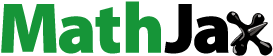
ABSTRACT
Continued use of chemical insecticides causes toxicity to non-target organisms, pest resistance and environmental pollution around the world. Nanocapsules of botanical insecticides are a suitable alternative for this target. The major constituents of Eucalyptus extract were determined by GC-MS: 1,8-cineole (70.94%) and 1,2-benzenedicarboxylic acid (6.08%). The Characterization of nanocapsule was investigated by dynamic light scattering, Zeta potential, scanning electron microscope, optical microscope and FTIR. The mean particle size distribution of nanocapsule is 380 nm and zeta potential of −26.36 ± 5.31 mV was obtained. It could be observed that nanocapsules have a spherical shape. To determine the insecticides of the nanocapsule with loads Eucalyptus extract was impregnated with concentration (0, 10, 15, 25, 35, 50 mg/ml) for 12, 24 and 48-h after exposure. The results indicated, that over time encapsulation exposure from 12 to 48 h, the highest time was comparatively more susceptible (LC50 = 14.93 mg/ml).
GRAPHICAL ABSTRACT
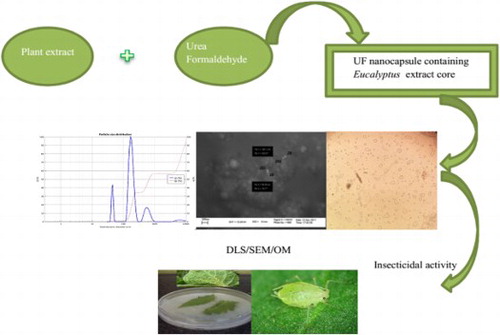
1. Introduction
The green peach aphid, Myzus persicae is the most economically important agricultural crop pests in Iran. Myzus persicae causes damage to its host by direct feeding, the transmission of plant viruses and the production of honeydew (Citation1–4). This widespread use of synthetic pesticides for agricultural has resulted in the presence of their residues in rivers, lakes, sea, soils, groundwater, and even drinking water, proves the high risk of these chemical insecticides on human health, toxicity to nontarget organisms and pest resistance (Citation5–9). The first case of chemical insecticide resistance in Myzus persicae was reported by Anthon in 1995 (Citation10). Many researchers have indicated that chemical insecticides cause qualitative and quantitative changes in the soil microbiota (Citation11, Citation12). The use of plant materials as botanical pesticides for the protection of farm crops against insect attack has a long history, botanical pesticides such as Thymus vulgaris (Citation13), Azadirachta indica (Citation14), Rosmarinus officinalis (Citation15), Artemisia absinthium (Citation16), Piper nigrum (Citation17), Artemisia vestita (Citation18) Crysanthemum cinerariaefolium (Citation19), tea leaves (Citation20). The botanical pesticides are an excellent alternative to synthetic pesticides as a means to decrease negative health effects, and non-target organisms and the environment (Citation21–23). Eucalyptus is known to contain bioactive compounds that indicated antifungal, antibacterial, antioxidative, fumigant and insecticides activities (Citation24–28).
Encapsulation of plant extracts and essential oils suitable method to improve stability, persistence, and protect them from adverse environmental conditions such as undesirable effects of light and moisture (Citation29). Encapsulation is a technology, which is specifically suitable to stabilize and control the release of bioactivity of natural extracts (Citation30). Encapsulation technology has been applied to develop controlled-release types of pesticides for crop protection (Citation31). Several encapsulation processes rely on the in situ polymerization technique and have been assayed briefly by different researchers (Citation32). Nano-pesticides consist of either very small particles of a pesticide active component (Citation33). During the past few years, a few researchers published studies on natural pesticides with nano-encapsulated covers such as Moretti and et al, investigated the effect of microcapsule and emulsions of R. officinalis L and Thymus herbal-Barona Loisel on Lymantria dispar L. that’'s one of the most serious pests cork oak forests. The result indicated that the encapsulation was a suitable method for protecting essential oils of very a different chemical ingredient (Citation34).
There is, however, no report on the insecticidal activity of hydroalcoholic Eucalyptus globulus extract and also nano-encapsulation of E. globulus extract as a green synthesis on the Myzus persicae. This paper first time report on the manufacture of urea formaldehyde (UF) nanocapsules loaded by E. globulus extract prepared by in situ polymerization in an oil-in-water emulsion. Our study aimed at developing insecticides using the nano-encapsulated Eucalyptus extract that is economical and safe. The nanocapsules Eucalyptus extract was used as an insecticide carrier for the first time against Myzus persicae. As the new technology in nano-encapsulated Eucalyptus extracts through the controlled release of active ingredients overcome the restrictions of plant extract usage in laboratory condition. In the present study, the insecticidal activity of the nano-encapsulation of Eucalyptus extract and pure extract on Myzus persicae was investigated.
2. Materials and methods
2.1. Materials
Urea (CH4N2O) and Polysorbate 20 (Tween, C58H114O26) were purchased from Merck Company. Formaldehyde (37 wt% in water) (CH2O) was obtained from Iranian Company (Dr. Mojallali). Sodium hydroxide solution 1 N and sulfuric acid 1 N were applied to adjust the pH of the solution. Palm oil was purchased from Margarian Company in Iran. The herb Eucalyptus globulus extract was used for insecticidal property for pest control.
2.2. Insect tested
Myzus persicae adults collected from greenhouses and farm from Anzali port in the north of Iran. The Myzus persicae were held with the host plant in mesh glass cages in 50 × 60 × 60 cm under greenhouse conditions. Myzus persicae stock was maintained at 25 ± 1°C and humidity 64 ± 10%.
2.3. Preparation of the plant extract
The E. globulus was collected from the Anzali port in the north of Iran in August 2017. The collected plants were washed with tap water and finally rinsed with distilled water to remove impurities. The plants were dried at room temperature, and then the sample was crushed by an electric mixture to powder. The sample was kept in a closed glass. The powder of Eucalyptus provided after grinding was used for extraction. 10 g of the powder Eucalyptus was refluxed in a Soxhlet apparatus in 100 ml of ethanol and water (30 vol% alcohol and 70 vol% distilled water). After 6 h, the hydroalcoholic extract was filtered through Whatmann No.42 filter paper, and the filtrate was dried to evaporate the organic solvent and water by freeze-dried apparatus (Lyophilization), then the sample was kept in the refrigerator at 4°C.
