how the 1H NMR spectra of the other two possible regioisomers would differ from that obtained; i.e. the 2-iodovanillin would be predicted to have ortho proton coupling of 6–9 Hz, and the 6-iodovanillin’s para coupling expected to be 0–1 Hz.
Figure 2. 5-Iodovanillin student product and 1H NMR spectrum with inset of expanded aromatic region.
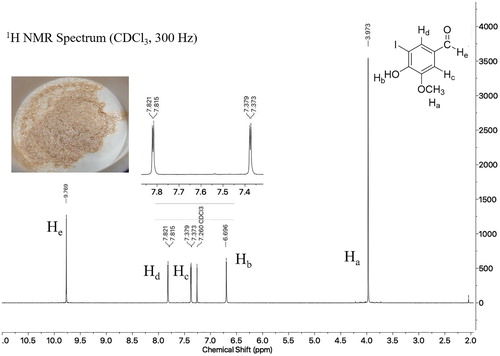
An IR spectrum of the 5-iodovanillin is generally provided for interpretation, as well as a 13C NMR spectrum. This experiment often serves as the primary introduction to 13C NMR spectroscopy in our course, because all eight carbons have unique chemical shifts and span the range of spectrum from 58 ppm to 193 ppm (see Supporting Information). Students can predict the chemical shifts of the different aromatic carbons by using “Additive parameters for predicting NMR chemical shifts or aromatic carbons in CDCl3” tables such as those found in Mohrig et al. ( Citation26).
Pre-laboratory green chemistry discussions for this experiment focus on the use of a renewable starting material, choice of water as a solvent, use of safer reactants compared to those typically used in EAS reactions, and minimization of waste since no extractions or co-solvents are used (with the exception of the recrystallization solvents). Post-lab worksheet questions on green chemistry vary between semesters but may include a question which asks students to identify a green chemistry principle that is not met in the experiment (most students recognize the poor atom economy inherent in the use of Oxone®) or an instructional question about E-factor based on provided masses of waste produced compared to their recovered yield (see Supporting Information).
Suzuki-Miyaura coupling reaction of 5-iodovanillin
The reflux version of the Suzuki-Miyaura reaction of 5-iodovanillin with 4-methylphenyl boronic acid was conducted in our organic chemistry teaching laboratory during one semester and two summer sessions. We elected to give an experimental procedure that does not identify the position of the iodo substituent, calling it a vanillin derivative, so as to not negate the discovery aspect of the prior experiment. Survey results from 384 students who completed the experiment during the 2017 summer and fall sessions indicated that the procedure was straightforward and easy to follow. Greater than 70% of the students isolated a crude yield of >51% and the average recrystallized yield was approximately 47%. Interestingly, the appearance of the crystalline products isolated ranged from white pearly crystals to light tan, with some students reporting yellowish crystals. Most students (3.8/5.0) enjoyed learning about the Suzuki reaction as important Nobel Prize winning chemistry and appreciated using a compound they synthesized themselves in a multi-step reaction. Two negative comments found in the open feedback section of the survey were that: (1) the Suzuki mechanism was not covered in their organic chemistry lecture course and was therefore very challenging to understand and (2) no new bench techniques were learned from the experiment. The latter comment will hopefully be circumvented by using the microwave procedure developed in our curriculum development research lab once funds are available to purchase the microwave for the teaching laboratories.
The product was characterized by melting point and 1H NMR spectroscopy. As noted in the experimental section, our research labs and students consistently determined a melting point lower (137–139°C) for the Suzuki product lower that the 148°C reported in the literature ( Citation25). The 1H NMR spectrum () was straightforward for students with respect to the methyl, methoxy, phenol, and aldehyde singlets and challenged the students to use chemical shift, coupling constants, and integration to identify the four different sets of aromatic protons. In particular, Hf and Hg overlap, presenting a puzzle many students, according to a survey, enjoyed solving.
