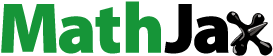
ABSTRACT
Cadmium(II) is well known for its toxicity and its removal is a prominent task in the field of environmental research. Trifolium resupinatum (TR) has been investigated for the biosorptive removal of Cd(II). The material was characterized by FTIR and SEM. These well established the nature of functional groups and the surface morphology. The effects of various process parameters including time of contact, concentration, pH and temperature were optimized. The maximum removal was observed at 20 min and a pH of7. The equilibrium was described by the Langmuir model showing the maximum biosorption of 31.95 mg/g. The binding of Cd(II) ions was found to be endothermic in nature. The biosorbent from T. resupinatum was found to adsorb potentially the toxic metal ions from its aqueous solutions.
GRAPHICAL ABSTRACT
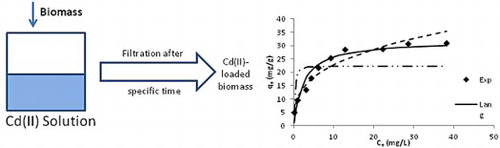
Introduction
Technological development by human beings has additionally resulted into contamination of water bodies with harmful substances and toxic materials above limits set by WHO and EPA. Being non-biodegradable, metal ions, especially Cd(II), are at the top of these pollutants. anthropogenic disturbances have resulted into environmental pollution through discharge of heavy metals especially cadmium (Citation1–3). Cadmium- released by various industries results in bioaccumulation into the food chain and thus considered for global concern for living organisms. The wastes produced by various anthropogenic activities including electroplating industrial waste, Ni–Cd batteries, alloys and effluents from pigment and textile industries, cause Cd(II) levels to increase in water bodies (Citation4–6). Such accumulation in biota and living species causes upset in the activity of major body organs such as kidneys, lungs and liver. The popular disaster of itai-itai was caused by contamination of cadmium in rivers of Japan (Citation7). It has been prescribed by WHO that the permissible limit of cadmium is 0.005 mg/L in drinking water. Environmental Regulation Authority has suggested the concentration of cadmium lower than 2.0 mg/L before its release into water framework (Citation8).
Many established methods have been used for heavy metals recovery from waste water like osmosis membrane technology, precipitation, filtration and electrochemical treatment (Citation9). These methods are not only expensive but generate secondary pollutants (Citation10). Due to counterproductive results at low concentration of Cd(II), these methods are being regarded as disadvantageous. Biosorption has come out as headway towards non-hazardous environment-friendly, economical and upstanding technology playing marvelous role in this area. Both organic and inorganic materials can be removed from their solutions by biosorption (Citation11) using low cost and easily available biosorbent materials (Citation12).
Trifolium resupinatum is a semi-erract annual legume reseeded as winter forge crop and can survive even at −12°C. It is a native of Turkey, Afghanistan, Pakistan, Greece, Iran and Iraq. It is cultivated in a wide range of soils but it is best suited for low lying areas with well drained clay loam and airy texture soils with pH from 5 to 8. It has been successfully grown in areas that receive between 450 and 600 mm of annual rainfall. This crop has been widely used as green fertilizer, grazing or hay production (Citation13–15). High production of this material indicates that the biomass material can be easily achieved from this plant. To the best of our knowledge, no study about the use of this plant material for Cd(II) removal has been reported in the literature.
The purpose of this work was to make a detailed analysis of various parameters as well as factors which affect the biosorption of Cd(II). The surface of the plant material has been characterized using various techniques. The factors like change in dose of material, contact time, pH and Cd(II) concentration, affecting the process have been optimized. The mathematical models to study the kinetics and equilibrium have also been studied. For the purpose, nonlinear approach was selected.
Materials and methods
All the AR grade chemicals used were purchased from Merck (Germany). The stock solution (1000 mg/L) of metal ion was prepared from the salt, Cd(NO3)2.4H2O using deionized water. The solutions of desired concentrations were prepared by diluting the stock solution.
Collection and pretreatment of biomass
The branches of T. resupinatum used as biosorbent were taken from verdant grounds of neighboring areas of University of the Punjab Lahore, in February 2015. Following the washing and drying in sunshine, the biomass was ground to fine powder. Then it was passed through 80-mesh sieve (ASTM standards) and the obtained fraction was washed with water to get rid of dust particles and other impurities. Ending up with oven drying till constant weight, the material was kept in desiccator for further use. The material before use was soaked in nitric acid (0.1M) for 3 h to attain chemical activation and to remove soluble moieties. During filtration, the biomass was washed many times with distilled water until a pH of 7 was achieved. The biomass was dried till constant mass and stored in airtight container for further use. The material was termed as TR.
