ABSTRACT
The biogenic synthesis of silver nanoparticles (AgNPs) using Aloe fleurentiniorum extract and antibacterial activity of the synthesized AgNPs were investigated. Examinations using ultraviolet–visible spectrophotometry, fourier transform infrared spectroscopy, energy-dispersive X-ray spectroscopy, and transmission electron microscopy confirmed the efficacy of AgNP biosynthesis using A. fleurentiniorum extract. Purely prepared AgNPs were spherical and moderately sized. The antibacterial activity of AgNPs was evaluated through the agar well diffusion assay using Staphylococcus aureus, methicillin-resistant S. aureus, Escherichia coli, and Salmonella typhimurium. The biosynthesized AgNPs consistently displayed strong antibacterial activity.
Introduction
Nanoparticles are particles with a diameter of less than 100 nanometers. Nanoparticles (NPs), including silver nanoparticles (AgNPs), have diverse potential applications in the biotechnology, biomedical, and agriculture sectors as components of packaging and coating materials, food storage materials, optical and electronic materials, cleaning agents, besides other applications ( Citation1–3).
NPs can be synthesized using chemical and physical methods. Biological methods of NP synthesis, which use plant extracts, are considered eco-friendly, relatively safe, and economical compared to conventional chemical and physical methods. The plant extract-based biological approach can be readily scaled up to address a broad range of manufacturing needs. This approach does not require the use of specialized temperature, power, toxic chemicals, or high pressure conditions (Citation 4). The many types of nanomaterials include the aforementioned AgNPs, as well as NPs incorporating gold, magnesium (Citation 5), alginate (Citation 6), zinc, copper, and titanium (Citation 7). AgNPs are particularly advantageous, since they display strong antimicrobial activity against bacteria, fungi, protozoa, viruses, and other microorganisms.
AgNPs have been synthesized by many plant extracts, including of plants the leaves of Aloe vera, Portulaca oleracea, and Cynodon dactylon (Citation 8) Artemisia marschalliana (Citation 9), Rosmarinus officinalis (Citation 10), and Terminalia chebula (Citation 11); root extracts of Diospyros paniculata (Citation 12); and cowpea seeds (Vigna sp. L) (Citation 13). Microorganisms have also been used for biosynthesis of AgNPs, including Aspergillus terreus (Citation 14), as have monosaccharides (Citation 15).
In seeking another source of raw material for the biosynthesis of AgNPs, we presently studied Aloe fleurentiniorum (also known as Yemeni Brown Aloe). This plant grows naturally in Yemen, Oman, and in the southern region of the Kingdom of Saudi Arabia. We evaluated the use of A. fleurentiniorum extract as a reducing agent for the biosynthesis of AgNPs. The synthesized AgNPs were characterized using a variety of techniques and their antibacterial activity was evaluated.
Materials and methods
Materials
A. fleurentiniorum plants were collected from southern Yemen. The plants were verified as A. fleurentiniorum by expert personnel at the Herbarium of the Department of Botany and Microbiology, King Saud University, Kingdom of Saudi Arabia. Freshly harvested plants were washed thoroughly several times with distilled water and air-dried in the dark. Silver nitrate, Muller-Hinton agar, and Nutrient broth were obtained from Sigma–Aldrich (USA). Gram-positive bacteria included Staphylococcus aureus ATCC 29213 and methicillin-resistant S. aureus (MRSA). Gram-negative bacteria included Escherichia coli and Salmonella typhimurium. The bacteria were obtained from the Laboratory of Microbiology, Science College, King Saud University.
Biogenic synthesis of AgNPs using A. fleurentiniorum extract
A previously described method (Citation 16) was used, with some minor modifications, for the biogenic synthesis of AgNPs using A. fleurentiniorum extract. Dried powder of the plants (10 g) was soaked in 100 mL deionized water and boiled for 10 min with continuous stirring. The extract was then filtered through Whatman No 1. To synthesize AgNPs, 10 mL of the filtered extract was added to 100 mL of AgNO3 (0.01 mM). The mixture was stirred for 10 min and centrifuged at 13000 rpm for 20 min. The pellet of AgNPs were dehydrated in an oven at 40°C for 2 h. The resulting preparation was then characterized.
Characterization of AgNPs
Ultraviolet–visible spectroscopy
UV–Vis spectroscopy was done using an Ultrospec 2100 Pro UV/visible spectrophotometer (Biochrom, UK) at wavelengths ranging from 200 to 800 nm.