2.4. Preparation of Eucalyptus extract nanocapsule formulation
The preparation of the nanocapsule formulation of the Eucalyptus extract was performed by in situ polymerization technique using emulsion based on the oil-in-water method (Citation35). The plant extract was selected as nanocapsule nuclei, urea, and formaldehyde as nanocapsule wall pre-polymer. Four dosages of urea [Formulation1: 65, F2: 45, F3: 35, F4: 10 g], different values of 37% formaldehyde solutions [F1: 130, F2: 90, F3: 70 and F4: 20 ml] and distilled water were charged into a flask, equipped with the mechanical stirrer (Stirring initial rate: 300 rpm) and oil bath. The pH of the solution was adjusted to 8–9 by adding NaOH solution at room temperature. The solution was heated to the temperature of 60–65°C for 1 h. While the temperature was maintained at 60–65°C and high speed homogenizer [Stirring rate: F1:500, F2: 600, F3: 700, F4: 800 rpm], 20% (v/v) refined Palm oil and 10% (v/v) Polysorbate 20 was added to the solutions. The polymerization stirring rates is an important parameter in maintaining a uniform size distribution (Citation36). Next, 2 (g/ml) of Eucalyptus extract was added slowly and after 30 min, the pH of the solution was adjusted by sulfuric acid 1 N, until the poly urea-formaldehyde shell covers the particles of the extract. The creamy viscous liquid was produced after 3 h. At the end of the reaction, the liquid mixture was cooled by the addition of distilled water. After one hour cooling, nanocapsule product appeared as a cream solid. Moisture evaporates from samples by oven-drying. Place samples in an oven at approximately 50°C for 24 h, and then samples dried. Finely, F4 was chosen for insecticides activity studies as optimum formulation due to lowest UF value and high agitation rate (rpm). represents the composition of nanocapsules prepared at different processing parameters (Citation37). The mechanism of urea-formaldehyde formation was shown by Siva and Sathiyanarayanan (Citation38).
2.5. Characterization of nanocapsule
Nanocapsule morphology and size were determined SEM and OP. Dynamic light scattering technique has been applied to assign the mean size of nanocapsules (F4). The encapsulation yield was calculated from the equation (1). N is initial the weight (g) and C is weight of nanocapsule produced (g), (Citation39)(1)
(1)
Zeta potential: Zeta potential of synthesized nancapsules was analyzed to determine the charge present on the nanocapsules (Citation40). The Zeta potential test of F4 was performed at experimental parameters such as, temperature 24.47°C, pH 6.23, dielectric constant 78.60, electric field 8.51 V/cm and conductivity 0.041 mS/cm.
Table 1. The composition of nanocapsule prepared at different processing parameters.
2.6. The gas chromatography-mass spectrometry analysis (GC-MS)
GC-MS analysis of hydroalcoholic extracts of Eucalyptus performed using Perkin Elmer GC-MS (Model Perkin Elmer Clarus, 500, USA). Dimension: 30 Mts, ID: 0.25 mm, film thickness: 0.25 μm. The flow rate of the mobile phase was set at 1.0 ml/min (carrier gas: He). In the gas chromatography part, temperature programed (Oven temperature) was 60°C raised (3 min) to 280°C at 5°C/min and the injection volume was 1 μl of the sample (Citation20).
2.7. Fourier transform infrared Spectrophotometer
Fourier transform infrared (FTIR) is considered a powerful technique that can be applied to determine the chemical structure and type of chemical bonds present in compounds. FTIR measurements were done using Potassium bromide (KBr) technique. In this technique, a small amount of the sample powders [10 mg of Eucalyptus extract and10 mg of nanocapsule powder (F4)] is dispersed in KBr and then the samples were placed in FTIR spectroscopy (Alpha FTIR Spectrometer from Bruker optic). The FTIR spectrum of the plant extract and nanocapsule [F4: 10 g Urea + 20 ml formaldehyde solution + 200 ml distilled water + 20% (v/v) Palm oil + 10% (v/v) Polysorbate 20 and Stirring rate: 800 rpm] recorded at the 4000–500 cm−1 range.
2.8. The release profile of encapsulated plant extract
The release profile of encapsulation plant extract was evaluated at 25°C and 65% relative humidity. 2.00 g of the nanocapsule, placed in a plastic Petri dish covered with a nylon net. After (1, 3, 7, 11, 15, 25 days) the amount of the residual content of the encapsulated plant extract was weight. Each experiment was replicated three times. The release profile of encapsulation plant extract was done by a method (Citation41).
2.9. Insecticidal activity
To evaluate the insecticidal activity of Eucalyptus extract formulation, solutions were prepared at concentrations of 10, 15, 25, 35 and 50 mg/ml. A stock solution (w/v) was made with dissolving 10, 15, 25, 35 and 50 mg of nanocapsule (F4) in 100 ml distilled water, which was on a Shaker (Mod: Heidolph Unimax 1010) placed for homogenization (Agitation speed: 5 rpm, Time: 10 min).
The insecticidal activity of Eucalyptus extract formulation was tested by the fumigant method (Citation42). Insecticides activity of the nanocapsule involves Eucalyptus extract was evaluated, filter paper (Whatman No. 42) was impregnated with concentration (0, 10, 15, 25, 35, 50 mg/ml). 1 ml of the solutions was used. The impregnated filter papers were then attached to the plastic Petri dishes (8 cm diameter). Twenty-five of insects released into the Petri dishes. Petri dish was then either sealed with the pinholes Parafilm (Citation43). The number of dead insects was counted after initial exposure to the insecticides. Each concentration was replicated three times. Mortality was calculated 12, 24 and 48 h after exposure. Greenhouse conditions for all bioassays were 64 ± 10% RH and 25°C with a 16:8 (Light: Dark) photoperiod. The Probit analysis was employed in analyzing the dose-mortality response to estimate LC50 (Citation44). All statistical analyses were done using the statistical software SPSS version 13.0.
Nine concentrations of 0, 5, 10, 15, 20, 40, 60, 80 and 100 mg/ml were used for bioassays with Eucalyptus extract. The insecticidal activity of Eucalyptus extract was evaluated, filter paper (Whatman No. 42) was impregnated with concentrations 0, 5, 10, 15, 20, 40, 60, 80 and 100 mg/ml. 1 ml of the solutions was used. The impregnated filter papers were then attached to the plastic Petri dishes (8 cm diameter) was sealed with Parafilm to prevent any loss of plant extract that covered with perforated Parafilm for ventilation. Twenty-five of insects released into the Petri dishes. The number of dying insects was counted after 48 h of exposure. Laboratory conditions for all bioassays were 64 ± 10% RH and 25°C with a 16:8 (Light: Dark) photoperiod.
