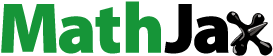
ABSTRACT
The objective of this study is to prepare a novel mouthwash that contains Amorphous Calcium Phosphate nanoparticles (ACP-NPs) in a chitosan aqueous solution in order to prevent and treat the existing enamel decalcification around the brackets and gingivitis. ACP-NPs have been synthesized via a co-precipitation technique from the solution of ammonium hydrogen phosphate and calcium chloride, as well as the addition of sodium hydroxide for the adjustment of pH ∼ 8. Fourier transform infrared (FTIR) spectroscopy, X-ray diffraction (XRD), field emission scanning electron microscope (FESEM), Energy dispersive X-ray spectroscopy (EDX), and the Barrett–Joyner–Halenda (BJH) desorption have been performed on the samples. The ACP-NPs seemed to contain an initial particle size of less than 40 nm and the Ca/P ratio of 1.14. We have applied chitosan since it can function as the stabilizer, antibacterial, and anti-inflammatory and thus, prevent plaque, bad breath, and decrease gingivitis. Agar dilution has been utilized to assess the antibacterial or effectiveness of the prepared mouthwash. This particular mouthwash can be considered smart since it has the ability to increase the ion release at a carcinogenic pH 4, which is the significant timing when these ions are mostly needed and required to inhibit the caries.
GRAPHICAL ABSTRACT
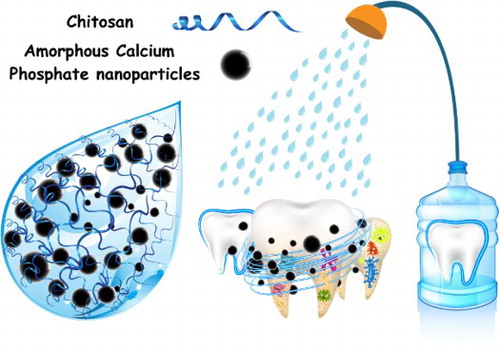
1. Introduction
Since the fix appliances in orthodontic are attached to the tooth surface, it is quite difficult to remove the food particles as well as cleaning the teeth and their related oral mucosa, while this matter is associated with the incidence and severity of White Spots Lesions (WSLs) (Citation1,Citation2). The existing white spots on teeth are known to be the resultants of decalcification (Citation3). Enamel decalcification and remineralization are the processes by which minerals, primarily calcium and phosphorus, can be induced on the labial surfaces of banded or banded teeth during the orthodontic treatments (Citation1,Citation4). On the other hand, the gums are usually more likely to swell during an orthodontic treatment and since this fact can cause controlling the problem a bit difficult (Citation5), searching and discovering new and natural alternatives for the available mouthwashes is quiet vital (Citation6). Mouthwashes have evolved over the years from containing any members from a whole range of various antimicrobial reagents, e.g. chlorhexidine (Citation7), towards the incorporation of more natural components such as natural polymers, bio-ceramics, and polysaccharides (Citation8). Recently, natural polymers including chitosan seem to have caught the interest of many due to being an alternative for the synthetic polymers in various technological procedures, since they have presented interesting features including being biocompatible, biodegradable, easily available, and particularly capable of functioning as a novel type of stabilizing materials (Citation9).
As a natural polysaccharides polymer, chitosan has received a great amount of attention regarding the preparation of different Mouthwashes (Citation10, Citation11). Very few compounds are classified as biodegradable and bioactive; therefore, chitosan and amorphous calcium phosphate, as members of hydroxyapatite family, are among the secure biomaterials that are employed in the field of dentistry and orthopedic tools (Citation12, Citation13). Nowadays, using of different nanomaterials such as Ag, CuO, and TiO2 are used in preparing new mouthwashes with various functionality (Citation14–16). Amorphous calcium phosphate (ACP) is known as one of the promising substances that can release calcium and phosphate for the prevention and treatment of decalcification (Citation17, Citation18). Recently, materials that contain ACP nanoparticles (ACP-NPs) have been especially attractive on the account of their excellent performance, considering how their properties are better than ACP in bulk form due to their specific area. This particular study is aimed to prepare and investigate the antibacterial properties of a water-soluble form of chitosan in a mouthwash that contains ACP-NPs rinse to function against de-mineralization and plaque regrowth; these materials have been considered for this objective due to their high level of calcium and phosphate ions. Moreover, this mouthwash will be designed to be “smart” in removing the cavity, WSLs, and relieve inflammation in patients that are undergoing orthodontic treatment.