The obtained material was characterized by its point of zero charge, bulk density and ash contents using the standard protocols. The surface morphology was studied by scanning electron microscopy (SEM) and the functional groups were identified using Fourier transformed infrared (FTIR) spectrum.
Batch biosorption experiment
Batch method was used to investigate the effectiveness of T. resupinatum (TR) biomass for the removal of Cd(II) from its aqueous solutions. In a typical experiment, 50 mL of Cd(II) solution of known concentration (10–100 mg/L) at a specific solution pH (between 3 and 8) was taken in a 250 mL conical flask and a known amount of TR (0.1–1.0 g) was added. The mixture was agitated on an orbital shaker at 150 rpm for a predefined interval of time (5–120 min). After filtration through Whattman filter paper no. 1, the remaining concentration of Cd(II) was determined using atomic absorption spectrophotometer (AAnalyst 100, Perkin Elmer) at the standard operating conditions. The amount of Cd(II) sorbed by TR was determined using Equation (1)(1)
(1) where qe is biosorbent uptake capacity at equilibrium (mg/g), v is the volume of solution (mL), m is the mass of biosorbent (mg), Co is the initial concentration and Ce is the equilibrium concentration of metal ion (mg/L). The effects of factors were studied in the given ranges using the same method.
Results and discussion
Characterization of the sorbents
Bulk density of the biosorbent was 0.237 g cm−3, the moisture content was found to be 16% and the ash content was 14% by weight. Scanning electron microscopy is a powerful tool to investigate surface morphology of biosorbent by using focused electron bean on the surface to create an image (Citation16). In particular, this technique allows in evaluation of morphological changes on the biomass surface. These may include changes in cell wall structure after metal ions binding onto the surface. This technique provides qualitative information of the surface structure. represents the surface morphology of biomass before and after biosorption of Cd(II) ions through scanning electron microscope. Before binding of Cd(II) ions, the surface of T. resupinatum was very porous and rough. Such a surface is suitable for binding of metal ions (Citation17, Citation18). After adsorption of Cd(II) ions, the topography of T. resupinatum was modified into relatively smooth, heterogeneous and delicate surface due to filling of pores with metal ions.
Figure 1. Scanning electron micrographs for the studied biosorbent (T. resupinatum) before (a) and after (b) binding of Cd(II) ions.
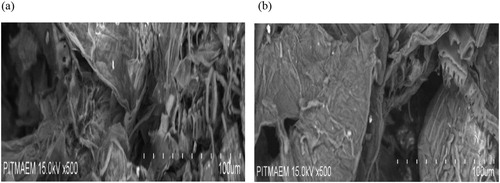
FTIR analysis was performed to investigate the functional groups in T. resupinatum which might be involved in binding of Cd(II) ions and the possible ways of interactions between adsorbate (Cd(II) ions) and adsorbent (T. resupinatum). FTIR spectra of T. resupinatum before and after biosorption of Cd(II) ions are shown in in the range of 4000–500 cm−1. It was noted that various functional groups such as carboxylic, amino, hydroxyl and carbonyl were involved to adsorb Cd(II) ions on surface of T. resupinatum. Some prominent FTIR spectral peaks of before and after biosorption are shown in . The band at wavenumber 3280 cm−1 was shifted to 3344 cm−1, after metal binding, showing the involvement of O–H and N–H functional groups. The carboxylate stretching was observed at 1590 cm−1 which shifted to 1599 cm−1 after biosorption of Cd(II) ions. The shift in positions of other bands such as C–N, C = O, C–O, C–C was also observed. Metal oxygen peak (M–O bond) was observed at 559, 542 cm−1, confirming the attachment of Cd(II) ions (Citation19, Citation20). Thus it was found that oxygen and nitrogen containing functional groups were responsible for metal attachment.
Figure 2. FTIR spectrum for T. resupinatum biosorbent before (a) and after (b) biosorption of Cd(II) ions.