Fourier transform infrared (FTIR) spectroscopy
FTIR spectroscopy analysis was carried out for both AgNPs and extract of A. fleurentiniorum to monitor the presence of functional groups responsible for synthesis of NPs. FTIR spectra were scanned from 400 to 4000 cm−1 at a resolution of 4 cm−1.
Energy-dispersive X-ray spectroscopy (EDS)
EDS analysis was performed using an Altima IV device (Regaku, Japan) as previously described (Citation 17).
Transmission electron microscopy (TEM)
TEM was used to determine the size and morphology of the AgNPs. A JEM-1011 microscope (JEOL, Japan) was used.
Antibacterial activities
The antibacterial activities of the biogenically synthesized AgNPs were studied using two representative gram-positive bacteria (S. aureus and MRSA) and two representative gram-negative bacteria (E. coli and S. typhimurium) through the agar well diffusion method (Citation 18). Twenty milliliters of Mueller Hinton Agar medium was poured into petri dishes and allowed to solidify. The agar was inoculated to create a lawn of growth of each bacterium; next, 6 mm diameter wells were created using a sterile agar borer. One hundred microliter aliquots of the AgNP suspension were added to the wells. The dishes were incubated at 37°C for 24 h. The diameters of resulting zones of growth inhibition around the wells were measured.
Statistical analysis
The results are presented as the mean ± standard deviation (SD). All procedures were carried out in triplicate. The statistical analysis was done using SPSS software (SPSS Inc., USA) with one-way analysis. A P-value < 0.05 was considered statistically significant.
Results and discussion
To our knowledge, this is the first study of the biogenic synthesis of AgNPs using an extract of the Aloe species A. fleurentiniorum. Synthesis of NPs, including AgNPs, using plant extracts has advantages, including safety, simplicity, absence of organic solvents, and low-cost (Citation 3, Citation 19, Citation 20). In the present study, an immediate color change of the plant extract–AgNO3 mixture to dark brown, which reflected the reduction of Ag+ to Ag0, was evident. Moreover, the formation of the AgNPs was confirmed through UV–visible spectrophotometry. The absorption spectrum was recorded at 400 nm (), and the pattern of absorption was similar to that shown in previous reports (Citation 8, Citation 21, Citation 22).
Figure 1. UV–vis absorption spectrum of the AgNPs phytosynthesized using A. fleurentiniorum extract.
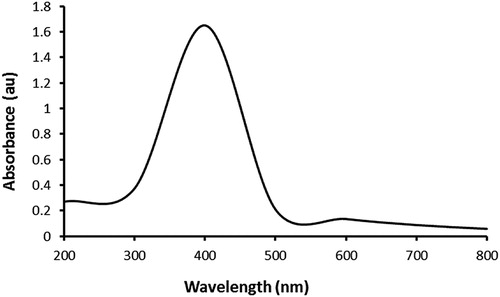
FTIR examination was performed to confirm the existence of biomolecules in the A. fleurentiniorum extract, which were responsible for the biosynthesis of AgNPs. The FTIR spectra of the A. fleurentiniorum extract and the biogenically synthesized AgNPs are displayed in (A,B), respectively. A number of bands in the FTIR spectrum of A. fleurentiniorum extract were absent in that of the biogenically synthesized AgNP solution. The extreme band in the FTIR profile was 3411.2 cm−1 and was associated with O-H stretching due to phenols (Citation 23). The extreme second band in the FTIR profile, at 1603.7 cm−1 , was associated with the C = O bond present in ketones, carboxylic acids, quinones, and esters (Citation 24, Citation 25). Other bonds were not evident in biogenically synthesized AgNPs.
Figure 2. FTIR spectra of Aloe fleurentiniorum extract (A) and AgNPs produced by A. fleurentiniorum extract (B).
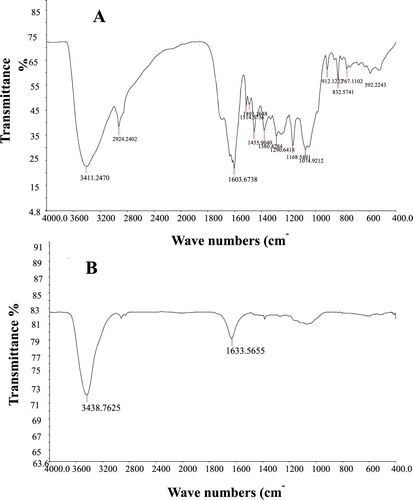
EDS analysis () was carried out to evaluate the elemental composition of AgNPs produced by the A. fleurentiniorum extract. Strong signals were observed in the Ag zone. Strong signals at approximately 3 keV are typical of the absorption peak of AgNPs (Citation 26, Citation 27, Citation 9). EDS analysis also revealed the existence of uncontaminated Ag atoms (72.39%). The minimal presence of other signals was attributed to chlorine and oxygen, which most likely belonged to biomolecules from the plant extract (Citation 9).