2. Materials and method
2.1. Materials and reagents
Calcium Chloride (CaCl2, 99%, Merck, Germany), ammonium hydrogen phosphate [(NH4)2HPO4, Sigma, 99%, U.S.A.], and sodium hydroxide (NaOH, 98%, Sigma, U.S.A.) have been utilized as Ca-precursor, P-precursor, and pH adjusting reagent, respectively. All of the chemicals that have been used were of analytical grade, without any purification. All of the glassware involved in this research have been cleaned with fresh acidic solutions (HNO3/HCl; 3:1; v/v), washed with deionized water, and dried before being used. Deionized water has been included and employed in all of the experiments.
2.2. Synthesis of ACP-NPs
The ACP-NPs have been prepared through a modified co-precipitation method. Briefly, calcium chloride was dissolved in deionized water to form the Ca-precursor (0.036 M) and P-precursor solution (0.040 M), which were formulated by dissolving ammonium hydrogen phosphate in deionized water (). As the next step, the Ca-precursor and P-precursor solutions were combined thoroughly with Ca/P molar ratio of 1.50. Then, the pH value of the last solution was fixed and adjusted at 8.0 by appending the NaOH solution (0.1 M) while being continuously stirred at room temperature (25°C) until a white slurry formation was achieved. The precipitate was recovered from the solution by using a centrifuge (7000 rpm) for 10 min while being repeatedly washed to remove the existing soluble ions such as Cl−, NH4+, and Na+. Finally, the prepared calcium phosphate precipitates were washed with acetone for three times and dried at room temperature for a period of 24 h; they were later on stored under vacuum for characterization and further studies.
2.3. Preparation of ACP-based mouthwash
Chitosan from shrimp with a low molecular weight and a deacetylation degree of 90% has been obtained from Sigma-Aldrich Co., U.S.A. The chitosan was dissolved in acetic acid (0.10 M) solution with the concentration of 1.25% (w/w); then they were mixed with a defined amount of ACP-NPs (0.75% w/w) and gently stirred until it was thoroughly dissolved. The mixtures were reacted at 50°C for 24 h for the purposes of fabricating a stable mouthwash.
2.4. Characterization and instruments
The prepared ACP-NPs have been characterized through several laboratory types of equipments including FESEM (Field emission scanning electron microscopy; TESCAN, MIRA 3)/EDX (Energy Dispersive X-Ray), FTIR (Fourier transform infrared spectroscopy; Avatar 370, Thermo Nicolet, U.S.A.), and XRD (X-ray Powder Diffraction; D8 ADVANCE-BRUKER, Germany).
3. Results and discussions
illustrates the XRD pattern of the prepared ACP. The characterized peaks are indexed as ACP structure, which is in agreement with the JCPDS file # 01-080-3958. The broad XRD pattern suggests the existence of an amorphous calcium phosphate sample with a broad maximum at approximately 25° and 37°. The background level in the XRD pattern is often utilized by the ceramicists and polyamorists in order to ascertain the amorphous fraction of their materials. The substance that does not correlate with the “crystalline” phase regarding the diffract grams of Ca/P materials is normally known and contemplated to be “amorphous” (Citation19, Citation20).
The FTIR spectrum of the prepared ACP is shown in . Most of the derived peaks can be related to the ACP, including phosphate ()-derived bands at 470, 564, 603, 963, 1035, and 1094 cm−1, as well as the carbonate (
)-derived bands at 1567 and 2300–2500 cm−1, along with the broad peak that exists in between 3000 and 3800 cm−1 which is appointed to the O–H group of adsorbed water (Citation21). This result is quite similar to those of the ACP reported in Refs. (Citation22).
