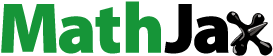
ABSTRACT
In this study, Corydalis yanhusuo extract acts as a green inhibitor for J55 steel in 3.5% NaCl solution saturated with CO2. Rapid resolution liquid chromatography shows the concentrations of Palmatine to be 0.6358 g/L, Tetrahydropalmatine 0.7715 g/L, and Corydaline 0.3755 g/L. Potentiodynamic polarization results suggest that Corydalis yanhusuo extract is a mixed-type inhibitor. The inhibition efficiency of Corydalis yanhusuo extract increases with the increase of concentration and reaches 92.36% at 5%v/v but decrease with the increased temperature. The inhibition efficiency is 69% at 80°C. The protective film formed on the metal surface was confirmed by Fourier transforms infrared spectroscopy, UV–visible, scanning electrochemical microscope and scanning electron microscope methods. The quantum chemical calculations predicate that Palmatine acts as the major component for inhibition performance.
GRAPHICAL ABSTRACT
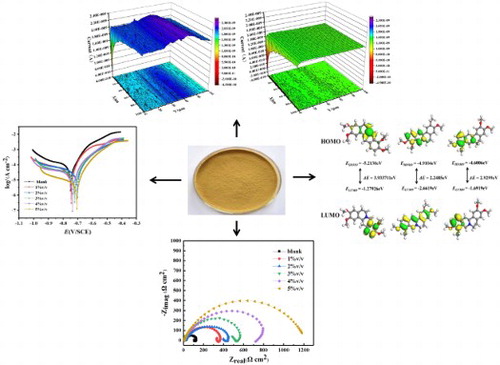
Introduction
J55 steel is one of the most common metals used in oil drilling due to its excellent mechanical properties and reasonable price, but its high corrosion risk makes it largely impossible for sustained use. It is well known that CO2 could dissolve in water and form carbonic acid (H2CO3) which is more destructive than hydrochloric acid (HCl) to metallic materials under the same pH conditions (Citation1,Citation2). CO2 corrosion, also known as sweet corrosion, tends to cause premature failure of oilfield production equipment, even huge economic losses. In order to address this problem, researchers have developed a variety of protective means, such as the use of corrosion resistant alloy materials, electrochemical protection, coating technology and corrosion inhibitors. Among them, adding corrosion inhibitor, due to its low cost, high efficiency and wide applicability, has been used in various fields, especially in petroleum extraction and refining (Citation3,Citation4). Nowadays, the combination of J55 steel and inhibitor has been among the most typical methods to protect J55 from corrosion in oil and gas field (Citation5–7). Until now, most efficient synthetic inhibitors have shown good corrosion inhibition. However, since environmental protection has received growing attention and environment legislation has become increasingly comprehensive, the high toxicity and low biodegradability are severe problems of commercial inhibitors. Therefore, environment-friendly inhibitors have drawn much more eyesight. The extract from plant leaves, seeds, flowers and peels can be used for steel protection because they contain different organic compounds which have hetero-atoms like N, S, O, P and other effective groups. These atoms or groups can form adsorption centers so that the molecules can adsorb onto the steel surface. In recent years, researchers have studied green inhibitors such as Lannea coromandelica leaf (Citation8), Retama monosperma (L.) Boiss. Seeds (Citation9), Azadirachta indica leaves (Citation10), Oxandra asbeckii (Citation11), Tagetes erecta (Citation12), Mansoa alliacea (Citation13), lychee fruit (Citation14), Egyptian licorice (Citation15), morus alba pendula leaves (Citation16), Aniba rosaeodora (Citation17), Ligularia fischeri (Citation18), Thymus vulgaris (Citation19), Ginkgo leaf (Citation20), Centaurea cyanus (Citation21), Rollinia occidentalis (Citation22) and Sunflower seed hull (Citation23). They have shown good corrosion inhibition performances in various conditions.