A post-laboratory worksheet (see Supporting Information) expands upon the concepts presented in the experiment introduction, explores the mechanism of the reaction, and provides opportunity for interpretation of results. Students are asked to analyse the mechanism provided to write a reaction scheme which includes all by-products, highlighting reaction stoichiometry. The mol% of palladium is calculated and questions probe student understanding of the role of the catalyst, change in oxidation states, and use of carbon black. The worksheet also provides an expanded aromatic region spectrum to aid students with 1H NMR interpretation. Green chemistry worksheet questions vary but often include an atom economy and reaction efficiency calculation which they now have a context for from previous reaction calculations performed during the semester. In the worksheet example provided, a qualitative comparison to the process mass intensity (PMI) calculation gives students an appreciation for a metric more representative of the overall true environmental impact of a reaction.
Conclusion
In summary, vanillin, a familiar natural product, was used as the starting material for a two-step synthesis modeling an electrophilic aromatic substitution reaction and a palladium-catalyzed Suzuki-Miyaura cross-coupling reaction. These experiments were successfully implemented into an introductory organic chemistry laboratory course at a large university in which green chemistry is an important component of the curriculum. Aqueous reaction conditions were used in both experimental protocols, and green chemistry principles were addressed in each. The crystalline products, purified by recrystallization technique, provided educational 1H and 13C NMR spectroscopic analyses. Student feedback for the two-step synthesis was positive (3.8/5.0) with one student stating, “Overall I enjoyed seeing how a product synthesized in a previous experiment could be used in another experiment. I thought that it was a good way to emphasize the techniques that we’ve learned so far in lab.”
Acknowledgements
This work was funded by NSF Center for Sustainable Polymers, CHE-1413862; NSF Lando REU CHE-1359181; and the Robert C. Brasted Memorial Fund. The photo of the vanillin bottle in the graphical abstract designed by Beau C. Gilles, was purchased from Getty Images, iStock, order #2055468447, accompanied by a standard license agreement.
Disclosure statement
No potential conflict of interest was reported by the authors.
Notes on contributors
James J. Palesch earned a Bachelor of Science in Chemistry at the University of Minnesota in 2017. He worked beside Dr Jane Wissinger while participating in the summer Lando/NSF REU at the University of Minnesota and continued on as an undergraduate researcher. Currently, he works at the National Renewable Energy Laboratory in Golden, CO where he is a project manager within the Energy Systems Integration Facility.
Beau C. Gilles graduated with a Bachelor of Science in Chemistry in 2016 and worked for Jane E. Wissinger as an undergraduate researcher and as a Curriculum Development Teaching Assistant after graduation. He currently works as a Digital Media Generalist for a video production company where he does animation, sound design, and visual effects.
Jared Chycota earned a Bachelor of Science in Chemistry at the University of Minnesota in 2017. He was a directed studies student in the Wissinger lab during the fall of 2016. Jared continued on to earn a Master’s degree in Chemistry Education at the University of Minnesota and is currently a teacher in the St. Paul Public Schools District in St. Paul, Minnesota.
Moriana K. Haj earned a B.S. degree in Chemistry from the University of Minnesota in 2015. Her work with Professor Wissinger was supported by a 2013 Robert C. Brasted Memorial Fellowship, awarded for outstanding academic performance and potential for academic teaching. She completed undergraduate honors thesis research in Professor Thomas Hoye's laboratories. She is currently working on her Ph.D. in the Jacobsen research group at Harvard University.
Grant W. Fahnhorst is a PhD candidate at the University of Minnesota and currently studies under the guidance of Professor Thomas Hoye. Grant's research is focused on the development of new sustainable polymers with an emphasis on the conversion of biomass into reactive monomers. He graduated from Hamline University with a bachelor of science in chemistry in 2014.
Jane E. Wissinger is a Professor of Chemistry and Organic Laboratory Director at the University of Minnesota. Her teaching mission and research involves the development of new curriculum materials which model green chemistry principles, guided-inquiry pedagogies, and sustainable polymers. She is involved in numerous initiatives on a national level to promote the integration of green and sustainable chemistry in both high school and college level chemistry education programs.
ORCID
Jane E. Wissinger http://orcid.org/0000-0002-9240-3629
Additional information
Funding
References
- Dicks, A.P., The Greener Organic Chemistry Reaction Index. In Green Organic Chemistry in Lecture and Laboratory; Dicks, A.P., Ed. CRC Press Taylor & Francis Group: Boca Raton, FL, 2012; pp 259–271.