The investigation of toxicity durability Eucalyptus extract and nanocapsule with loads Eucalyptus extract on Myzus persicae was investigated at 25°C and 64% relative humidity. 50 mg/ml of the nanocapsule and 60 mg/ml of Eucalyptus extract, was prepared. Filter paper (Whatman No. 42) was impregnated with 1 ml of nanocapsule and Eucalyptus extract, the impregnated filter papers were then attached to the plastic Petri dish (8 cm diameter), twenty-five of Myzus persicae released into Petri dishes. In the same release period (2 and 30 days after preparation of nanocapsule (50 mg/ml) and pure Eucalyptus extract (60 mg/ml) in room temperature), the mortality of the test Myzus persicae after 48 h of exposure when treated with nanocapsule and pure Eucalyptus extract, nanocapsule with loads Eucalyptus extract compared to pure plant extract.
3. Results and Discussion
3.1. Detection of secondary metabolites in the plant extracts
The characterization of chemical compounds in the hydroalcoholic Eucalyptus globulus extract is shown in . The major chemical compounds in the hydroalcoholic extract were found () as 1,8-cineole (70.94%), 3-cyclohexene-1-ol (3.13%), beta. fenchyl (5.38%), 1,2-benzenedicarboxylic acid (6.08%), dodecane (1.50%). Eucalyptol (1, 3, 3-trimethyl-2-oxabicyclo [2.2.2] octane), also known as 1,8-cineole (CIN), is a natural constituent of the several natural sources (Citation45, Citation46). The main source is the Eucalyptus leaf oil, where CIN is present up to 90% (Citation47). The 1,8-cineole content of Eucalyptus globulus oil reported in the literature was 32.62%. This wide variation in chemical composition of Eucalyptus globulus could be ascribed to environmental conditions (Citation48). Several studies have been undertaken in the past to discover the potential of plants. 1,8-cineole, on the other hand, exhibits insecticidal activity 1,8-cineole, on the other hand, exhibits insecticides activity (Citation27, Citation49–51).
Table 2. The chemical constituents of the plant extract from Eucalyptus.
3.2. Fourier transform infrared (FTIR) Spectrophotometer
The FTIR spectrum of the pure Eucalyptus extract and nanocapsule (F4) recorded in the 4000–500 cm−1 range is indicated in (a, b). The (a) shows the FTIR spectra of the Eucalyptus extract powder. The peak at 3391.15 cm−1 is typically attributed to O–H bonds of phenols and alcohols (Citation52). The peak at 2929.42 cm−1 indicated the presence of –C–H (CH2; Vibration mode: Asymmetric and symmetric stretching). The peak at1607.38 cm−1 corresponds to N–H (NH2), C=C (Alkene; Vibration mode: Bending, stretching). The peak at 1516.50 cm−1 is indicated to N=O (NO2) and C=C (Aromatic) stretching. The bond at 1405.42 cm−1 is attributed to –C–H (CH3) bending. The peak at 1269.73–107.98 cm−1 can be assigned to the C–X, SO, C–N (NH2), C –O (OH, COOH) stretching. The peak at 863.29 cm−1 is indicated the presence of –C–H (Aromatic) bending. The peak at 680.02 and 529.40 cm−1 is assigned to the C–X (Halogen; Vibration of halogen and out of plane) (Citation53). (b) shows the FTIR spectra of the urea formaldehyde loaded with Palm oil and plant extract (F4). A strong absorption band at 3442.72 cm−1 indicated hydrogen bond N– H and OH. The band at 1643.54 cm−1arise from C=O stretching in –CONH2 and –NH–CO (Citation36). The peak near 2360 cm−1 corresponds to CO2. Absorption bands at 1112.78, 1196.04 and 1451.04 cm−1 correspond to –CN stretching, –CO stretching and C–H bending. The overlapping peaks at 2910.79 and 2964.49 cm−1 can be assigned to the CH/CH2/CH3 stretching (Citation54). The peak at 578 cm−1 is assigned to C–X (Halogen; Vibration of halogen and out of plane). The peak at 911.94 cm−1 is assigned to –C–H Alkenes (vibration mode: out of plane). The peak at 2084.35 cm−1 corresponds to N=C.
3.3. Characterization of nanocapsules
Morphology of the nanocapsules (F1-F4) was determined by scanning electron microscope (SEM) and optical microscope of nanocapsule (F4: optimum formulation). SEM images of nanocapsule (F1–F4), Dynamic light scattering (Nanocapsule: F4) and optical microscope (OM) image (F4) was illustrated in –. It could be observed that nanocapsules have a spherical shape. The particle size distribution of nanocapsule (F4) is 380 nm (). The mean zeta potential of synthesized nanocapsules was found to be −26.36 ± 5.31 mV which indicates that particles were relatively stable (Citation40). The zeta potential diagram of nanocapsule was indicated in . There are two spectrums of the nanometric particle (Citation1, Citation2) in treatment which particles under 100 nanometers can be found and make nanocapsule form. The morphology results of nanoparticles loaded with garlic essential oil and nanoparticle size indicated that the nanoparticles had a spherical shape and 240 nm in the average size distribution (Citation28). The nanoencapsulation yields are 95.52, 93.61, 94.59 and 94.16 for F1, F2, F3 and F4 respectively. The size distribution and the mean size of the nanocapsle (F4) is shown in .
Figure 2. SEM image of UF nanocapsule containing Eucalyptus extract core [F1: 65 g Urea + 130 ml formaldehyde solution + 200 ml distilled water + 20% (v/v) Palm oil + 10% (v/v) Polysorbate 20 and stirring rate: 500 rpm].
![Figure 2. SEM image of UF nanocapsule containing Eucalyptus extract core [F1: 65 g Urea + 130 ml formaldehyde solution + 200 ml distilled water + 20% (v/v) Palm oil + 10% (v/v) Polysorbate 20 and stirring rate: 500 rpm].](/cms/asset/824d4433-2474-4644-abad-55c511742025/tgcl_a_1643930_f0002_ob.jpg)
Figure 3. SEM image of UF nanocapsule containing Eucalyptus extract core [F2: 45 g Urea + 90 ml formaldehyde solution + 200 ml distilled water + 20% (v/v) Palm oil + 10% (v/v) Polysorbate 20 and stirring rate: 600 rpm].