As a traditional Chinese medicine, Corydalis yanhusuo is very cheap and abundant. It contains numerous natural organic compounds including alkaloids, flavonoids, volatile oils and sugars. They contain a large quality of N–H, C=C, C=O and other functional groups which can provide electrons to the metal surface, forming a protective film, thus having the potential of being a corrosion inhibitor (Citation24). The present study seeks to investigate the inhibitive properties of Corydalis yanhusuo extract for J55 steel corrosion in 3.5% NaCl solution saturated with CO2. To meet this objective, potentiodynamic polarization curves, electrochemical impedance spectroscopy (EIS), Fourier transforms infrared spectroscopy (FT-IR), UV–visible spectroscopy, scanning electrochemical microscope (SECM), scanning electron microscope (SEM) and quantum chemical calculations are performed.
Experiment
Materials and preparations
The Corydalis yanhusuo extract used in this study was prepared from southeast China and was dried thoroughly before putting into use. The dry CHYS was washed with tap water, dried in shade and grounded into powder. The CHYS powder (50.0 g) was soaked in flask of one necked with absolute ethanol (300 ml, 99.9% purity) and let it stand for 48 h at room temperature. Then the solution was refluxed by a magnetic stirrer for 5 h at 65°C. After cooling to room temperature, the solution was filtered and concentrated to 100 ml.
The J55 steel used for all the experiments have the following composition (wt.%): C 0.24%, Si 0.22%, Mn 1.1%, P 0.103%, S 0.004%, Cr 0.5%, Ni 0.28%, Mo 0.021%, Cu 0.019% and Fe balance. Before the experiments, all J55 samples were polished with emery papers from 600 to 1200 grade and used distilled water to have them washed. Then the samples were degreased with acetone and absolute ethanol and dried at room temperature. The test solution was prepared with analytical grade NaCl (≧99.9% purity) and distilled water, injecting CO2 (99.99% purity) for 3 h until saturated.
Rapid resolution liquid chromatography
Rapid resolution liquid chromatography (RRLC) was employed to identify the phenolic components of the Corydalis yanhusuo extract. The analysis was performed using an Agilent 1290 chromatograph system (Agilent Technologies, Palo Alto, CA, USA). The test sample was separated using a ZORBAX Eclipse Plus C18 column (2.1 × 50 mm, 1.8 μm), with the absorbance peak observed at 280 nm. The mobile phases used for the tests were as follows: solvent A: 0.6% acetic acid solution (adjusted the pH to 5.0 by triethylamine) and solvent B: acetonitrile. The temperature of the column was set to 30°C with the flow-rate of 0.2 ml/min. the standard reference substances Palmatine (Shanghai Aladdin Bio-Chem Technology Co., LTD, ≥97% purity), Tetrahydropalmatine (≥97% purity) and Corydaline (≥98% purity) with different concentrations were used to identify the phenolic components of Corydalis yanhusuo extract and each concentration was repeated five times.
Weight-loss tests
For weight-loss tests, a series of silicon carbide papers (grades 600, 800, 1000 and 1200) were employed to abrade the surface of J55 steel. Then the surface was washed by distilled water and acetone, finally dried and weighted. After that, the specimens were immersed in 100 ml of 3.5% NaCl solution saturated with CO2 with and without various concentrations of Corydalis yanhusuo extract at different temperatures (40°C, 60°C and 80°C) which were kept for 72 h. Then samples were picked out and rinsed with tap water, then dried.
In order to guarantee the accuracy of all the above experiments, three sets of samples were measured to calculate their average values. The corrosion rate (CR) was calculated with the following equation at different temperatures:(1)
(1) where ΔW represents weight loss of sample (g) and S is the exposure area (m2). t denotes the immersion time (h).
The inhibition efficiency (η%) was calculated by the following equation:(2)
(2) where
and
are the corrosion rates of J55 steel in 3.5% NaCl solution saturated with CO2 with and without Corydalis yanhusuo extract, respectively.
Electrochemical measurements
The inhibition effects of the Corydalis yanhusuo extract in 3.5% NaCl solution on J55 steel was studied by EIS and polarization techniques with AUTOLAB PGSTAT302N electrochemical workstation. Electrochemical experiments were measured under a conventional three-electrode cell system in which J55 steel coupons with an exposed surface area of 1 cm2 used as working electrode. A platinum sheer electrode and a standard calomel electrode (SCE) were used as auxiliary and reference electrodes, respectively. Before the electrochemical measurement, a stabilization period (1 h) was allowed to ensure that the electrode reached a stable condition.