A typical TEM image () was used to measure the shape and size of the biosynthesized AgNPs. Spherical and slightly agglomerated AgNPs were observed. The AgNP diameter varied from 8 to 27 nm. These measurements were consistent with previous studies (Citation 17, Citation 9, Citation 22).
The antibacterial activity of AgNPs synthesized using A. fleurentiniorum extract was examined using two representative gram-positive bacteria (S. aureus ATCC 29213 and MRSA) and two representative gram-negative bacteria (E. coli and S. typhimurium). Preparations containing 1.5 and 4 mg of AgNPs were used. summarizes the diameters of the inhibition zones. The results indicated a strong antibacterial activity of the biosynthesized AgNPs. The results echo previous descriptions of the antibacterial activity of other plant extracts (Citation28–31, Citation 21).
Table 1. Antibacterial activity of AgNPs synthesized by A. fleurentiniorum extract.
Conclusions
In this study, the biogenic synthesis of AgNPs using A. fleurentiniorum extract was investigated. This is the first description of the use of this extract for the biosynthesis of AgNPs. The biosynthesized AgNPs were obtained as a pure preparation. The success of the biosynthesis was confirmed using a variety of analytical examinations. The NPs were spherical and had a moderate size. Their antibacterial activity was confirmed against representative gram-positive and gram-negative bacteria that can pose health risks. These data reinforce the efficacy of using plant extracts for the biosynthesis of AgNPs, and indicate the specific value of A. fleurentiniorum extract for AgNP biosynthesis.
Acknowledgements
This project was supported by Researchers Supporting Project number (RSP-2019/5) King Saud University, Riyadh, Saudi Arabia.
Disclosure statement
No potential conflict of interest was reported by the authors.
Notes on contributors
Saleh H. Salmen is assistant professor in Botany and Microbiology Department, King Saud University, Kingdom of Saudi Arabia.
Sulaiman Ali Alharbi is professor in Botany and Microbiology Department, King Saud University, Kingdom of Saudi Arabia.
Additional information
Funding
References
- Rittner, M.N.; Abraham, T.; Miner, J. Metals Mater. Soc. 1998, 50, 37–38. doi: 10.1007/s11837-998-0065-4
- Zhang, X.F.; Liu, Z.G.; Shen, W.; Gurunathan, S. Int. J. Mol. Sci. 2016, 17, 1534. doi: 10.3390/ijms17091534
- Ahluwalia, V.; Elumalai, S.; Kumar, V.; Kumar, S.; Sangwan, R.S. Microb. Pathog. 2018, 114, 402–408. doi: 10.1016/j.micpath.2017.11.052
- Antariksh, S.; Tripathi, R.M.; Fahmina, Z.; Priti, S. Mater. Lett. 2012, 67, 91–94. doi: 10.1016/j.matlet.2011.09.038
- Gu, H.; Ho, P.L.; Tong, E.; Wang, L.; Xu, B. Nano Lett. 2003, 3, 1261–1263. doi: 10.1021/nl034396z
- Ahmad, Z.; Pandey, R.; Sharma, S.; Khuller, G.K. Ind. J. Chest Dis. Allied Sci. 2005, 48, 171–176.
- Retchkiman, S.; Canizal, P.S.; Becerra-Herrera, G.; Zorrilla, R.; Liu, C.; Ascencio, H.B. Opt. Mater. 2006, 29, 95–99. doi: 10.1016/j.optmat.2006.03.014
- Tarad, A.A.; Al harbi, S.A.; Salmen, S.H.; Wainwright, M. Biotechnol. Biotechnol. Equip. 2017, 31, 411–417. doi: 10.1080/13102818.2016.1267594
- Salehi, S.; Shandiz, A.S.; Ghanbar, F.; Darvish, M.R.; Ardestani, M.S.; Mirzaie, A.; Jafari, A. Int. J. Nanomed. 2016, 11, 1835–1846.