![Figure 3. SEM image of UF nanocapsule containing Eucalyptus extract core [F2: 45 g Urea + 90 ml formaldehyde solution + 200 ml distilled water + 20% (v/v) Palm oil + 10% (v/v) Polysorbate 20 and stirring rate: 600 rpm].](/cms/asset/b4bb8d58-daf2-4235-b1fc-013b0762377a/tgcl_a_1643930_f0003_ob.jpg)
Figure 4. SEM image of UF nanocapsule containing Eucalyptus extract core [F3: 35 g Urea + 70 ml formaldehyde solution + 200 ml distilled water + 20% (v/v) Palm oil + 10% (v/v) Polysorbate 20 and stirring rate: 700 rpm].
![Figure 4. SEM image of UF nanocapsule containing Eucalyptus extract core [F3: 35 g Urea + 70 ml formaldehyde solution + 200 ml distilled water + 20% (v/v) Palm oil + 10% (v/v) Polysorbate 20 and stirring rate: 700 rpm].](/cms/asset/50e065fc-b01b-4db9-9f36-916f3dceb466/tgcl_a_1643930_f0004_ob.jpg)
Figure 5. SEM image of UF nanocapsule containing Eucalyptus extract core [F4: 10 g Urea + 20 ml formaldehyde solution + 200 ml distilled water + 20% (v/v) Palm oil + 10% (v/v) Polysorbate 20 and stirring rate: 800 rpm].
![Figure 5. SEM image of UF nanocapsule containing Eucalyptus extract core [F4: 10 g Urea + 20 ml formaldehyde solution + 200 ml distilled water + 20% (v/v) Palm oil + 10% (v/v) Polysorbate 20 and stirring rate: 800 rpm].](/cms/asset/d0c5cfdc-e1c6-448c-9017-772f99fc1bae/tgcl_a_1643930_f0005_ob.jpg)
3.4. Release profile of nanocapsule
The pattern of plant extract release from the nano-capsule is shown in . The amount of the residual content of the encapsulated plant extract after 1, 7, 14 and 21 days decreased from 100% to 96.5%. When exposed to room temperature and a moist environment, nanocapsule initially absorbed water. This absorption led to some modification in the wall materials (Citation34). After 7 days of exposure, no further decrease in the sample weight was observed. Therefore, it can be concluded that encapsulation of the extract plant can significantly prevent a fast weight loss. Over time, the gradual release of the extracts from the nanocapsule was observed.
3.5. Determination of Eucalyptus extract insecticidal activity and Eucalyptus extract and UF nanocapsule containing Eucalyptus extract core on insect
In the present investigation, the insecticidal activity of Eucalyptus extract nanocapsule was evaluated against Myzus persicae. From the , it can be seen that the highest concentration (50 mg/ml) of the Eucalyptus extract encapsulation yielded 100% mortality of Myzus persicae at 48 h exposure. The mortality of Myzus persicae was increased with an increased solution concentration and exposure time of Eucalyptus extract nanocapsule. These results agree with researchers (Citation29, Citation41). The total mortality of all insects was achieved with the highest concentration and over time, presumably due to the slow and the persistent release of the active components from the nanocapsule (Citation29). Probit analysis showed in , that over time encapsulation exposure from 12 to 48 h, the highest time was comparatively more susceptible (LC50 = 14.93 mg/ml). Tukey’'s HSD test showed the significant difference between the treatment (Sig <0.05) in (). Results show that concentration and time were all significant for the mortality of test pests after two days of exposure when treated with encapsulated Eucalyptus. The insecticidal activity of Eucalyptus extract at different concentrations is shown in . From the Figure, insecticidal activity appears at 5 ppm and was increased with an increased in Eucalyptus extract to 60 mg/ml, which provides a perfect insecticidal activity for 48-h exposure. 60, 80 and 100 mg/ml plant extract gave 100% mortality and were significantly (F = 1630.350, df = 8, Sig = 0.000) at 48 h. LC50 of the testing Eucalyptus extract was determined to be 16.95 mg/ml for 48-h exposure (). Insecticidal activities of nano-encapsulated Eucalyptus globulus extract with UF were not reported against insects till data as evidenced by researchers. The repellent and insecticidal effects of E. globulus essential oil with different nanostructures (nanoemulsion and nanocapsules) were tested on Haemotobia irritans flies and Musca domestica. After 24-h, a significant decrease in horn flies’ population was observed using free and nano-encapsulated forms investigated. Eucalyptus globulus essential oil demonstrated insecticidal and repellent effects on M. domestica and H. irritans flies (Citation55). In another study, investigated the encapsulation of Eucalyptus oil nanoemulsion with Chitosan and to examine the larvicidal activity on Culex tritaeniorhynchus. The encapsulated beads of Eucalyptus oil indicated 100% mortality of Culex tritaeniorhynchus rate within 12-h (Citation56). Insecticidal activities of E. globulus extract were not studied against pests till data as evidenced by researchers, while the insecticide activities of E. globulus oil were investigated on insects. Abdel and Morsy, reported that essential oils from E. globulus showed toxicity against M. domestica (Citation57). In a similar study, effects of E. globulus essential oils against Lasioderma serricorne F. and Rhyzopertha dominica F. were investigated by using the fumigation method. The mortality of Lasioderma serricorne F. and R. dominica was increased as the exposure time and the dose of the E. globulus oil increased. Obtained results suggest that toxicity properties of E. globulus oil can be proposed as alternatives to chemical pesticides for controlling R. dominica and L. serricorne (Citation58). The summary of the literature about the natural insecticides with major compounds, including 1,8-cineole in was presented (Citation59–63). In the present study, the mortality of Myzus persicae was increased as the exposure time and the dose of the nanoencapsulated Eucalyptus globulus extract increased. The toxicity durability of Eucalyptus extract () 100% mortality and for nanocapsule, 100% mortality for 2 days. The toxicity durability of Eucalyptus extracts 8% mortality and for nanocapsule, 72% mortality for 30 days, presumably due to the slow and the persistent release of the active components from the nanocapsule (Citation29). The result was indicated, that the encapsulation is a suitable method for protecting plant extract of very a different chemical ingredient. Nowadays by using new technologies such as nano-encapsulated formulation can overcome the limitations of plant extract and essential oil (Citation34). In this study, the plant extract of Eucalyptus was encapsulated by in-situ polymerization of oil/water. Fumigation assay was conducted to investigate the toxicity of the E. globulus against adults of Myzus persicae. The effective fumigation assay of Eucalyptus globulus extract was basically due to the presence of the volatile constituents such as monoterpenes (1,8-Cineole) (Citation64, Citation65). The mechanism of action of these compounds exerts their neurotoxic effects on insects involving multifarious mechanisms such as inhibition of nerve conduction enzyme acetylcholinesterase and octopamine synapses (Citation66).