Polarization curves measurements were started by changing the electrode potential automatically from cathodic to the anodic at ±300 mV versus open circuit potential (OCP) at a scan rate of 1.0 mV/s. Before each potentiodynamic polarization test, −1.1 V vs. SCE is applied for the working electrode for 3 min to remove possible corrosion product films. Impendence measurements were carried out using AC signals of amplitude 10 mV peak to peak at OCP with the frequency range from 100 to 0.00001 kHz. All impedance data were fitted to appropriate circuits using the ZSimDemo 3.30d software.
The inhibition efficiency (ηI%) can be calculated by the following relation:(3)
(3) where
and
are the corrosion current density values without and with inhibitor, respectively.
The inhibition efficiency (ηR%) based on AC impedance was evaluated using the following equation:(4)
(4) where
and
are the charge transfer resistances in the absence and presence of the inhibitor.
UV–visible and FT-IR analysis
UV–visible absorption spectra were performed to samples of J55 steel immersed in 3.5% NaCl solution saturated with CO2 in the absence and presence of 5% Corydalis yanhusuo extract for 48 h. All spectra were carried out with UV-2600 instrument, SHIMADZU Company.
The FT-IR spectra were recorded on Nicolet 6700 spectrometer which connected with Omnic software using the KBr disk technique.
Surface analysis
The J55 steel of size 3 × 0.3 × 0.3 cm were immersed in 3.5% NaCl saturated with CO2 in the absence and presence of optimum concentration (5% v/v) of Corydalis yanhusuo extract for 24 h. Then, these samples were processed with a model of CHI900C electrochemical system based on scanning electrochemical microscopy studies. The system consists of a 10 μm platinum tip as the probe, an Ag/AgCl/KCl (saturated) reference electrode and a platinum counter electrode. The results were obtained at about 10 μm from the specimen surface (100 × 100 μm) at a scan rate of 1 μm/step.
The surface topographical information of J55 steel without and with different concentrations of Corydalis yanhusuo extract inhibitor were obtained using an SEM model ZEISS EV0 MA15 instrument. Chemical composition of the same steel sample surface was recorded by an energy-dispersive spectroscopy (EDX) detector.
Computational details
Quantum chemical calculations and MD simulations were performed with Materials studio software 7.0. As for the former, DMol3 module was used to optimize the geometries of the molecules. Then the generalized gradient approximation/Becke exchange plus Lee–Yang–Parr functional of DMol3 module were used to compute the exchange-functional without any symmetry constraint. The electrons distributions were calculated by a basis set of double number polarization.
Results and discussion
Rapid resolution liquid chromatography
The chromatogram of Corydalis yanhusuo extract identified by RRLC was shown in . The three peaks representing Palmatine, Tetrahydropalmatine and Corydaline, respectively, refer to the standard references.
Figure 1. RRLC chromatograms of Corydalis yanhusuo extract recorded at 280 nm in the optimized chromatography conditions. Peaks: 1, Tetrahydropalmatine; 2, Palmatine; and 3, Corydaline.
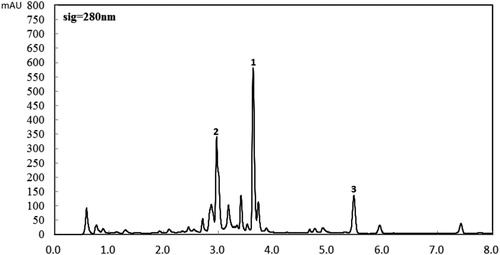
The calibration curves were constructed with five concentrations (0.2, 1, 1.5, 2, 4 g/L) of standard references. The parameters derived from the curves were shown in . Good linearity was shown for all the curves (R2 > 0.99) in the range of concentrations and the content of each alkaloid was determined: Palmatine, 0.6358 g/L, Tetrahydropalmatine 0.7715 g/L and Corydaline 0.3755 g/L.