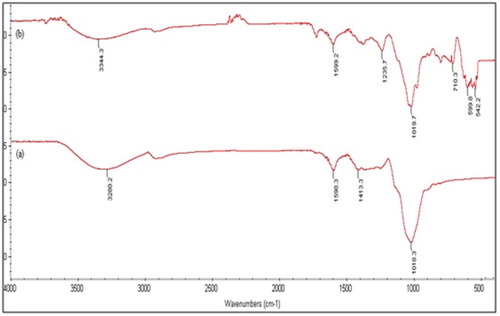
Table 1. Surface functional groups of T. resupinatum involved in biosorption of Cd(II) ions.
Batch adsorption experiments
Effect of biosorbent dosage
Adsorbent dose is an important parameter that affects adsorption capacity. It helps in deciding the optimum amount of biomass required to uptake maximum possible metal ions under a given set of conditions. The adsorption capacity gradually decreases as biosorbent dose is increased. The increase in adsorbent dose may cause aggregation of adsorbent and consequently the available adsorption sites decrease (Citation21). Another reason may be inter-particle interactions resulting from high adsorbent dose that can lead to the decrease in the total surface area of adsorbent and an increase in diffusional path length (Citation22).
The biosorbent dosage was studied in the ranges 2–20 g/L (0.1–1 g per 50 mL of solution). As shown in , the capacity was found to decrease with increase in dose. Since the concentration of Cd(II) ions was not changed, so for the finite number of ions, the adsorption sites were increased, indicating the decrease in the capacity. The optimum dose of 2 g/L (0.1 g per 50 mL of solution) was selected due to the best capacity found at 50 mg/L of Cd(II) ions.
Effect of contact time
The studies of contact time are very significant as these help optimize and attain minimum time required for the removal of maximum amount of metal ions from solution and hence support in scaling up the process. The equilibrium time, infact, assists to study biosorption mechanism and thus helps find out pathway towards determination of rate of biosorption. With the help of kinetic data, modeling and design of transport mechanism can be well described. The effect of contact time on cadmium ions sorption was investigated for a duration of 1–120 min. As shown in , the rapid binding of Cd(II) was observed during initial 5 min. The equilibrium was achieved in 20 min under the present experimental conditions. When time was increased from 5 min to 20 min (∼ 400% increase in time), the sorbed amount of Cd(II) was found to increase from 4.790 mg/g to 4.941 mg/g (3.15% increase). There was no observable change in the biosorbed amount of Cd(II) after 20 min. Hence, it was found that 20 min was sufficient time to achieve equilibrium in Cd(II)-TR system. The equilibrium time, in the present case, was found to be shorter than a number of studies reported in the literature for the biosorptive removal of Cd(II). shows such data for the purpose of comparison.
Figure 4. Effect of contact time on binding of Cd(II) by T. resupinatum (metal ions concentration of 50 mg/L in a volume of 50 mL and a dose of 2 g/L). exp is the experimental values of q. PFO is the pseudo-first-order and PFO is the PSO kinetic model under the studies set of conditions.
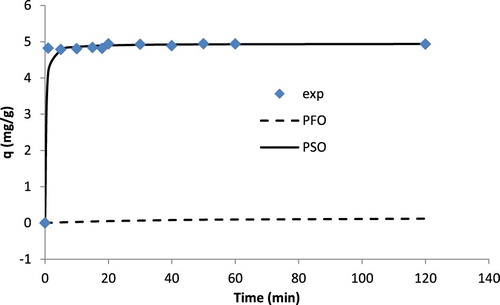
Table 2. Comparison of the potential of binding of Cd(II) by TR with other similar biosorbents *(L = Langmuir, DR = Dubinin–Radushkevich, PSO = Pseudo-second-order and PFO = Pseudo-first-order model).
The obtained data from time of contact studies were utilized for the studies of the kinetics of Cd(II) biosorption. It was found in the literature that biosorption of metal ions usually followed pseudo-first-order (PFO) and pseudo-second-order (PSO) models. Hence these two models were used for the present studies. The nonlinear approach was used to study the goodness-of-fit of the mathematical models. The equations for both of the models are given in Equations (2) and (3) as under.(2)
(2)
(3)
(3) where k1 and k2 are the rate constants for the PFO and PSO models respectively. The goodness-of-the-fit was determined using the root mean square error (RMSE) as given in Equation (4).