Figure 9. The mean mortality percentage of Myzus persicae at different concentrations of Eucalyptus extract at 48-h.
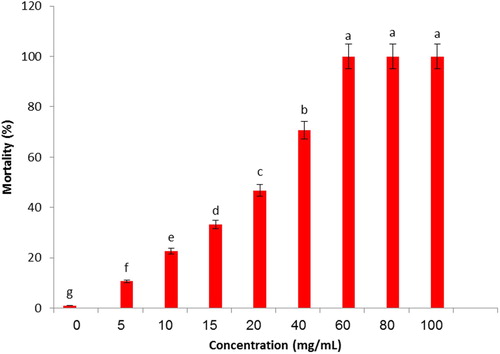
Figure 10. Comparison of toxicity durability of Eucalyptus extract and UF nanocapsule containing Eucalyptus extract core (F4) on Myzus persicae.
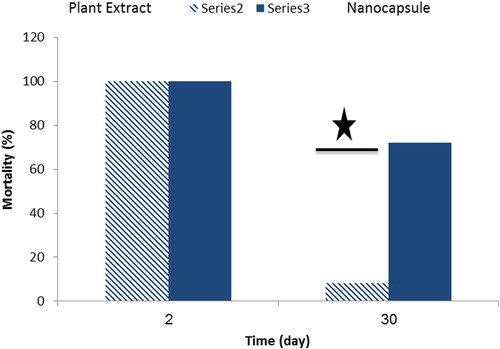
Table 3. Insecticidal activity of Eucalyptus extract formulation on Myzus persicae at 12, 24 and 48 h.
Table 4. Probit analysis for insecticidal activity of Eucalyptus extract nanocapsule on Myzus persicae at 12, 24 and 48 h.
Table 5. Probit analysis for insecticidal activity of Eucalyptus extract on Myzus persicae.
Table 6. The summary of the literature about the natural insecticides with major compounds, including 1,8-cineole.
4. Conclusion
globulus The insecticidal activity of producing nano-encapsulated plant extract of Eucalyptus was investigated on Myzus persicae. As the new technology in nano-encapsulated Eucalyptus extracts through the controlled release of active ingredients overcome the restrictions of plant extract usage in laboratory condition. Mean particle size distribution of nanocapsules (F4) investigated by Dynamic light scattering technique is 380 nm and zeta-potential of −26.36 mV. The observation of nanocapsules by SEM and optical microscope (OM), it could be concluded that nanocapsules have a spherical shape. The main reason for their insecticidal properties of the Eucalyptus globulus extract might be attributed to the highest percentage of 1,8-cineole (70.94%) which can be used as a botanical insecticidal agent for integrated Myzus persicae. Results show that concentration and time were all significant for the mortality of test pests after two days of exposure when treated with encapsulated Eucalyptus. The toxicity durability of Eucalyptus extracts was reported 8% mortality and for nanocapsule, 72% mortality for 30 days. The amount of the residual content of the encapsulated plant extract after 1, 7, 14 and 21 days decreased from 100% to 96.5%. The conclusions of this study indicate that nano-encapsulated Eucalyptus extract affects the pest Myzus persicae, and can be effective alternative synthetic pesticides.
Conflict of interest
The authors disclose that there is no any conflict of interest in connection with submitted manuscripts.
Disclosure statement
No potential conflict of interest was reported by the authors.
Notes on contributors
Zohreh Khoshraftar received M.Sc. degree in applied chemistry from Islamic Azad University- Ardabil branch, Ardabil, Iran, in 2014. She is a Ph.D. Student in Applied Chemistry in Islamic Azad University- Science and Research branch, Tehran, Iran, since 2015. Her main areas of interests are Nano technology, Green chemistry and water treatment.
Ali Akbar Safekordi is a Full Professor of chemical Engineering. He received his Ph.D. from University of Manchester. He published more than 150 papers in international journals. His main areas interests are Food Processing, Mass Transfer Phenomena, Equipment Design and Green Chemistry.
Ali shamel is an Associate Professor of physical chemistry. He published more than 20 papers in international journals. He supervisor of 150 Master Degree and 2 Ph.D. Degree. His main areas interests are nano synthesis, Adsorption, Solvent effect, Kinetics and Green chemistry.
Mohammad Zaefizadeh is an assistant professor of agriculture Faculty of Biology, Ardabil branch, Islamic Azad University, Ardabil, Iran. He published more than 10 papers in international journals.
References
- Khoshraftar, Z.; Safekordi, A.A.; Shamel, A.; Zaefizadeh, M. Evaluation of Insecticidal Activity of Nanoformulation of Melia Azedarach (leaf) Extract as a Safe Environmental Insecticide. Int. J. Environ. Sci. Technol. 2019, 1–12. doi:10.1007/s13762-019-02448-7.
- Wang, S.Y.; Feng, Y.; Liang, N.N.; Tang, R.; Liu, Y.H.; Liu, T.X. Starving Aphelinus Asychis Negatively Affects Host Feeding and Parasitism on Myzus Persicae. J. Asia-Pac. Entomol. 2018. doi:10.1016/j.aspen.2018.03.017.
- Castle, S.J.; Berger, P.H. Rates of Growth and Increase of Myzus Persicae on Virus-Infected Potatoes According to Type of Virus-Vector Relationship. Entomol. Exp. Appl. 1993, 69, 51–60. doi:10.1111/j.1570-7458.1993.tb01727.x.
- Tang, Q.L.; Ma, K.S.; Hou, Y.M.; Gao, X.W. Monitoring Insecticide Resistance and Diagnostics of Resistance Mechanisms in the Green Peach Aphid, Myzus Persicae (Sulzer)(Hemiptera: Aphididae) in China. Pestic. Biochem. Physiol. 2017, 143, 39–47. doi:10.1016/j.pestbp.2017.09.013.
- Zolgharnein, J.; Shahmoradi, A.; Ghasemi, J. Pesticides Removal Using Conventional and low-Cost Adsorbents: a Review. Clean–Soil, Air, Water 2011, 39, 1105–1119. doi:10.1002/clen.201000306.
- Rodrigues, E.T.; Alpendurada, M.F.; Ramos, F.; Pardal, MÂ. Environmental and Human Health Risk Indicators for Agricultural Pesticides in Estuaries. Ecotoxicol. Environ. Saf. 2018, 150, 224–231. doi:10.1016/j.ecoenv.2017.12.047.