The estimated size and surface morphology of ACP-NPs has been illustrated by the particles size distribution () and FE-SEM images at different magnifications (). It had been observed that the particles are spherical in the co-precipitation ACP powder that is composed of primary nano-sized particles with diameters in a range of below 40 and about 57 nm by FE-SEM and particle size distribution. These NPs can be joined by the physical interactions such as van der Waals and/or Coulombic forces to turn into irregular agglomerates that are several tens of micrometers in size (Citation23). A great level of satisfying porosity has occurred with the agglomerates, while the interstitial porosity in between the primary NPs are the outcomes of the evaporating solvent (Citation24). The mentioned NPs and the high porosity seem to have subscribed to the great specific surface area that has been calculated and obtained for the ACP-NPs.
Figure 5. FESEM images of synthesized ACP-NPs at different magnifications; (a) 30, (b) 70, and (c) 100 kx, its EDX result (d), and elemental patterns (e).
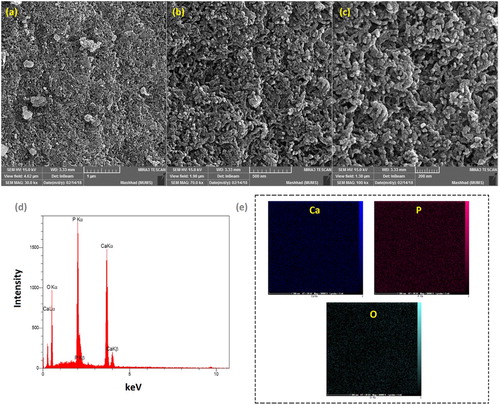
demonstrates the isotherms of nitrogen adsorption–desorption regarding the as-prepared ACP. In accordance to the International Union of Pure and Applied Chemistry (IUPAC), both of the existing isotherms seem to be a type IV isotherm loop that is known as one of the characteristics of porous structure (Citation25). The specific surface area of the Brunauer–Emmett–Teller (BET), regarding the as-prepared ACP, has been observed to be 138.31 m2 g−1. The Barrett–Joyner–Halenda (BJH) desorption cumulative pore volumes of ACP has been discovered to be 0.38cm3 g−1. The obtained high BET specific surface areas seem to be the resultants of the small sizes and porous structure of the as-prepared ACP nan-spheres (Citation26).
Representing dental plaque bacteria, three types of microorganisms have been applied and utilized in this project. Streptococcus mutans (ATCC 35668), Streptococcus sanguis (ATCC 10556), and Streptococcus oralis (ATCC 35037) have been obtained from BuAli Research Institute, Mashhad, Iran, which were assigned to be the subculture in 5% sheep's blood agar. Initially, taken from an overnight culture, 5–6 colonies were diluted in a brain heart infusion broth and then incubated in an aerobic environmental condition for the duration of 1–2 h at a temperature of 35°C for the purpose of achieving the concentration of 1.5 × 108 CFU/ml. Afterwards, in order to obtain the last concentration of 1.5 × 106 CFU/ml, the colonies were carefully diluted with saline solution. The lowest concentration of each antimicrobial agent that hinders the extension of microorganisms that are being examined seem to be recognized as the minimum inhibitory concentration (MIC), which can be diagnosed by observing the absence of turbidity that coordinates with negative control. For antibacterial experiments, 0.5 ml of the diluted microorganisms has been positioned in tubes that contained different concentrations of each nanoparticle; the tubes were then incubated overnight at a temperature of 35–37°C in a closed environment. The determination process of MIC was based on the turbidity that has been measured by the spectrophotometer (Eppendorf AG, Hamburg, Germany). After distinguishing the MIC, 50 ml of the corresponding bacterial suspension was spread in the sheep's blood agar and incubated at 37°C for a period of 24 h. The prepared mouthwash and two known types of mouthwashes, such as Oral-B and chlorhexidine, have been assessed by the agar dilution technique. The mouthwashes have been examined in ten different concentrations including 1/2, 1/4, 1/8, 1/16, 1/32, 1/64, 1/128, 1/256, 1/512, and 1/1024 while taking sterile agar as the diluent. and and shown an antibacterial effect scheme, antibacterial image and its values on the growth of three types of microorganisms, respectively.