Table 1. Analytical parameters of calibration curves of Corydalis yanhusuo extract.
Weight-loss measurements
Weight-loss is a typical method to investigate the relationship between corrosion rate and inhibitor concentrations. The data of weight-loss measurements for J55 steel in 3.5%NaCl solution saturated with CO2 in the absence and presence of different concentrations Corydalis yanhusuo extract at various temperatures are listed in . shows the relationships between inhibition efficiency and concentration at different temperatures. It can be observed from that the inhibition efficiency of 5%v/v Corydalis yanhusuo extract is 88% at 313 K. However, as temperature increases, inhibition efficiency shifts toward a negative direction, which indicates that temperature can significantly affect the adsorption process of Corydalis yanhusuo extract. The explanation for this phenomenon is that the increase of temperature accelerates the Brownian motion of the molecule, resulting in the desorption of corrosion inhibitor molecule from the metal surface. What is more, a higher temperature could destroy the active ingredients of the extract, thus decreasing the inhibition efficiency (Citation25).
Figure 2. Inhibition efficiency obtained by weight-loss tests at different concentrations of Corydalis yanhusuo extract at various temperatures.
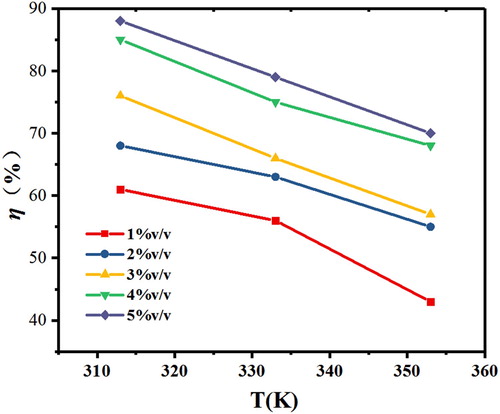
Table 2. The results obtained from weight-loss measurements.
Until now, a lot of natural extracts have been used as corrosion inhibitors the inhibition efficiencies of which have been investigated. shows the information of some plant extract inhibitors. By careful observation of these inhibition efficiencies and concentrations, it can be found that Corydalis yanhusuo extract has the potential to be an effective inhibitor.
Table 3. Previous natural products used as corrosion inhibitors in various media and their inhibition efficiency.
EIS measurements
The EIS of J55 steel in 3.5% NaCl solution saturated with CO2 in the absence and presence Corydalis yanhusuo extract at room temperature (25°C) are shown in . It can be seen that the impedance data of different inhibitor concentrations present a depressed semicircle, which is related to the heterogeneity and roughness of J55 steel surface (Citation26,Citation27). As the concentration of Corydalis yanhusuo extract increases, the radius of the impedance loop increases significantly, while the shapes of impedance loops do not change, indicating the mechanism of corrosion remain unchanged (Citation28).
Figure 3. The EIS plots obtained for the J55 steel in 3.5%NaCl solution saturated with CO2 without and with various concentrations of Corydalis yanhusuo extract.
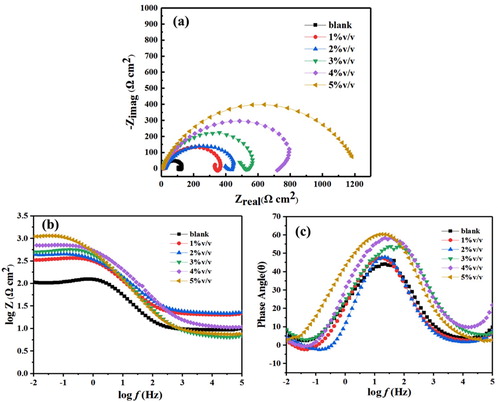
As shown in , the EIS spectra are analyzed by an equivalent circuit which contains solution resistance (RS), charge transfer resistance (Rct), inductive resistance (RL), constant phase element (CPE) and inductance (L). And the fitting data are listed in . Particularly, the value of CPE can be calculated by the following equation (Citation29,Citation30):(5)
(5) where Y0 represents the magnitude of CPE and ω is the angular frequency. j2
= −1 is an imaginary number and n is the CPE exponent which is a pure capacitor when n = 1and an inductor when n =−1. The values of Cdl are derived according to the Helmholtz model (Citation31):
(6)
(6)
Table 4. The relevant parameters obtained from Nyquist plots at different concentration of inhibitor for J55 steel in 3.5% NaCl solution saturated with CO2.