- Ye, M.; Beach, J.; Martin, J.W.; Senthilselvan, A. Pesticide Exposures and Respiratory Health in General Populations. J. Environ. Sci. 2017, 51, 361–370. doi:10.1016/j.jes.2016.11.012.
- Jallow, M.F.; Awadh, D.G.; Albaho, M.S.; Devi, V.Y.; Thomas, B.M. Pesticide Risk Behaviors and Factors Influencing Pesticide use among Farmers in Kuwait. Sci. Total Environ. 2017, 574, 490–498. doi:10.1016/j.scitotenv.2016.09.085.
- Baqar, M.; Sadef, Y.; Ahmad, S.R.; Mahmood, A.; Li, J.; Zhang, G. Organochlorine Pesticides Across the Tributaries of River Ravi, Pakistan: Human Health Risk Assessment Through Dermal Exposure, Ecological Risks, Source Fingerprints and Spatio-Temporal Distribution. Sci. Total Environ. 2018, 618, 291–305. doi:10.1016/j.scitotenv.2017.10.234.
- Anthon, E.W. Evidence for Green Peach Aphid Resistance to Organo-Phosphorous Insecticides1. J. Econ. Entomol. 1955, 48, 56–57. doi:10.1093/jee/48.1.56.
- Hartmann, M.; Frey, B.; Mayer, J.; Mäder, P.; Widmer, F. Distinct Soil Microbial Diversity Under Long-Term Organic and Conventional Farming. ISME J. 2015, 9, 1177–1194.
- Komorowicz, I.; Gramowska, H.; Barałkiewicz, D. Estimation of the Lake Water Pollution by Determination of 18 Elements Using ICP-MS Method and Their Statistical Analysis. J. Environ. Sci. Health, Part A: Environ. Sci. Eng. 2010, 45, 348–354. doi:10.1080/10934520903467873.
- Enan, E.E. Molecular and Pharmacological Analysis of an Octopamine Receptor From American Cockroach and Fruit fly in Response to Plant Essential Oils. Arch. Insect Biochem. Physiol. 2005, 59, 161–171. doi:10.1002/arch.20076.
- Nathan, S.S.; Choi, M.Y.; Seo, H.Y.; Paik, C.H.; Kalaivani, K.; Kim, J.D. Effect of Azadirachtin on Acetylcholinesterase (AChE) Activity and Histology of the Brown Planthopper Nilaparvata Lugens (Stål). Ecotoxicol. Environ. Saf. 2008, 70, 244–250. doi:10.1016/j.ecoenv.2007.07.005.
- Dayan, F.E.; Cantrell, C.L.; Duke, S.O. Natural Products in Crop Protection. Bioorg. Med. Chem. 2009, 17, 4022–4034. doi:10.1016/j.bmc.2009.01.046.
- Höld, K.M.; Sirisoma, N.S.; Ikeda, T.; Narahashi, T.; Casida, J.E. α-Thujone (the Active Component of Absinthe): γ-Aminobutyric Acid Type A Receptor Modulation and Metabolic Detoxification. Proc. Natl. Acad. Sci. 2000, 97, 3826–3831. doi:10.1073/pnas.070042397.
- Singh, R.N.; Saratchandra, B. The Development of Botanical Products with Special Reference to Seri-Ecosystem. Caspian J. Environ. Sci. 2005, 3, 1–8.
- Chu, S.S.; Liu, Q.R.; Liu, Z.L. Insecticidal Activity and Chemical Composition of the Essential oil of Artemisia Vestita From China Against Sitophilus Zeamais. Biochem. Syst. Ecol. 2010, 38, 489–492. doi:10.1016/j.bse.2010.04.011.
- Casida, J.E.; Quistad, G.B. Golden age of Insecticide Research: Past, Present, or Future? Annu. Rev. Entomol. 1998, 43, 1–16. doi:10.1146/annurev.ento.43.1.1.
- Khoshraftar, Z.; Shamel, A.; Safekordi, A.A.; Zaefizadeh, M. Chemical Composition of an Insecticidal Hydroalcoholic Extract From tea Leaves Against Green Peach Aphid. Int. J. Environ. Sci. Technol. 2018, 1–8. doi:10.1007/s13762-018-2177-x.
- Koul, O.; Walia, S.; Dhaliwal, G.S. Essential Oils as Green Pesticides: Potential and Constraints. Biopestic Int. 2008, 4, 63–84.
- Devine, G.J.; Furlong, M.J. Insecticide use: Contexts and Ecological Consequences. Agric. Human. Values. 2007, 24, 281–306.
- Villaverde, J.J.; Sevilla-Morán, B.; Sandín-España, P.; López-Goti, C.; Alonso-Prados, J.L. Biopesticides in the Framework of the European Pesticide Regulation (EC) No. 1107/2009. Pest Manage. Sci. 2014, 70, 2–5. doi:10.1002/ps.3663.
- Batish, D.R.; Singh, H.P.; Kohli, R.K.; Kaur, S. Eucalyptus Essential oil as a Natural Pesticide. Forest Ecol. Manag. 2008, 256, 2166–2174. doi:10.1016/j.foreco.2008.08.008.
- Martins, C.; Natal-da-Luz, T.; Sousa, J.P.; Gonçalves, M.J.; Salgueiro, L.; Canhoto, C. Effects of Essential Oils from Eucalyptus Globulus Leaves on Soil Organisms Involved in Leaf Degradation. PLos. One 2013, 84, e61233. doi:10.1371/journal.pone.0061233.
- Sebei, K.; Sakouhi, F.; Herchi, W.; Khouja, M.L.; Boukhchina, S. Chemical Composition and Antibacterial Activities of Seven Eucalyptus Species Essential Oils Leaves. Biol. Res. 2015, 48, 7. doi:10.1186/0717-6287-48-7.
- Maciel, M.V.; Morais, S.M.; Bevilaqua, C.M.L.; Silva, R.A.; Barros, R.S.; Sousa, R.N.; Sousa, L.C.; Brito, E.S.; Souza-Neto, M.A. Chemical Composition of Eucalyptus spp. Essential Oils and Their Insecticidal Effects on Lutzomyia Longipalpis. Vet. Parastiol. 2010, 167, 1–7. doi:10.1016/j.vetpar.2009.09.053.
- Rossi, Y.E.; Palacios, S.M. Insecticidal Toxicity of Eucalyptus Cinerea Essential oil and 1, 8-Cineole Against Musca Domestica and Possible Uses According to the Metabolic Response of Flies. Ind. Crops Prod. 2015, 63, 133–137. doi:10.1016/j.indcrop.2014.10.019.