- Ghaedi, M.; Yousefinejad, M.; Safarpoor, M.; Zare Khafri, H.; Purkait, M.K. J. Industr. Eng. Chem. 2015, 31, 167–172. doi: 10.1016/j.jiec.2015.06.020
- Chandra, S.E.; Krishna Rao, K.V.; Madhusudana Rao, K. Mater. Let. 2016, 174, 129–133. doi: 10.1016/j.matlet.2016.03.106
- Hanumanta Rao, N.; Lakshmidevi, N.; Pammi, S.V.; Kollu, P.; Ganapaty, S.; Lakshmi, P. Mater. Sci. Eng. C 2016, 62, 553–557. doi: 10.1016/j.msec.2016.01.072
- Mohammadi, S.; Pourseyedi, S.; Amini, A. J. Environ. Chem. Eng. 2016, 4, 2023–2032. doi: 10.1016/j.jece.2016.03.026
- Sayed, S.M.; Bahkali, A.H.; Marwa, M.B.; Hirad, A.H.; Elgorban, A.E.; El-Metwally, M.A. Int. J. Pharmacol. 2015, 11, 858–863. doi: 10.3923/ijp.2015.858.863
- Colin, P.; Zheng, D.; Muhi, M.Z.; Scott Pease, M.; Mottaleb, A.; Rafiq Islam, M. Nanotechnol. 2014, doi: 10.1155/2014/480284
- Silva, B.O.; Seabra, A.B. Biointerface Re. Appl. Chem. 2016, 6, 1280–1287.
- Elgorban, A.M.; Al-Rahmah, A.N.; Sayed, S.R.; Hirad, A.; Mostafa, A.F.; Bahkali, A.H. Biotechnol. Biotechnol. Equip. 2016, 30, 299–304. doi: 10.1080/13102818.2015.1133255
- Valodkar, M.; Nagar, P.S.; Jadeja, R.N.; Thounaojam, M.C.; Devkar, R.V.; Thakore, S. Colloids Surf. A Physicochem. Eng. Asp. 2011, 384, 337–344. doi: 10.1016/j.colsurfa.2011.04.015
- Ramdani, D.; Chaudhry, A.S.; Seal, C.J. J. Agric. Food Chem. 2013, 61, 496–4967. doi: 10.1021/jf4002439
- Duran, N.; Seabra, A.B. Curr. Nanosci. 2018, 14, 82–94.
- Premkumara, J.; Sudhakara, T.; Dhakala, A.; Babu Shresthaa, J.B.; Krishnakumara, S.; Balashanmugam, P. Biocataly. Agric. Biotechnol. 2018, 15, 311–316. doi: 10.1016/j.bcab.2018.06.005
- Rolim, W.R.; Pelegrino, M.T.; Lima, B.A.; Ferraz, L.S.; Costaa, F.N.; Bernardes, J.S.; Rodigues, T.; Brocchi, M.; Seabra, A.B. Appl. Surf. Sci. 2019, 463, 66–74. doi: 10.1016/j.apsusc.2018.08.203
- Preeti, D.; Mausumi, M. J. Nanopart Res. 2013, 15, 1366. doi:10.1007/s11051-012-1366-7.
- Sun, Q.; Cai, X.; Li, J.; Zheng, M.; Chen, Z.; Yu, C.P. Colloids Surf. A Physicochem. Eng. Aspects 2014, 444, 226–231. doi: 10.1016/j.colsurfa.2013.12.065
- Carballo, T.; Gil, M.V.; Gómez, X.; González-Andrés, F.; Morán, A. Biodegradation 2008, 19, 815–830. doi: 10.1007/s10532-008-9184-4
- Nadagouda, M.N.; Speth, T.F.; Varma, R.S. Acc. Chem. Res. 2011, 44, 469–478. doi: 10.1021/ar1001457
- Khan, M.; Khan, S.T.; Khan, M.; Adil, S.F.; Musarrat, J.; Al-Khedhairy, A.A.; Al-Warthan, A.; Siddiqui, M.R.; Alkhathlan, H.Z. Int. J. Nanomed. 2014, 9, 3551–3565.
- Puiso, J.; EJonkuviene, D.; Macioniene, I.; Salomskiene, J.; Jasutiene, I.; Kondrotas, R. Colloids Surf. B Biointerfaces 2014, 121, 214–221. doi: 10.1016/j.colsurfb.2014.05.001
- Ismail, E.; Khenfouch, M.; Dhlamini, M.; Dube, S.; Maaza, M. J. Alloys Comp. 2017, 695, 3632–3638. doi: 10.1016/j.jallcom.2016.11.390
- Khalil, T.A.; Ovais, M.; Ullah, I.; Ali, M.; Shinwari, Z.K.; Maaza, M. Arabian J. Chem. 2017, doi: 10.1016/j.arabjc.2017.07
- Matinise, N.; Kaviyarasu, K.; Mongwaketsi, N.; Khamlich, S.; Maaza, M. Appl. Surf. Sci. 2018, 446, 66–73. doi: 10.1016/j.apsusc.2018.02.187