where ε0 is the permittivity of vacuum and ε is the local dielectric constant. The d is the thickness of the protective layer and A is the surface area of the electrode. It is obvious that the values of Rct increase and the values of Cdl decrease as the concentration of Corydalis yanhusuo extract become higher. The reason for this phenomenon is that on J55 surface a protective layer is formed which isolates the adsorption of the aggressive medium.
Further information can be found from the bode plot ((b)) and phase plot ((c)) that the slope between log|Z| and logf, as well as the maximum phase angle increased with the increase of concentrations of Corydalis yanhusuo extract, demonstrating the formation of a protective film formed on J55 steel surface so as to reduce the diffusion of metal (Citation32).
Potentiodynamic polarization curves
The mechanism of corrosion inhibition of Corydalis yanhusuo extract has been investigated by potentidynamic polarization curves and the results are shown in . The polarization parameters calculated from polarization curves are listed in .
Figure 5. The polarization curves plots obtained for the J55 steel in 3.5%NaCl solution saturated with CO2 without and with various concentrations of Corydalis yanhusuo extract.
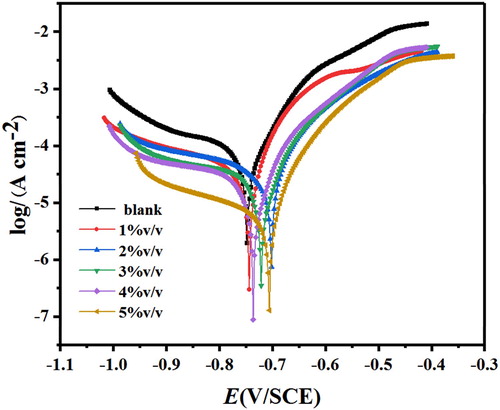
Table 5. The relevant parameters obtained from polarization curves at different concentration of inhibitor for J55 steel in 3.5% NaCl solution saturated with CO2.
As can be seen from , both anodic and cathodic curves shift toward a negative direction, but their shapes do not change. This indicates that Corydalis yanhusuo extract retards the anodic dissolution of metal as well as cathodic evolution of hydrogen gas but the mechanism has no change.
Closer scrutiny from reveals that the values of Ecorr move toward positive shift compared with the blank. However, the change is less than 85 mV, which indicates that CYSHE acts as a mixed-type inhibitor (Citation33,Citation34). It can be seen from that the values of βa and βc show irregular changes, which indicates both the anodic and cathodic reactions are retarded. The values of Icorr decrease obviously with the increasing Corydalis yanhusuo extract concentration, suggesting that the protective layer retards the electrode reaction. When Corydalis yanhusuo extract concentration reaches 5%v/v, the inhibition efficiency is 91.5%, which represents good inhibition for J55 steel in 3.5%NaCl solution saturated with CO2 environment. Furthermore, the surface coverage increases in the inhibitors, suggesting that a compete and the stable film is formed on the metal surface.
FT-IR and UV–visible analysis
The FT-IR result for Corydalis yanhusuo extract is shown in (a). The absorption band at 3411.14 cm−1 belongs to the stretching vibration of phenolic N–H. The peak at 3141.42 cm−1 can be attributed to C–H stretching vibration. The peaks at 2920.73 and 2855.34 cm−1 are attributed to C–H stretching vibration of lipids and aromatic rings. The band at 1739.65 cm−1 represents C=O stretching vibration. The band at 1625.22 cm−1 can be assigned to the stretching vibration of C=C. The vibration of the conjugated ring can be found at 1506.71 cm−1. The peak located at 1388.19 cm−1 is the C–N stretching vibration. The band at 1276.65 cm−1 is corresponding to C–O stretching vibration. The regions at 1098.03 and 1040.82 cm−1 are associated with the C–O stretching vibration of a benzene ring, and the absorption peaks at 1000–400 cm−1 are related to a benzene ring or aromatic heterocyclic substituent groups. Further information can be found that the peaks of N–H, C=O, C–N shift to 3309.69, 1741.44 and 1408.70 cm−1, respectively, suggest these functional groups are used for interaction with the metal surface, thus confirming the formation of adsorption film on the metal surface.