- Yang, F.L.; Li, X.G.; Zhu, F.; Lei, C.L. Structural Characterization of Nanoparticles Loaded with Garlic Essential Oil and Their Insecticidal Activity AgainstTribolium Castaneum(Herbst) (Coleoptera: Tenebrionidae). J. Agric. Food Chem. 2009, 57, 10156–10162. doi:10.1021/jf9023118.
- Nikmaram, N.; Roohinejad, S.; Hashemi, S.; Koubaa, M.; Barba, F.J.; Abbaspourrad, A.; Greiner, R. Emulsion-based Systems for Fabrication of Electrospun Nanofibers: Food, Pharmaceutical and Biomedical Applications. RSC Adv. 2017, 7, 28951–28964. doi:10.1039/C7RA00179G.
- Kang, M.A.; Seo, M.J.; Hwang, I.C.; Jang, C.; Park, H.J.; Yu, Y.M.; Youn, Y.N. Insecticidal Activity and Feeding Behavior of the Green Peach Aphid, Myzus Persicae, after Treatment with Nano Types of Pyrifluquinazon. J. Asia-Pac. Entomol. 2012, 15, 533–541. doi:10.1016/j.aspen.2012.05.015.
- Salaun, F. Microencapsulation by Interfacial Polymerization. In Encapsulation Nanotechnologies; Mittal, V., Ed.; Scrivener Publishing/Wiley: Salem, MA, 2013, pp 137–173.
- Kookana, R.S.; Boxall, A.B.; Reeves, P.T.; Ashauer, R.; Beulke, S.; Chaudhry, Q.; Cornelis, G.; Fernandes, T.F.; Gan, J.; Kah, M.; Lynch, I. Nanopesticides: Guiding Principles for Regulatory Evaluation of Environmental Risks. J. Agric. Food. Chem. 2014, 62, 4227–4240. doi:10.1021/jf500232f.
- Moretti, M.D.; Sanna-Passino, G.; Demontis, S.; Bazzoni, E. Essential oil Formulations Useful as a new Tool for Insect Pest Control. AAPS PharmSciTech. 2002, 3, 64–74. doi:10.1208/pt030213.
- Rochmadi, A.P.; Hasokowati, W. Mechanism of Microencapsulation with Urea-Formaldehyde Polymer. Am. J. App. Sci. 2010, 7, 739–745.
- Nguon, O.; Lagugné-Labarthet, F.; Brandys, F.A.; Li, J.; Gillies, E.R. Microencapsulation Byin SituPolymerization of Amino Resins. Polym. Rev. 2018, 58, 326–375. doi:10.1080/15583724.2017.1364765.
- Yuan, L.; Liang, G.; Xie, J.; Li, L.; Guo, J. Preparation and Characterization of Poly (Urea-Formaldehyde) Microcapsules Filled with Epoxy Resins. Polymer 2006, 47, 5338–5349. doi:10.1016/j.polymer.2006.05.051.
- Siva, T.; Sathiyanarayanan, S. Self Healing Coatings Containing Dual Active Agent Loaded Urea Formaldehyde (UF) Microcapsules. Prog. Org. Coat. 2015, 82, 57–67. doi:10.1016/j.porgcoat.2015.01.010.
- Khoee, S.; Yaghoobian, M. An Investigation Into the Role of Surfactants in Controlling Particle Size of Polymeric Nanocapsules Containing Penicillin-G in Double Emulsion. Eur. J. MedChem. 2009, 44, 2392–2399. doi:10.1016/j.ejmech.2008.09.045.
- Patel, V.R.; Agrawal, Y.K. Nanosuspension: An Approach to Enhance Solubility of Drugs. J. Adv. Pharm. Technol. Res. 2011, 2, 81–87. doi:10.4103/2231-4040.82950.
- Passino, G.S.; Bazzoni, E.; Moretti, M.D.L. Microencapsulated Essential Oils Active Against Indianmeal Moth. Bol.San.Veg.Plagas. 2004, 30, 125–132.
- Negahban, M.; Moharramipour, S.; Sefidkon, F. Insecticidal Activity and Chemical Composition of Artemisia Sieben Besser Essential oil From Karaj, Iran. J. Asia-Pac. Entomol. 2006, 9, 61–66. doi:10.1016/S1226-8615(08)60276-9.
- Kim, S.I.; Roh, J.Y.; Kim, D.H.; Lee, H.S.; Ahn, Y.J. Insecticidal Activities of Aromatic Plant Extracts and Essential Oils Against Sitophilus Oryzae and Callosobruchus Chinensis. J. Stored Prod. Res. 2003, 39, 293–303. doi:10.1016/S0022-474X(02)00017-6.
- Finney, D.J. Probit Analysis, 3rd ed.; Cambridge University Press: London, 1971. 333 pp.
- Isikber, A.A.; Alma, M.H.; Kanat, M.; and Karci, A. Fumigant Toxicity of Essential Oils FromLaurus Nobilis AndRosmarinus Officinalis Against all Life Stages OfTribolium Confusum. Phytoparasitica. 2006, 34, 167–177. doi: 10.1007/BF02981317
- Tapondjou, A.L.; Adler, C.; Fontem, D.A.; Bouda, H.; Reichmuth, C.H. Bioactivities of Cymol and Essential Oils of Cupressus Sempervirens and Eucalyptus Saligna Against Sitophilus Zeamais Motschulsky and Tribolium Confusum du Val. J. Stored Prod. Res. 2005, 41, 91–102. doi:10.1016/j.jspr.2004.01.004.
- Aparicio, S.; Alcalde, R.; Dávila, M.J.; García, B.; Leal, J.M. Properties of 1, 8-Cineole: a Thermophysical and Theoretical Study. J. Phys. Chem. 2007, 111, 3167–3177. doi:10.1021/jp067405b.
- Kumar, P.; Mishra, S.; Malik, A.; Satya, S. Compositional Analysis and Insecticidal Activity of Eucalyptus Globulus (Family: Myrtaceae) Essential oil Against Housefly (Musca Domestica). Acta Trop. 2012, 122, 212–218. doi:10.1016/j.actatropica.2012.01.015.
- Tripathi, A.K.; Prajapati, V.; Aggarwal, K.K.; Kumar, S. Toxicity, Feeding Deterrence, and Effect of Activity of 1, 8-Cineole From Artemisia Annua on Progeny Production of Tribolium Castanaeum (Coleoptera: Tenebrionidae). J. Econ. Entomol. 2001, 94, 979–983. doi:10.1603/0022-0493-94.4.979.