- Kemsley, J. Explosion at the University of Maryland. http://cenblog.org/the-safety-zone/2011/09/explosion-at-the-university-of-maryland/ (accessed Jul 18, 2018).
- Lang, P.T.; Harned, A.M.; Wissinger, J.E. Oxidation of Borneol to Camphor using Oxone and Catalytic Sodium Chloride: A Green Experiment for the Undergraduate Organic Chemistry Laboratory. J. Chem. Educ. 2011, 88 (5), 652–656. doi: 10.1021/ed100853f
- Hussain, H.; Green, I.R.; Ahmed, I. Journey Describing Applications of Oxone in Synthetic Chemistry. Chem. Revs. 2013, 113, 3329–3371. doi: 10.1021/cr3004373
- Naresh, M.; Arun Kumar, M.; Mahender Reddy, M.; Swamy, P.; Nanubolu, J.; Narender, N. Fast and Efficient Bromination of Aromatic Compounds with Ammonium Bromide and Oxone. Synthesis. (Mass) 2013, 45, 1497–1504. doi: 10.1055/s-0033-1338431
- Tamhankar, B.V.; Desai, U.V.; Mane, R.B.; Wadgaonkar, P.P.; Bedekar, A.V. A Simple and Practical Halogenation of Activated Arenes Using Potassium Halide and Oxone® in Water-Acetonitrile Medium. Synth. Comm. 2001, 31, 2021–2027. doi: 10.1081/SCC-100104419
- Firouzabadi, H.; Iranpoor, N.; Kazemi, S. Direct Halogenation of Organic Compounds with Halides using Oxone in Water – A Green Protocol. Can. J. Chem. 2009, 87, 1675–1681. doi: 10.1139/V09-125
- Krishna Mohan, K.V.V.; Narender, N.; Kulkarni, S.J. Simple and Regioselective Oxyiodination of Aromatic Compounds with Ammonium Iodide and Oxone®. Tetrahedron Lett. 2004, 45 (43), 8015–8018. doi: 10.1016/j.tetlet.2004.09.010
- Morra, B.; Dicks, A.P., Recent Progress in Green Undergraduate Organic Laboratory Design. In Green Chemistry Experiments in Undergraduate Laboratories; Fahey, J.T., Maelia, L. E., Ed. ACS Symposium Series: American Chemical Society, Washington, DC, 2016; Vol. 1233, pp 26–27.
- Hopson, R.; Lee, P.Y.B.; Hess, K.M. 1-Dimensional Selective Nuclear Overhauser Effect NMR Spectroscopy to Characterize Products from a Two-step Green Chemistry Synthesis. J. Chem. Educ. 2018, 95 (4), 641–647. doi: 10.1021/acs.jchemed.7b00494
- Sigma-Aldrich Safety Data Sheet: 5- Bromovanillin. https://www.sigmaaldrich.com/MSDS/MSDS/DisplayMSDSPage.do?country=US&language=en&productNumber=130605&brand=ALDRICH&PageToGoToURL=https%3A%2F%2Fwww.sigmaaldrich.com%2Fcatalog%2Fsearch%3Fterm%3D5-bromovanillin%26interface%3DAll%26N%3D0%26mode%3Dmatch%2520partialmax%26lang%3Den%26region%3DUS%26focus%3Dproduct (accessed Jul 25, 2018).
- Sigma-Aldrich Safety Data Sheet - 5-Iodovanillin. https://www.sigmaaldrich.com/MSDS/MSDS/DisplayMSDSPage.do?country=US&language=en&productNumber=129488&brand=ALDRICH&PageToGoToURL=https%3A%2F%2Fwww.sigmaaldrich.com%2Fcatalog%2Fsearch%3Fterm%3D5-iodovanillin%26interface%3DAll%26N%3D0%26mode%3Dmatch%2520partialmax%26lang%3Den%26region%3DUS%26focus%3Dproduct (accessed Jul 25, 2018).