(4)
(4) where qt(exp) and qt(cal) are the experimental and the model's calculated biosorption capacity values at a given time t. The fitting of the two models is shown in . The dotted line expresses the values as calculated from PFO. The solid line shows the values obtained from PSO. The parameters for both the models are shown in . As can be observed (), PSO was better in expressing the experimental values. It was also obvious from the RMSE values for both the models. RMSE value for PFO was 5.122 and that for PSO was 0.2586, showing a better fit. In addition, PSO model's calculated qe value was closer to that obtained experimentally with a D% (=100 x (qe(cal)−qe(exp))/qe(exp)) of only 0.09% as compared to that for PFO (−97.15%). These indicators show that PSO was better than PFO in explaining the kinetics of Cd(II)-TR system. Most of the studies reported in the literature for Cd(II) biosorption also supports the observation, as shown in .
Table 3. Parameters for the kinetic modeling for Cd(II)-TR system.
Effect of cadmium ion concentration
The initial concentration of metal ions is a driving force for the binding of metal ions with the biosorbent material. Greater initial concentration indicates a greater binding behavior for the available finite binding sites present in the biosorbent. The effect of initial Cd(II) concentration was studied in 10–100 mg/L range. It was found that the increase in the initial concentration resulted in the increased biosorption capacity (Figure not shown).
The effect of concentration also provides information about the equilibrium process. The equilibrium concentration and the respective capacities are used to study the process by the use of various mathematical models. Freundlich (F), Langmuir (L) and Dubinin–Radushkevich (DR) models are the most applied models for biosorption of divalent metal ions. The equations for these models are shown in Equations (5), (6) and (7) respectively.(5)
(5)
(6)
(6)
(7)
(7) where β is expressed in mol2/J. It is used to calculate the mean free energy of adsorption E (1/√2β). The other parameter ε (RT ln(1 + 1/Ce)) is Polanyi potential expressed in J/mol. The nonlinear fitting of the model was studied using the RMSE.
The fitting of the models for the obtained data is shown in . The calculated values of the respective parameters are given in . KF for Freundlich model is too high, indicating the noncompliance of this model for experimental data. The experimental data was used to draw the Langmuir model curve. The maximum biosorption capacity qm was found to be 31.95 mg/g. It was very close to the experimental qe value as at the studied set of conditions. The Langmuir constant KL was found to be 0.4146. The dimensionless constant RL (=1/(1 + KLC0)) was calculated to be 0.0236–0.1943. The values being within 0 and 1; the adsorption was found to be favorable. The specific surface area SL (=NA qm A/M) was determined to be 6.40 m2/g. Here A is the cross sectional area of on Cd(II) ion (3.73 × 10−20m2), NA is the Avogadro number and M is the molecular mass of cadmium. The fitting of DR model was also studied. The capacity of biomass was calculated to be 22.15 mg/g. The mean free energy of adsorption was found to be 2.83 × 10−4 J/mol. This indicated that the nature of adsorption was physical adsorption. The goodness-of-fit of these studied models was evaluated using the RMSE values. The RMSE value was found minimum for the Langmuir model. This showed that the experimental data was well explained using the Langmuir model. The constants and other related parameters for Freundlich and DR models were not reliable due to noncompliance of models with the experimental data. Hence, the Cd(II)-TR system followed the Langmuir model forming a monolayer of Cd(II) ions on the surface of biomass. The biosorption capacity for Cd(II) was found to be greater than a number of similar materials reported in the literature ().
Figure 5. Effect of change in concentration on the biosorption of Cd(II) ions by T. resupinatum (50 mL, 20 min). Exp is the experimental qe values. Lang is the Langmuir model, Freu is the Freundlich model and DR is the qe values for DR model.
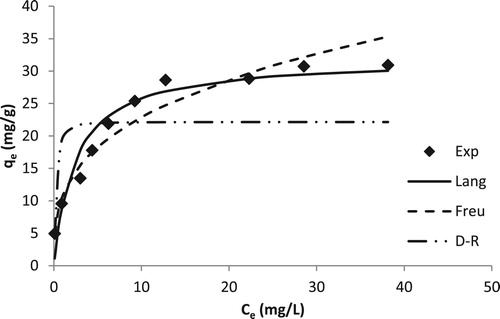
Table 4. Parameters determined for Freundlich, Langmuir and DR equilibrium models.