- Arabi, F.; Moharramipour, S.; Sefidkon, F. Chemical Composition and Insecticidal Activity of Essential oil From Perovskia Abrotanoides (Lamiaceae) Against Sitophilus Oryzae (Coleoptera: Curculionidae) and Tribolium Castaneum (Coleoptera: Tenebrionidae). J. Trop. Insect Sci. 2008, 28, 144–150. doi:10.1017/S1742758408079861.
- Bekele, J.; Hassanali, A. Blend Effects in the Toxicity of the Essential oil Constituents of Ocimum Kilimandscharicum and Ocimum Kenyense (Labiateae) on two Post-Harvest Insect Pests. Phytochemistry 2001, 57, 385–391. doi:10.1016/S0031-9422(01)00067-X.
- Khoshraftar, Z.; Shamel, A. Adsorption of Malachite Green Dye from Aqueous Solutions Using Roots of Azolla Filiculoides. J. Phys. Theor. Chem. 2017, 13, 237–252.
- Easmin, S.; Sarker, M.Z.I.; Ghafoor, K.; Ferdosh, S.; Jaffri, J.; Ali, M.E.; Mirhosseini, H.; Al-Juhaimi, F.Y.; Perumal, V.; Khatib, A. Rapid Investigation of α-Glucosidase Inhibitory Activity of Phaleria Macrocarpa Extracts Using FTIR-ATR Based Fingerprinting. J. Food Drug Anal. 2017, 25, 306–315. doi:10.1016/j.jfda.2016.09.007.
- Ullah, H.; Azizli, K.; Man, Z.B.; Ismail, M.B. Synthesis and Characterization of Urea-Formaldehyde Microcapsules Containing Functionalized Polydimethylsiloxanes. Procedia. Eng. 2016, 148, 168–75. 4th International Conference on Process Engineering and Advanced Materials.
- Galli, G.M.; Roza, L.F.; Santos, R.C.; Quatrin, P.M.; Ourique, A.F.; Klein, B.; Wagner, R.; Baldissera, M.D.; Volpato, A.; Campigotto, G.; Glombowsky, P. Low Dose of Nanocapsules Containing Eucalyptus Oil Has Beneficial Repellent Effect Against Horn Fly (Diptera: Muscidae). J. Econ. Entomol. 2018, 111, 2983–2987. doi:10.1093/jee/toy267.
- Sugumar, S.; Chandrakar, D.; Kanimozhi, G.; Mukherjee, A.; Chandrasekaran, N. Eucalyptus Oil Emulsion Loaded Biodegradable Polymeric Beads as a Bio-Larvicide Agent Against Culex Tritaeniorhynchus. Adv. Sci., Eng. Med. 2015, 7, 134–139. doi:10.1166/asem.2015.1660.
- Abdel, A.H.; Morsy, T.A. The Insecticidal Activity of Eucalyptus Globulus oil on the Development of Musca Domestica Third Stage Larvae. J. Egypt. Soc. Parasitol. 2005, 35, 631–336.
- Ebadollahi, A.; Safaralizadeh, M.H.; Pourmirza, A.A. Fumigant Toxicity of Essential Oils of Eucalyptus Globulus Labill and Lavandula Stoechas L., Grown in Iran, Against the two Coleopteran Insect Pests; Lasioderma Serricorne F. and Rhyzopertha Dominca F. Egypt. J. Biolo. Pest Contr. 2010, 20, 1–5.
- Bett, P.K.; Deng, A.L.; Ogendo, J.O.; Kariuki, S.T.; Kamatenesi-Mugisha, M.; Mihale, J.M.; Torto, B. Chemical Composition of Cupressus Lusitanica and Eucalyptus Saligna Leaf Essential Oils and Bioactivity Against Major Insect Pests of Stored Food Grains. Ind. Crops Prod. 2016, 82, 51–62. doi:10.1016/j.indcrop.2015.12.009.
- Hamza, A.F.; El-Orabi, M.N.; Gharieb, O.H.; El-Saeady, A.H.A.; Hussein, A.R.E. Response of Sitophilus Granarius L. to Fumigant Toxicity of Some Plant Volatile Oils. J. Radiat. Res. Appl. Sci. 2016, 9, 8–14. doi:10.1016/j.jrras.2015.05.005.
- Lee, B.H.; Lee, S.E.; Annis, P.C.; Pratt, S.J.; Park, B.S.; Tumaalii, F. Fumigant Toxicity of Essential Oils and Monoterpenes Against the red Flour Beetle, Tribolium Castaneum Herbst. J. Asia-Pac. Entomol. 2002, 5, 237–240. doi:10.1016/S1226-8615(08)60158-2.
- Jemâa, J.M.B.; Haouel, S.; Bouaziz, M.; Khouja, M.L. Seasonal Variations in Chemical Composition and Fumigant Activity of Five Eucalyptus Essential Oils Against Three Moth Pests of Stored Dates in Tunisia. J. Stored Prod. Res. 2012, 48, 61–67. doi:10.1016/j.jspr.2011.10.001.
- Pant, M.; Dubey, S.; Patanjali, P.K.; Naik, S.N.; Sharma, S. Insecticidal Activity of Eucalyptus oil Nanoemulsion with Karanja and Jatropha Aqueous Filtrates. Int. Biodeterior. Biodegrad 2014, 91, 119–127. doi:10.1016/j.ibiod.2013.11.019.
- Jemâa, J.M.B.; Haouel, S.; Khouja, M.L. Efficacy of Eucalyptus Essential Oils Fumigant Control Against Ectomyelois Ceratoniae (Lepidoptera: Pyralidae) Under Various Space Occupation Conditions. J. Stored Prod. Res. 2013, 53, 67–71. doi:10.1016/j.jspr.2013.02.007.
- Reyes, E.I.; Farias, E.S.; Silva, E.M.; Filomeno, C.A.; Plata, M.A.; Picanço, M.C.; Barbosa, L.C. Eucalyptus Resinifera Essential Oils Have Fumigant and Repellent Action Against Hypothenemus Hampei. Crop Prot. 2019, 116, 49–55. doi:10.1016/j.cropro.2018.09.018.
- Rattan, R.S. Mechanism of Action of Insecticidal Secondary Metabolites of Plant Origin. Crop Prot. 2010, 29, 913–920. doi:10.1016/j.cropro.2010.05.008.