- Varró, G.; Mattyasovszky, L.; Grün, A.; Simon, A.; Hegedüs, L.; Kádas, I. Highly Stereoselective Synthesis of Trans-Dihydronarciclasine Analogues. Synthesis. (Mass) 2018, 50, 625–643. doi: 10.1055/s-0036-1591514
- Tang, R.-J.; Milcent, T.; Crousse, B. Regioselective Halogenation of Arenes and Heterocycles in Hexafluoroisopropanol. J. Org. Chem. 2018, 83 (2), 930–938. doi: 10.1021/acs.joc.7b02920
- Sharma, V.; Srivastava, P.; Agarwal, D.D.; Diwedi, K. Iodination of Industrially Important Aromatic Compounds with Aqueous Potassium Triiodide. Russ. J. Org. Chem. 2016, 52 (3), 433–436. doi: 10.1134/S1070428016030234
- Doxsee, K.M.; Hutchinson, J.E. Green Organic Chemistry - Strategies, Tools and Laboratory Experiments; Brooks-Cole: Pacific Grove, CA, 2004. pp 186–188.
- Succaw, G.L.; Doxsee, K.M. Palladium-catalyzed Synthesis of a Benzofuran: A Case Study in the Development of a Green Chemistry Laboratory Experiment. Educ. Quim. 2009, 20 (4), 433–440.
- Nobelprize.org The Nobel Prize in Chemistry 2010. https://www.nobelprize.org/nobel_prizes/chemistry/laureates/2010/ (accessed Jul 25, 2018).
- Kotha, S.; Lahiri, K.; Kashinath, D. Recent Applications of the Suzuki–Miyaura Cross-Coupling Reaction in Organic Synthesis. Tetrahedron 2002, 58 (48), 9633–9695. doi: 10.1016/S0040-4020(02)01188-2
- Zhang, W.; Berkeley, B.W.C., Jr. Green Techniques for Organic Synthesis and Medicinal Chemistry; John Wiley & Sons, Ltd: West Sussex, UK, 2012, pp 155, 156, 158–160, 189–193, 195, 250–253, 276, 277, 304, 307–308, 333, 335, 336, 353–356, 517, 541.
- Hamilton, A.E.; Buxton, A.M.; Peeples, C.J.; Chalker, J.M. An Operationally Simple Aqueous Suzuki-Miyaura Cross-coupling Reaction for an Undergraduate Organic Chemistry Laboratory. J. Chem. Educ. 2013, 6 (11), 1509–1513. doi: 10.1021/ed4002333
- Costa, N.E.; Pelotte, A.L.; Simard, J.M.; Syvinski, C.A.; Deveau, A.M.; Green, D. Aqueous Suzuki Coupling Reactions: Synthesis of Ethyl (4-Phenylphenyl)acetate, a Biaryl with Anti-Arthritic Potential. J. Chem. Educ. 2012, 89 (8), 1064–1067. doi: 10.1021/ed200212p
- Hill, N.J.; Bowman, M.D.; Esselman, B.J.; Byron, S.D.; Kreitinger, J.; Leadbeater, N.E. Ligand-free Suzuki-Miyaura Coupling Reactions Using an Inexpensive Aqueous Palladium Source: A Synthetic and Computational Exercise for the Undergraduate Organic Chemistry Laboratory. J. Chem. Educ. 2014, 91 (7), 1054–1057. doi: 10.1021/ed400531w
- Aktoudianakis, E.; Chan, E.; Edward, A.R.; Jarosz, I.; Lee, V.; Mui, L.; Thatipamala, S.S.; Dicks, A.P. “Greening Up” the Suzuki Reaction. J. Chem. Educ. 2008, 85 (4), 555. doi: 10.1021/ed085p555
- Keesara, S.; Parvathaneni, S. A 2-((4-Arylpiperazin-1-yl)Methyl)Phenol Ligated Pd(II) Complex: An Efficient, Versatile Catalyst for Suzuki–Miyaura Cross-Coupling Reactions. New J. Chem. 2016, 40, 7596–7603. doi: 10.1039/C5NJ03450G
- Mohrig, J.R.; Alberg, D.G.; Hofmeister, G.E.; Schatz, P.F.; Hammond, C.N. Laboratory Techniques in Organic Chemistry: Supporting Inquiry-Driven Experiments. 4h ed.; W. H. Freeman and Company: New York, NY, 2014, pp 422–424.