Effect of pH
The pH of the solution is a very important parameter since it controls the speciation of metal ions as well as the charge on the surface of the biomass. The surface charge is dependent on zero point charge pH (pHpzc) of the material. The pHpzc of TR biomass was found to be 6.3. Below this solution pH, the surface of the material becomes positively charged. Above pH of 6.3, the surface becomes negatively charged. This positive charge can also be explained on the basis of protonation of the functional groups present on the surface of biomass. At highly acidic pH, the functional groups containing O− and N− are protonated due to high potential of H+ ions. This protonation decreases with increase in pH since the concentration of H+ ions decrease. Thus increase in pH beyond pHpzc reverses the process and the functional groups start ionizing, although the pH of ionization is not the same for every functional group. It can be shown in the following scheme (Scheme 1).
In addition, cadmium is present as free Cd(II) ions below pH 7.5 after which it starts forming the precipitates as Cd(OH)2. These precipitates are not wanted as these are not available for metal-biomass interactions. Hence the upper limit of effect of pH study was kept till pH 8. shows the effect of pH. It was found that the metal binding increased with increase in the pH of solution. This rise was observed till a pH of 7, afterwards, there was a sudden increase in the qe values. This sudden rise was possibly due to precipitation of Cd(II) ions. The smaller qe at lower pH values was due to the positively charged surface of biomass repelling Cd(II) ions. With increase in pH, this repulsion decreased and the qe increased. It was found that TR was able to bind maximum Cd(II) ions at a pH of 7. This is in accordance with the reported studies in literature; that Cd(II) ions were adsorbed on the surface of various biosorbents in slightly acidic or neutral pH values.
Effect of temperature
Temperature is another important factor influencing the biosorption process. It deals with the studies of thermodynamics of the process and is directly linked with the kinetic energy of the metal ions. The increase in temperature would favor or oppose the metal biosorption process depending upon the route of energy involved. An endothermic process will be favored and exothermic process will be opposed by the increase in temperature. The effect of temperature was studied in 20–50°C (293–323 K) to determine the thermodynamic parameters. As shown in , the biosorption capacity increased slightly with increase in temperature. This indicates the endothermic nature of adsorption. This phenomenon may be due to two factors; first is raise in activated sites and second is decrease in thickness of boundary of metal ions in layer. Furthermore, reduced biosorption above particular temperature can be owing to destruction or dismantle of active sites. Thermodynamic parameters were determined using the following equation(9)
(9) where ΔG° (−RT ln Kd) is the change in standard free energy, ΔH° is the change in standard enthalpy and ΔS° is the change in standard entropy. Kd ((C0−Ce)/Ce) is the distribution coefficient. It was observed that the values of ΔG° decreased with an increase in temperature, indicating the favorable higher binding at higher temperatures. The process was found to be spontaneous due to negative ΔG° values. Other thermodynamic parameters were determined from the T vs ΔG° plot () and reported in . The value of ΔH° was found positive showing that the process was endothermic in nature. The positive values of ΔS° reflected an increase in the disorderness at the solid–liquid interface. The inferences are in accordance with the studies reported in the literature for the biosorptive removal of Cd(II) and other divalent metal ions.
Figure 7. Effect of temperature variation on the biosorption of Cd(II) by T. resupinatum and thermodynamic studies. Right axis shows the variation of qe with T and left axis indicates the change in standard free energy with T.
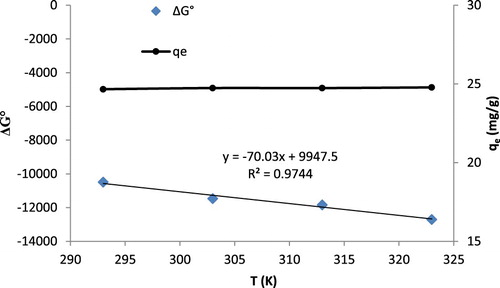
Table 5. Thermodynamic parameters for the biosorption of Cd(II) ions by T. resupinatum biosorbent.