Table 1. Effect of various mouthwashes on the growth of three types of microorganisms.
4. Conclusions
The present study has prepared ACP-NPs and chitosan mouthwash for the very first time and has performed investigations on their antibacterial effects. The existence of NPs that contain large surface areas have facilitated the mouthwash with antibacterial properties, along with high levels of calcium and phosphate ions release. The antibacterial qualities of the prepared mouthwash have matched with those of the previous ACP-NPs. Therefore, the ACP-NPs could be considered capable of impeding the caries restorations and stress bearing. The prepared mouthwash, in comparison to the ones in the market such as Oral-B and Chlorhexidine, contains more advantages such as being natural-based, non-toxic, eco-friendly, and low cost, along with the lack of hazardous side effects, e.g. stimulating the sense of taste or changing the color of teeth. Although most of the mouthwashes are not suitable for children due to their inability in utilizing the product correctly, yet this particular mouthwash has solved this problem by containing only natural materials.
Disclosure statement
No potential conflict of interest was reported by the authors.
Notes on contributors
Kimiya Pakravanan, MSc in solid state physics and she acts in green synthesis of different nanomaterials and investigation of their physical properties.
Mahmood Rezaee Roknabadi, professor of physics at Ferdowsi University of Mashhad with research interests in investigation of physical properties of various nanomaterials.
Fahimeh Farzanegan, associate professor of Orthodontics at Mashhad University of Medical Sciences with research interests in using of nanomaterials in dental applications.
Alireza Hashemzadeh, a postdoctoral researcher in the nanoscience center at Mashhad University of Medical Sciences, the specialist in design and synthesis of new inorganic nano-particles, porous and mesoporous materials for different purposes. He has 20 published works in this regard.
Majid Darroudi, assistant professor of Nanoscience at Mashhad University of Medical Sciences with research interests in green synthesis of different nanomaterials and investigation of their applications in medicine and pharmacy, published 110+ peer reviewed international papers with three-chapter books in both fields.
ORCID
Ali Reza Hashemzadeh http://orcid.org/0000-0001-7460-3312
Majid Darroudi http://orcid.org/0000-0002-2624-7242
References
- Mitchell, L. Decalcification During Orthodontic Treatment with Fixed Appliances – An Overview. Br. J. Orthod. 1992, 19 (8), 199–205.
- Kaygili, O. Synthesis and Characterization of Fe-Containing Biphasic Calcium Phosphate Ceramics. J. Aust. Ceram. Soc. 2018. doi:10.1007/s41779-018-0245-9.
- O'reilly, M.; Featherstone, J. Demineralization and Remineralization Around Orthodontic Appliances: An in Vivo Study. Am. J. Orthod. Dentofacial Orthop. 1987, 92, 33–40.
- Anuwongnukroh, N.; Dechkunakorn, S.; Kanpiputana, R. Oral Hygiene Behavior During Fixed Orthodontic Treatment. Dentistry. 2017, 7, 457–461.
- Ratcliff, P.A.; Johnson, P.W. The Relationship Between Oral Malodor, Gingivitis, and Periodontitis. A Review. J. Periodontol. 1999, 70 (5), 485–9.
- Khurshid, Z.; Naseem, M., Zafar, M.S., et al. Propolis: A Natural Biomaterial for Dental and Oral Healthcare. J. Dent. Res. Dent. Clin. Dent. Prospects. 2017, 11 (4), 265.
- Anderson, G.B.; Bowden, J., Morrison, E.C., et al. Clinical Effects of Chlorhexidine Mouthwashes on Patients Undergoing Orthodontic Treatment. Am. J. Orthod. Dentofacial Orthop. 1997, 111 (6), 606–12.
- Kokubo, T. Bioceramics and Their Clinical Applications, Elsevier: Sawston, UK, 2008.
- Rinaudo, M. Chitin and Chitosan: Properties and Applications. Prog. Polym. Sci. 2006, 31 (7), 603–32.
- Costa, E.; Silva, S., Madureira, A., et al. A Comprehensive Study into the Impact of a Chitosan Mouthwash upon Oral Microorganism's Biofilm Formation in Vitro. Carbohydr. Polym. 2014, 101, 1081–6.