The UV–visible spectra of J55 steel immersed in 3.5%NaCl solution saturated with CO2 with and without Corydalis yanhusuo extract for 24 h are shown in (b). The adsorption bands of Corydalis yanhusuo extract at 229 and 280 nm represent the transition of π–π* and n–π* for the carbonyl, C=O and N–H groups, respectively (Citation35,Citation36). Meanwhile, the bands shift to 230 and 281.5 nm after the immersion of J55 steel, suggesting that the formation of the complex between metal surface and solution.
SECM analysis
SECM is useful for presenting local electrochemical activity and pitting. It can recognize the ion transfer processes and active/passive regions at the interface of steel in the micrometer range or below compared to other in-situ techniques (Citation37–39). The SECM tests are conducted in AC-amperometry mode for the three-dimensional diagram of the surfaces of J55 steels in the absence and presence of 5% v/v Corydalis yanhusuo extract, respectively. Before each experiment, the approach curves were used to define the distance between the probe and the substrate. shows the horizontal and vertical scanning directions of J55 steels surface color mapping figure in the absence and presence of Corydalis yanhusuo extract as visualized by SECM. It can be seen clearly that the surface maps have different colors under the same reference, indicating that the corrosion inhibitor changes the current of the surface. This is because the insulating surface obstructs the diffusion of oxygen towards the tip (Citation40). On the contrary, the current increases when the probe tip is near the metal surface without inhibitor (conducting surface). Comparing the currents across the corroded sample ((a)) and the specimens incorporated with Corydalis yanhusuo extract ((b)), the inhibitor adsorbed on J55 steel surface and formation film can be confirmed (Citation41).
SEM-EDX
The surface morphologies of J55 steel immersed in 3.5%NaCl solution saturated with CO2 in the absence and presence of Corydalis yanhusuo extract for 24 h are shown in . It can be seen from (a) that the J55 steel is corroded severely without Corydalis yanhusuo extract. The aggressive solution cause surface roughness and a lot of cracks. The EDX is adopted to investigate the result of which in (a) shows that the element content of the J55 surface is composed of 73.69% Fe, 9.52% oxygen and 9.01% Cl without the inhibitor. By comparing to the blank, the specimen immersed in 3.5%NaCl solution with Corydalis yanhusuo extract shows a smoother surface and the content of oxygen and chlorine are significantly reduced, demonstrating that the protective film is formed on metal surface.
Quantum chemical calculations
The RRLC has identified that etrahydropalmatine, Palmatine and Corydaline are the major components. In order to investigate the component responsible for the inhibitive property and the relationship between molecular structure and inhibition efficiency, quantum chemical calculations have been applied on the basis of frontier molecular orbital theory (FMOT). According to FMOT, The highest occupied molecular orbital (HOMO) represents the ability to donate electrons and the low unoccupied molecular orbital (LUMO) is related to the electron accepting ability (Citation42,Citation43). Moreover, higher value of EHOMO is more likely to donate electrons to empty orbital, while lower value of ELUMO has more tendencies of accepting electrons. Thus, the energy gap between HOMO and LUMO (ΔE = ELUMO − EHOMO) represents the capacity of electron stability which determines the adsorption stability of the inhibitor on the metal surface (Citation44,Citation45). The optimized structures, HOMO and LUMO of major components of Corydalis yanhusuo extract including Tetrahydropalmatine, Palmatine and Corydaline are shown in . The HOMOs of three alkaloids are mainly distributed in the ring-containing nitrogen atoms and the LUMOs are distributed in the benzene ring attached to the heterocyclic ring, suggesting that the nitrogen atoms as the active sites can be the adsorption center. As can be seen from that the order of ΔE values is Tetrahydropalmatine > Corydaline > Palmatine, which predicts that Palmatine has more tendency for absorption onthe metal surface.