Conclusions
T. resupinatum biomass is relatively a novel biosorbent for the binding of Cd(II) ions from its aqueous solutions. In current work, the potential of T. resupinatum was studied for the removal of Cd(II) ions. The material was characterized using available techniques including SEM and FTIR. Scanning electron microscopy reveals many hollow cavities on the surface as well as micro pores on T. resupinatum. FTIR studies represented alcoholic, carboxylic, ether and carbonyl groups in the structure of TR, that were found responsible for Cd(II) binding. TR exhibited good removal at pH 7 in removing metal ions from aqueous solutions in just 20 min. The mathematical models for kinetics and equilibrium were applied on the respective experimental data. Langmuir model described the equilibrium with biosorption capacity of 31.95 mg/g. PSO model explained the kinetics of the process. The endothermic nature of the process was confirmed from the changes in standard free energy. The material was found suitable for the binding of Cd(II) ions from its aqueous solutions.
Disclosure statement
No potential conflict of interest was reported by the authors.
Notes on contributors
Asif Masaud Khurram, is the Ph.D. scholar in the Institute of Chemistry, University of the Punjab, Lahore. His research area is about the water treatment processes. Currently, he is working as an Assistant Professor in the Govt. Science College, Lahore.
Umar Farooq is working as Assistant Professor of Analytical Chemistry in the Institute of Chemistry, University of the Punjab, earned his M.Sc. Chemistry with distinction from The Islamia University of Bahawalpur in 2003. He did Ph.D. in 2011 under HEC Indigenous Fellowship Program. His area of specialization is Analytical/Environmental Chemistry. He has more than 30 publications in International Impact Factor Journals, including three review articles. His research interests include development of environmentally friendly modified biosorbents for the treatment of polluted water layers.
Muhammad Makshoof Athar, Professor, Institute of Chemistry, University of the Punjab, Lahore, has post-doctoral experience from Ryerson University, Canada. He has more than 100 research articles to his credit including a number of reviews. His field of interest is applied/analytical chemistry, especially associated with the Environmental issues. He is also interested in the development of lowcost chromatographic and spectroscopic methods of analysis and polymer chemistry.
Muhammad Salman got his M.Sc. and M.Phil. in Applied Chemistry from the Institute of Chemistry, University of the Punjab in 2006 and 2011, respectively. He completed his Ph.D. in December 2015. He is working as Assistant Professor in the same institute since October 2014. His field of interest is Applied Chemistry, especially associated with environmental issues and production of chemicals. He has published above 60 research articles including one book and a book chapter in the field of Applied Chemistry.
ORCID
Umar Farooq http://orcid.org/0000-0003-0148-1013
Muhammad Salman http://orcid.org/0000-0003-4642-5891
Additional information
Funding
References
- Boening, D.W. Chemosphere 2000, 40, 1335–1351. doi: 10.1016/S0045-6535(99)00283-0
- Celik, A.; Demirbas, A. Energy Sources 2005, 27, 1167–1177. doi: 10.1080/00908310490479583
- Gonzalez, A.R.; Ndung'u, K.; Flegal, A.R. Env. Sci. Technol. 2005, 39, 5505–5511. doi: 10.1021/es048835n
- Cheung, C.W.; Porter, J.F.; Mckay, G. Water Res. 2001, 35, 605–612. doi: 10.1016/S0043-1354(00)00306-7
- Kadirvelu, K.; Thamaraiselvi, K.; Namasivayam, C. Bioresour. Technol. 2001, 76, 63–65. doi: 10.1016/S0960-8524(00)00072-9
- Kulkarni, S.J.; Kaware, J.P. Int. J. Eng. Sci. Innov. Technol. 2014, 1 (8), 61–65.
- Ossman, M.E.; Mansour, M.S. Int. J. Ind. Chem. 2013, 4, 1–7. doi: 10.1186/2228-5547-4-13
- Panda, G.C.; Das, S.K.; Chatterjee, S.; Maity, P.B.; Bandopadhyay, T.S.; Guha, A.K. Colloid. Surfac. B: Biointerfac. 2006, 50, 49–54. doi: 10.1016/j.colsurfb.2006.03.022
- Rao, K.S.; Anand, S.; Venkateswarlu, P. Indi. J. Chem. Technol. 2010, 17, 329–336.