- Costa, E.; Silva, S., Costa, M., et al. Chitosan Mouthwash: Toxicity and in Vivo Validation. Carbohydr. Polym. 2014, 111 (4), 385–92.
- Yates, M.; Ramos, M., Saez Rojo, E., et al. Biomaterials from Beer Manufacture Waste for Bone Growth Scaffolds AU – Martin-Luengo. M.A. Green Chemistry Letters and Reviews 2011, 4 (3), 229–33. doi:10.1080/17518253.2010.544331.
- Pepla, E.; Besharat, L.K., Palaia, G., et al. Nano-Hydroxyapatite and Its Applications in Preventive, Restorative and Regenerative Dentistry: A Review of Literature. Ann. Stomatol. (Roma) 2014, 5 (3), 108–14.
- Abadi, M.F.D.; Mehrabian, S., Asghari, B., et al. Silver Nanoparticles as Active Ingredient Used for Alcohol-Free Mouthwash. GMS. Hyg. Infect. Control. 2013, 8 (1). doi:10.3205/dgkh000205.
- Allaker, R.P. The Use of Nanoparticles to Control Oral Biofilm Formation. J. Dent. Res. 2010, 89 (11), 1175–86. doi:10.1177/0022034510377794.
- Eslami N, Ahrari F, Rajabi O, et al. The Staining Effect of Different Mouthwashes Containing Nanoparticles on Dental Enamel. J. Clin. Exp. Dent. 2015;7(4):e457-e461. doi:10.4317/jced.52199.
- Zhao, J.; Liu, Y., Sun, W.B., et al. First Detection, Characterization, and Application of Amorphous Calcium Phosphate in Dentistry. J. Dent. Sci. 2012, 7 (4), 316–23.
- Jabri, M.; Mejdoubi, E., El Gadi, M., et al. Synthesis and Optimization of a New Calcium Phosphate Ceramic Using a Design of Experiments. Res. Chem. Intermed. 2013, 39 (2), 659–69. doi:10.1007/s11164-012-0587-9.
- Prevéy, P.S. X-Ray Diffraction Characterization of Crystallinity and Phase Composition in Plasma-Sprayed Hydroxyapatite Coatings. J. Therm. Spray Technol. 2000, 9 (3), 369–76.
- Chen, F.; Yang, B., Qi, C., et al. An Amorphous Calcium Phosphate Nanocomposite for Storing and Sustained Release of IgY Protein with Antibacterial Activity. RSC Adv. 2015, 5 (122), 100682–8.
- Zamanian, A.; Yasaei, M., Ghaffari, M., et al. Calcium Hydroxide-Modified Zinc Polycarboxylate Dental Cements. Ceram. Int. 2013, 39 (8), 9525–32.
- Yu, T.; Ye, J., Gao, C., et al. Synthesis and Drug Delivery Property of Calcium Phosphate Cement with Special Crystal Morphology. J. Am. Ceram. Soc. 2010, 93 (5), 1241–4.
- Luo, Y.; Zhao, R.; Pendry, J.B. van der Waals Interactions at the Nanoscale: The Effects of Nonlocality. Proc. Natl. Acad. Sci. U. S. A. 2014, 111 (52), 18422–7.
- Layrolle, P.; Ito, A.; Tateishi, T. Sol-Gel Synthesis of Amorphous Calcium Phosphate and Sintering into Microporous Hydroxyapatite Bioceramics. J. Am. Ceram. Soc. 1998, 81 (6), 1421–8.
- Sing, K.S.; Williams, R.T. Physisorption Hysteresis Loops and the Characterization of Nanoporous Materials. Adsorpt. Sci. Technol. 2004, 22 (10), 773–82.
- Ding, G.-J.; Zhu, Y.-J., Qi, C., et al. Porous Microspheres of Amorphous Calcium Phosphate: Block Copolymer Templated Microwave-Assisted Hydrothermal Synthesis and Application in Drug Delivery. J. Colloid Interface Sci. 2015, 443, 72–9. http://doi.org/10.1016/j.jcis.2014.12.004