Conclusion
The inhibition and adsorption of Corydalis yanhusuo extracts are investigated for J55 steel in 3.5% NaCl solution saturated with CO2 in this work. FT-IR spectroscopy and UV–visible spectroscopy were performed to characterize the extracts respectively. Weight-loss, EIS and polarization curves showed that the inhibition efficiency increases with the increase of inhibitor concentration and decreases with the increase of temperature. SEM-EDX and SECM confirmed the formation ofan adsorption film on the metal surface. The theoretical calculations verified that palamatine as one of the major components has more tendencies for adsorption.
Disclosure statement
No potential conflict of interest was reported by the authors.
Notes on contributors
Yuanhua Lin is a professor in the school of material science and engineering, at the southwestern petroleum university. He is also the fixed Researcher for National Key Laboratory of Oil and Gas Reservoir Geology and Development Engineering and the Director of CNPC Key Lab for Tubular Goods Engineering. His research interests are oil and gas drilling technology and oil well pipe materials.
Zhipeng Sun received his Bachelor's and Master's degrees in 2016 and 2019, respectively, from southwestern petroleum university, China. He is now pursuing his Ph.D. at the University of Science & Technology Beijing. The current research of him is focuses on the development of corrosion inhibitors.
Xi Luo now is an associate researcher in the Engineering Research Institute North China Branch Company of SINOPEC, Zhengzhou. He received his Master's degree from China University of Petroleum (East China). His research mainly engaged in gas production technology research.
Wanying Liu is an experimentalist in the school of material science and engineering, at the southwestern petroleum university. She is now pursuing her Ph.D. under the supervision of Prof. Ying Liu at the Sichuan university. Her research area mainly focuses on the corrosion and protection of metal materials and surface engineering technology.
Xuefeng Deng received his Master degree from China University of Petroleum (East China), majoring in the development of oil and gas filed. He is now working in SINOPEC for oil recovery engineering.
Jinchang Wang is an associate researcher in the SINOPEC. He received his Bachelor's and Master's degrees from Southwest Petroleum University. He is now pursuing his Ph.D. under the supervision of Prof. Yuanhua Lin. His research mainly engaged in drilling engineering and the corrosion of oil and gas materials.
Additional information
Funding
References
- Matjaž, F.; Jennifer, J. Corros. Sci. 2014, 86, 17–41.
- Xiang, Y.; Long, Z.; Li, C. Int. J. Greenhouse Gas Control 2017, 63, 141–149.
- Kahyarian, A.; Singer, M.; Nesic, S. J. Nat. Gas Sci. Eng. 2016, 29, 530–549.
- Hu, K.; Zhuang, J.; Ding, J. Corros. Sci. 2017, 125, 68–76.
- Yin, Z.F.; Li, H.; Liu, L. Corros. Prot. 2013, 34 (9), 489–492.
- Singh, A.; Lin, Y.; Ansari, K.R. Appl. Surf. Sci. 2015, 359, 331–339.
- Lin, Y.; Singh, A.; Ebenso, E.E. J. Taiwan Inst. Chem. Eng. 2015, 46, 214–222.
- Muthukrishnan, P.; Jeyaprabha, B.; Prakash, P. Arab. J. Chem. 2013, 10, S2343–S2354.
- Hamdani, N.E.; Fdil, R.; Tourabi, M. Appl. Surf. Sci. 2015, 357, 1294–1305.
- Swaroop, B.S.; Victoria, S.N.; Manivannan, R.J. Taiwan Inst. Chem. Eng. 2016, 64, 269–278.
- Lebrini, M.; Robert, F.; Lecante, A. Corros. Sci. 2011, 53 (2), 687–695.
- Mourya, P.; Banerjee, S.; Singh, M.M. Corros. Sci. 2014, 85 (3), 352–363.
- Suedile, F.; Robert, F.; Roos, C.; Lebrini, M. Electrochim. Acta 2014, 133 (7), 631–638.