- Mahmoud, A.E.D.; Fawzy, M. J. Bioremed. Biodegrad. 2015, 6, 1–7. doi: 10.4172/2155-6199.1000304
- Das, S.K.; Guha, A.K. Colloid. Surfac. B: Biointerfac. 2007, 60, 46–54. doi: 10.1016/j.colsurfb.2007.05.021
- Rajeswari, M.; Agrawal, P.; Pavithra, S.; Sandhya, G.R.P.; Pavithra, G.M. Biotechnol. Bioproc. Eng. 2013, 18, 321–325. doi: 10.1007/s12257-012-0424-4
- Celen, A.E.; Avcioglu, R.G.; Uzun, A. Crop Prot. 2006, 25, 496–500. doi: 10.1016/j.cropro.2005.08.006
- Tekeli, A.S.; Ates, E. Poljeprivreda. 2008, 14, 21–26.
- Tekeli, A.S.; Ates, E.; Varol, F. J. Cent. Europ. Agr. 2005, 6, 323–330.
- Reategui, M.; Malonado, H.; Ly, M.; Guibal, E. Appl. Biochem. Biotechnol. 2010, 162, 805–822. doi: 10.1007/s12010-010-8912-5
- Nies, D.H. Appl. Microbiol. Biotechnol. 1999, 51, 730–750. doi: 10.1007/s002530051457
- Sekhar, K.C.; Kamala, C.T.; Chary, N.S.A.; Sastry, R.K.; Rao, T.N.; Vairamani, M. J. Haz. Mater. 2004, 108, 111–117. doi: 10.1016/j.jhazmat.2004.01.013
- Burneau, A.; Carteret, C. Phy. Chem. Chem. Phys. 2000, 14, 3217–3226. doi: 10.1039/b002863k
- Cardoso, N.F.; Lima, E.C.; Pinto, I.S.; Amavisca, C.V.; Royer, B.; Pinto, R.B.; Alencar, W.S.; Pereira, S.F. J. Env. Manag. 2011, 92, 1237–1247. doi: 10.1016/j.jenvman.2010.12.010
- Christoforidis, A.K.; Orfanidis, S.; Papageorgiou, S.K.; Lazaridou, A.N.; Favvas, E.P.; Mitropoulos, A.C. Chem. Eng. J. 2015, 277, 334–340. doi: 10.1016/j.cej.2015.04.138
- Shukla, A.; Zhang, Y.H.; Dubey, P.; Margrave, J.L.; Shukla, S.S. J. Haz. Mater. 2002, 95, 137–152. doi: 10.1016/S0304-3894(02)00089-4
- Fagundes-Klen, M.R.; Ferri, P.; Martins, T.D.; Tavares, C.R.G.; Silva, E.A. Biochem. Eng. J. 2007, 34, 136–146. doi: 10.1016/j.bej.2006.11.023
- Schiewer, S.; Patil, S.B. Bioresour. Technol. 2008, 99, 1896–1903. doi: 10.1016/j.biortech.2007.03.060
- Argun, M.E.; Dursun, S. Bioresour. Technol. 2008, 99, 2516–2527. doi: 10.1016/j.biortech.2007.04.037
- Naiya, T.K.; Chowdhury, P.; Bhattacharya, K.; Das, S.K. Chem. Eng. J. 2009, 148, 68–79. doi: 10.1016/j.cej.2008.08.002
- Ibrahim, H.S.; Ammar, N.S.; Soylak, M.; Ibrahim, M. Spectrochim. Acta A 2012, 96, 413–420. doi: 10.1016/j.saa.2012.05.039
- Dang, V.B.H.; Doan, H.D.; Dang-Vu, T.; Lohi, A. Bioresour. Technol. 2009, 100, 211–219. doi: 10.1016/j.biortech.2008.05.031
- Low, K.S.; Lee, C.K.; Liew, S.C. Process Biochem. 2000, 36, 59–64. doi: 10.1016/S0032-9592(00)00177-1
- Omorogie, M.O.; Babalola, J.O.; Unuabonah, E.I.; Gong, J.R. Bioresour. Technol. 2012, 118, 576–579. doi: 10.1016/j.biortech.2012.04.053
- Ho, Y.S.; Ofomaja, A.E. Biochem. Eng. J. 2006, 30, 117–123. doi: 10.1016/j.bej.2006.02.012
- Farooq, U.; Khan, M.A.; Athar, M.; Kozinski, J.A. Chem. Eng. J. 2011, 171, 400–410. doi: 10.1016/j.cej.2011.03.094