- Liao, L.L.; Mo, S.; Luo, H.Q. J. Colloid Interface Sci. 2018, 520, 41–49.
- Deyab, M.A. J. Ind. Eng. Chem. 2015, 22, 384–389.
- Jokar, M.; Farahani, T.S.; Ramezanzadeh, B. J. Taiwan Inst. Chem. Eng. 2016, 63, 436–452.
- Chevalier, M.; Robert, F.; Amusant, N. Electrochim. Acta 2014, 131 (1), 96–105.
- Ji, G.; Anjum, S.; Sundaram, S. Corros. Sci. 2015, 90 (1), 107–117.
- Shabani-Nooshabadi, M.; Ghandchi, M.S. J. Ind. Eng. Chem. 2015, 31, 231–237.
- Qiang, Y.; Zhang, S.; Tan, B. Corros. Sci. 2018, 6, 6–16.
- Heakal, E.T.; Deyab, M.A.; Osman, M.M. Desalination 2018, 425, 111–122.
- Alvarez, P.E.; Fiori-Bimbi, M.V.; Neske, A. J. Ind. Eng. Chem. 2017, 58, 92–99.
- Hassannejad, H.; Nouri, A. J. Mol. Liq. 2018, 254, 377–382.
- Ding, B.; Zhou, T.G.; Hong, Z. J. Pharm. Biomed. Anal. 2007, 45 (2), 219–226.
- Tourabi, M.; Nohair, K.; Traisnel, M. Corros. Sci. 2013, 75 (7), 123–133.
- Zheng, X.; Zhang, S.; Li, W. Corros. Sci. 2014, 80 (3), 383–392.
- Liao, L.L.; Mo, S.; Luo, H.Q. J. Colloid Interface Sci. 2017, 499, 110–119.
- Li, X.; Deng, S.; Fu, H. Corros. Sci. 2014, 78, 29–42.
- Ehsani, A.; Mahjani, M.G.; Hosseini, M. J. Colloid Interface Sci. 2017, 490, 444–451.
- Mobin, M.; Rizvi, M. Carbohydr. Polym. 2017, 160, 172–183.
- Baran, E.; Cakir, A.; Yazici, B. Arab. J. Chem. 2016. doi:10.1016/j.arabjc.2016.06.008.
- Chevalier, M.; Robert, F.; Amusant, N. Electrochim. Acta 2014, 131, 96–105.
- Singh, A.; Ansari, K.R.; Kumar, A. J. Alloys Compd. 2017, 712, 121–133.
- Rahal, C.; Masmoudi, M.; Abdelhedi, R. J. Electroanal. Chem. 2016, 769, 53–61.
- Prabakaran, M.; Kim, S.H.; Hemapriya, V. J. Ind. Eng. Chem. 2016, 37, 47–56.
- Rajesh, H.; Dwarika, P.; Akhil, S. J. Environ. Chem. Eng. 2018, 6, 2290–2301.
- Jamali, S.S.; Moulton, S.E.; Tallman, D.E. Corros. Sci. 2014, 86, 93–100.
- Simões, A.M.; Bastos, A.C.; Ferreira, M.G. Corros. Sci. 2007, 49 (2), 726–739.
- Jensen, M.B.; Peterson, M.J.; Jadhav, N. Prog. Org. Coat. 2014, 77 (12), 2116–2122.
- Ansari, K.R.; Quraishi, M.A.; Singh, A. RSC Adv. 2016, 6, 24130–24141.
- Singh, A.; Lin, Y.; Obot, I.B. Appl. Surf. Sci. 2015, 356, 341–347.
- Obot, I.B.; Onyeachu, I.B.; Kumar, A.M. Carbohyd. Polym. 2017, 178, 200–208.
- Zhang, D.; Tang, Y.; Qi, S. Corros. Sci. 2016, 102, 517–522.
- Fouda, A.S.; Ellithy, A.S. Corros. Sci. 2009, 51, 868–875.
- Ahamad, I.; Prasad, R.; Quraishi, M.A. Mater. Chem. Phys. 2010, 124, 1155–1165.